The Cellular Microenvironment and Metastases
Erinn B. Rankin, Janine Erler and Amato J. Giaccia
• Metastases are responsible for more than 90% of all cancer-related deaths.
• Gene mutations, the tumor microenvironment, and host cells drive the metastatic spread of tumor cells.
• Metastasis can be subdivided into four steps: invasion, intravasation, survival in circulation, and extravasation.
• Colonization of metastatic tumor cells requires the ability to proliferate in a foreign tissue and stimulate angiogenesis.
• The formation of a premetastatic niche is essential for the growth of extravasating metastatic tumor cells.
• Organ specificity of tumor metastases is determined both by blood flow and tissue-specific factors.
• Primary tumors possess stem cells that can recapitulate the tumor from a single cell, and a subset of these cancer stem cells may inherently possess altered gene expression changes with increased metastatic potential.
• Antimetastatic therapy will likely require the targeted inhibition of many pathways that control proliferation, invasion, and angiogenesis.
Mechanisms of Metastasis
Hematogenous Metastasis
Most tumors metastasize through the bloodstream. Metastatic dissemination through the vasculature involves a series of distinct cellular adaptations, including invasion and migration from the primary tumor; intravasation into the vasculature; arrest and extravasation from the vasculature into the distant organ; and proliferation and survival into the new tissue microenvironment (Fig. 3-1). The acquisition of these biological traits by tumor cells involves the coordination of both cellular intrinsic and extrinsic signals.
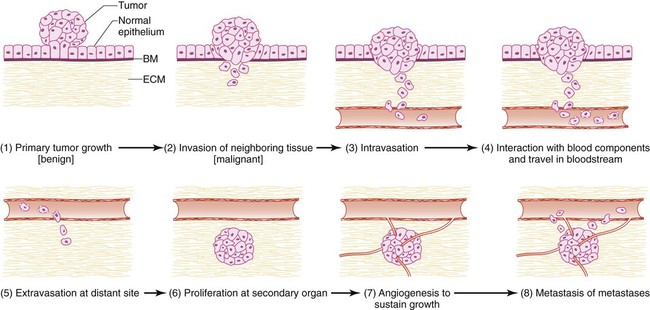
Invasion
Changes in Cell Adhesion
The first step of metastasis is invasion. Cells must undergo changes in their cell-cell and cell-matrix adhesion interactions to dissociate themselves from the tumor.1 Acquisition of an invasive phenotype requires changes in expression of genes that control cell-cell adhesion, as well as proteolytic degradation of the extracellular matrix (ECM).2 Cell-cell adhesions are mediated primarily by E-cadherin proteins expressed at junctions between cells.3 Cadherins bind cells through protein-protein interactions at their extracellular domains, whereas their intracellular domains signal to catenins and the actin cytoskeleton. Changes in E-cadherin expression allow cells to detach from their neighbors and begin their migratory route toward the circulatory or lymphatic system to seek out new terrain. Reduced expression of E-cadherin is often observed in aggressive cancers through epigenetic silencing, proteosomal degradation, proteolytic cleavage, or mutation. In fact, inactivating mutations of E-cadherin have been shown to predispose patients to gastric cancer, implicating E-cadherin as a tumor suppressor gene.1
Loss of E-cadherin is highly associated with epithelial to mesenchymal transition (EMT), a program that is essential for numerous developmental processes. 3 The acquisition of the invasive phenotype has many similarities to EMT, including loss of cell-cell adhesion mediated by E-cadherin repression and an increase in cell mobility. During EMT, a switch occurs from E-cadherin (an epithelial cell marker) to N-cadherin expression (a mesenchymal cell marker), which promotes cell-matrix adhesion instead of cell-cell adhesion.3
Several signal transduction pathways, such as the Ras-MAPK and WNT pathways, have been shown to regulate EMT (Fig. 3-2). In particular, the Ras-MAPK pathway activates two related transcription factors known as Snail and Slug.4,5 Both of these proteins act as transcriptional repressors of E-cadherin, and their expression induces EMT in cancer cells.6 Studies have indicated that Slug is an independent prognostic parameter for poor survival in patients with colorectal carcinoma. 7 Twist, another basic helix-loop-helix transcription factor that is necessary for proper embryonic development, has also been shown to induce EMT through the repression of E-cadherin. 8 Both Twist and Snail expression levels are elevated in patients with breast cancer and are associated with poor prognosis.8,9 Dysregulation of Wnt signaling is common in many types of human cancers and regulates EMT in part through Snail activation, an important early step in metastasis.10
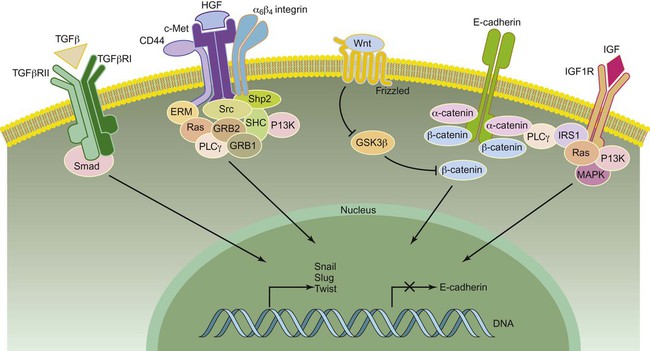
Cell Motility
Cancer cells are able to take advantage of many mechanisms to migrate and invade, including both individual and collective cell-migration strategies.2 Most cancer cells of epithelial origin undergo EMT and acquire invasive migration capacity to enter the circulatory or lymphatic system. Invasive migration is a dynamic and complex process involving changes in cell-matrix adhesion and the cytoskeleton. Changes in cell-matrix adhesion are necessary for the leading edge of the cell to grab onto the matrix surrounding it and pull itself forward in a movement similar to an inchworm. This invasive migration can be viewed as cycles of adhesion and detachment, allowing the cell to bind, then detach after pulling forward. Cell-matrix adhesions are in large part regulated by integrin proteins. Integrins are heterodimers of one of 18 alpha and 8 beta transmembrane proteins that bind to specific components of the ECM. 11 They can transmit signals into or out of the cell and are important mediators of malignant transformation.
Integrins are stimulated when they come into contact with specific ECM substrates, or through growth factor–stimulated signaling where they interact with receptor tyrosine kinases.14–14 For example, hepatocyte growth factor (also known as scatter factor) influences invasion by signaling through its receptor c-met.15 Integrin stimulation also promotes formation of focal adhesion contacts, focal adhesion kinase (FAK) activation through phosphorylation, and formation of FAK-Src complexes.14 It is noteworthy that Src mutations that have been implicated in tumor cell motility are often observed in human cancers such as adenocarcinoma of the colon.16 Intracellular signaling mediated by FAK activates Rac, RhoC, cdc42, and other guanosine triphosphatases that mediate cellular changes required for invasion.12 These changes include actin-myosin contraction that propels the cell forward and recruitment of matrix metalloproteinases (MMPs) to focal adhesion sites where they degrade the ECM, allowing the cell to glide forward when focal adhesion complexes are disassembled after contraction.2 In addition, the remodeled matrix tracks left behind the cell have been shown to facilitate movement of subsequent cells, similar to the generation of ski tracks by the first cross-country skier that allow subsequent skiers to move more easily.17
Disruption of the Basement Membrane
The basement membrane provides a physical barrier between epithelial cells and the stroma. The basement membrane is composed of numerous glycoproteins and proteoglycans that provide ligands for integrins, permitting control of cell orientation and outside to inside signaling. Epithelial and stromal cells produce a mixture of these components that form a dense meshwork underlying the epithelial cells. Tumor cells overcome this barrier by altering the expression of their cell surface receptors such that they can now adhere to basement membrane components.2 For example, tumor cells will increase expression of integrins that can bind laminin and collagen IV.18 Increased CD44 expression permits cell binding to hyaluronan, a basement membrane proteoglycan, and is observed in several types of human cancer, including metastatic colon carcinomas.19 In addition, cancer cells modify the components of the basement membrane to ease penetration. For example, reduced laminin expression is observed in poorly differentiated human colon carcinomas.20
ECM protease activity is tightly regulated by proteins that inhibit their functions.21 Tumor cells proteolytically disrupt the basement membrane by altering the balance between ECM proteases and their inhibitory proteins. For example, elevated MMP expression and activity increases degradation of the basement membrane. MMP-1 (also known as collagenase or gelatinase) degrades collagen IV and is increased in highly metastatic cancer cells.22 MMP degradation of the ECM not only facilitates cell movement but also generates a large number of bioactive cleaved peptides and releases growth factors and chemokines trapped within the ECM mesh.21 For example, MMP activity releases active forms of proteoglycans including heparin, hyaluronate, and chondroitin sulfate.23,24
Intravasation
The entry of tumor cells into the circulation (intravasation) and the exit of tumor cells from the circulation (extravasation) to host tissue represent critical steps in the metastatic process. One clear difference between intravasation and extravasation has to do with the composition of the blood vessels. Tumor blood vessels are malformed and irregular, often possessing breaks in their thin lining that permit the easy access of tumor cells into the circulation. In contrast, the vasculature of normal tissue where tumor cells extravasate do not have these same features. This very observation suggests that the processes of intravasation and extravasation are distinct and probably require different gene functions. Our knowledge of the genetic determinants involved in intravasation is limited. Gradients of chemoattractant proteins such as chemokines have been proposed to guide cells toward the circulatory system.17 In addition, tumor cells move along collagen fibers produced by invading cells, a process facilitated by host macrophages.17
Survival in the Circulatory System
Before these tumor cells can extravasate into a target tissue, they must first survive the environment of the circulatory system. Tumor cells in the circulatory system are subjected to immune attack, circulatory forces, and anoikis (apoptosis induced by loss of adhesion).25 Circulating tumor cells bind to platelets to protect themselves from these dangers, thus increasing their chance of survival.28–28 Tumor cells also bind to coagulation factors including thrombin, fibrinogen, tissue factor, and fibrin, creating emboli.29 These tumor cell emboli are more resilient to both circulatory forces and immune attack and have been shown to have greater metastatic potential than single tumor cells.27 In the circulation, aggregates of tumor cells are termed homotypic clumps because they are homogeneous in their cellular composition, whereas those associated with platelets are termed heterotypic clumps and may possess greater metastatic potential for the reasons described previously.26,28
Arrest and Extravasation
Much of our knowledge of tumor cell extravasation is patterned after leukocyte transmigration through endothelium. It is well known that leukocytes arrest before transmigration. Similarly, tumor cell arrest can occur passively through mechanical lodging or can be allowed by cell-surface molecules.32–32 Endothelial cells are constantly shed from the blood vessel walls, creating temporary gaps to which tumor cells can more easily attach because basement membrane components are exposed.35–35 Vessel wall damage also attracts platelets and tumor cells associated with platelets, which is enhanced by fibrinogen expression on the endothelial cell surface.36,37 Fibrin blood clots at the sites of tumor cell arrest can further damage vessels, attracting more platelets and circulating tumor cells.38 Increased blood coagulation is often observed in patients with cancer as a result of elevated levels of thromboplastin, procoagulant A, and phosphatidylserine produced by tumor cells.39,40 The most severe manifestations of this hypercoagulation state were described by Trousseau many years ago. The induction of the enzymes involved in this state can also be enhanced by changes in the tumor microenvironment.41
Tumor cell arrest is allowed by endothelial cell P- and E-selectins that bind to the tumor cells and by tumor glycosylation patterns and cell-cell adhesion molecules such as integrins and CD44.42–47 Increased cell-surface expression of mucin carbohydrate is associated with increased metastatic potential in human colon carcinoma.48 Tumor clump formation additionally facilitates tumor cell arrest by increasing the number of adhesive interactions. ECM components such as fibronectin and laminin enhance tumor cell arrest, and administration of targeting peptides to fibronectin and laminin can reduce metastatic formation.49 Tumor cells may reside and grow within the intravascular space until the metastatic lesion physically breaks through the vessel.50 Tumor cells may also extravasate by inducing endothelial cell retraction, permitting cell attachment to the ECM.50 It is highly noteworthy that vascular endothelial growth factor (VEGF) increases vascular permeability and may permit extravasation through Src activation.51,52 Thus anti-VEGF therapy could potentially act to inhibit metastases by decreasing vascular permeability. In some cases, tumor cells direct their movement and invasion into new organ terrain by following migrating white blood cells and tissue motility factors.53
Proliferation
The final steps of metastasis involve the resumption of cell proliferation at the secondary site and induction of angiogenesis to supply oxygen and nutrients. The host tissue can influence tumor growth through autocrine, paracrine, and endocrine signals. However, it is the net balance of positive and negative signals that determines metastatic proliferation. This phenomenon can partially explain organ-specific metastasis, because only certain cells will be able to respond to tissue-specific proliferation-stimulating signals and leave their dormant state.54 For example, insulin-like growth factor–1 (IGF1), hepatocyte growth factor, and transforming growth factor–α (TGF-α) are highly expressed in the liver,57–57 and cancer cells from colon, breast, and bladder overexpress receptors for these ligands, such as epidermal growth factor receptor58–62 and c-met receptor,63 resulting in proliferation of metastatic cells in these tissues.
Angiogenesis
The formation of a new blood supply from preexisting vasculature is stimulated by an angiogenic “switch” that occurs when the ratio of inducers to inhibitors is increased. Inhibitors of angiogenesis include the ECM proteins thrombospondin and endostatin.66–66 Angiogenic inducers include VEGF, platelet-derived growth factor, basic fibroblast growth factor, TGF-β, and ephrin, along with their family members.67 Of these, VEGF is the best characterized and has successfully been targeted through the use of monoclonal antibodies and soluble receptors.68 VEGF increases angiogenesis by stimulating endothelial cells, mobilizing endothelial progenitor cells, stimulating outgrowth of pericytes that line the walls of mature blood vessels, and increasing vascular permeability, allowing macromolecules to traverse endothelium.69,70 Furthermore, VEGF is thought to be a key molecule for the homing of VEGF receptor (VEGFR)–positive bone marrow–derived progenitor cells involved in premetastatic niche formation (see the later discussion in this chapter)71 and for homing of VEGFR-positive tumor cells to metastatic sites.72 Recruitment of bone marrow–derived circulating endothelial cells additionally increases angiogenesis.73 Thus angiogenesis is important both for primary tumor and metastatic tumor growth, making it an attractive target.
Lymphatic Metastasis
The vascular and lymphatic systems have numerous connections, and metastasizing tumor cells can easily pass from one system to another. In tumors such as breast, melanoma, prostate, gastric, and colon cancer, metastasis to the nearby draining lymph node is often the first step in metastatic dissemination.74 Additionally, the presence of lymph node metastases correlates with decreased survival in these patient populations.75 Therefore examination of lymph nodes for metastases is an important parameter when determining staging, prognosis, and treatment options in patients with cancer.74
Tumor cells access lymphatic vessels via multiple mechanisms. The high internal pressure within tumors provides a net flow of interstitial fluid and dissociated tumor cells away from the tumor and into the lymphatics and the local draining lymph node.75 Additionally, a strong correlation exists between tumors that actively secrete prolymphangiogenic factors, including VEGF-C and VEGF-D, and the development of lymph node metastases.74 Lymphangiogenesis is a critical process driving lymph node metastasis. Overexpression of prolymphangiogenic factors by tumors is sufficient to promote lymph node and distant metastasis, whereas inhibition of lymphangiogenesis significantly inhibits lymph node metastasis.74 In addition to tumor cells, lymphatic endothelial cells (LECs) promote lymph metastases by actively recruiting tumor cells to the lymphatic vessels. LECs produce a variety of chemoattractant factors such as SDF1 and CCL21 that bind to cognate receptors CXCR4 and CCR7 expressed by tumor cells.74 Together these findings demonstrate that lymphatic metastasis is mediated at least in part by active processes that involve signaling between the tumor cells and lymphatic endothelial cells. Therefore therapeutic intervention of tumor cell–LEC signaling may offer therapeutic benefits in the treatment of metastatic cancer.
Transcoelomic Metastasis
In contrast to the majority of tumors that disseminate through the vascular or lymphatic systems, ovarian cancer predominately disseminates through the transcoelomic route. Ovarian cancer is a highly metastatic cancer, because approximately 70% of patients diagnosed with epithelial ovarian cancer present with stage III or IV disease in which the tumor has disseminated beyond the ovaries and pelvic organs to the peritoneum and mesothelial lining of abdominal organs.76 Transcoelomic metastases significantly contribute to morbidity in patients with ovarian cancer because these tumors are numerous and often obstruct vital organs in the abdomen, including the bowels.77 Additionally, transcoelomic metastases are associated with the formation of ascites that contribute to morbidity by increasing intraabdominal pressure, leading to impaired circulation and respiratory distress.77 Similar to hematogenous metastasis, transcoelomic metastasis occurs through a series of distinct steps that allow for successful colonization of tumor cells to mesothelial cells lining the abdominal organs.
Adhesion
The first step in transcoelomic metastasis is detachment from the primary tumor. Although this process in ovarian cancer is not completely understood, evidence suggests that EMT transition contributes to this process. Similar to the hematogenous tumors previously described, the EMT phenotype in ovarian cancer allows for the tumor cells to lose their cell-cell and cell-basement membrane adhesive properties. Loss of the cell adherin junction protein, E-cadherin, is thought to be a key event triggering peritoneal dissemination. E-cadherin loss is found in metastatic tumor cells and is associated with an invasive phenotype and poor patient survival in persons with ovarian cancer.78 In addition to active cell detachment, it has been proposed that ovarian tumor cells may also detach from the primary tumor through passive mechanisms in which tumor cells on the ovarian surface exfoliate off into the peritoneal fluid.77
Anoikis
Circulating tumors cells in the peritoneal fluid must resist anoikis for successful metastatic colonization. Several molecules have been shown to contribute to anoikis resistance in ovarian cancer. One of the first molecules to be linked to ovarian tumor cell resistance to anoikis is RAB25, a small guanosine triphosphatase that is increased in stages III and IV serous ovarian cancers.79 Increased RAB25 expression promotes anchorage-independent proliferation, prevents anoikis, and induces the aggressiveness of cancer cells in vivo. The immunomodulatory protein B7-H4 was also shown to protect ovarian cancer cells from anoikis. B7-H4 is an attractive therapeutic target for ovarian cancer therapy given the restricted expression of B7-H4 on normal tissue and its upregulation in the majority of ovarian tumors.80 Additional proteins including ADRB2 and ABHD4 have been shown to mediate resistance to anoikis in ovarian cancer cells and are additional therapeutic targets for the treatment of ovarian cancer.81,82
Immune Evasion
When circulating in the peritoneal fluid, ovarian tumor cells employ mechanisms to evade immunologic attack. Proinflammatory cytokines, complement proteins, and activated T cells are all found at significantly elevated levels in peritoneal fluid collected from patients with ovarian cancer.77 Perhaps the most striking evidence supporting a role for immunosurveillance in ovarian cancer is shown by clinical data demonstrating a 38% 5-year survival rate of patients who had CD3+ T cells contained within the ovarian tumor specimen compared with a 4.5% 5-year survival rate among patients without infiltrating T cells.83 Consistent with an immunologic response against tumors, patients with ovarian cancer produce antibodies against multiple tumor antigens including mutated p53, folate receptor α, IGF binding protein 2, HER2/neu, and topoisomerase II α.84 Patients with antibodies recognizing p53 have a significantly higher survival rate compared with those without p53 antibodies, further supporting the notion that ovarian tumors are under immunosurveillance.84
To overcome immunosurveillance, ovarian cancer cells have developed multiple mechanisms to evade and modulate the immune responses. Cancer cell spheroid formation is thought to protect tumor cells from immune attack by limiting the penetration of complement proteins and antibodies into the spheroids.77 Additionally, ovarian cancer cells actively suppress immune responses by expressing proteins that recruit regulatory T cells and myeloid-derived suppressor cells, and they produce proteins that inhibit complement activation.77
Peritoneal Implantation and Metastatic Growth
When establishing peritoneal implants, ovarian cancer cells attach to and invade into to the mesothelium covering the surface of all abdominal organs including the peritoneum, omentum, diaphragm, and bowel. The mesothelium is composed of a single layer of mesothelial cells that are attached to a basement membrane containing collagen types I and IV, fibronectin, and laminin.78 Integrins and the hyaluronic acid cell surface receptor CD44 are the key molecules mediating ovarian attachment to the mesothelial layer. Once attached, the MMP family mediates further adhesion and invasion into the basement membrane.78 Once at the distant site, the activation of VEGF and the induction of tumor angiogenesis plays a critical role in successful metastatic colonization. Together, these data demonstrate that many aspects of transcoelomic metastasis are mediated by active signaling processes between tumor cells and cells within the tumor microenvironment. Targeting these processes offers therapeutic strategies for the treatment of metastatic disease.85
Metastasis and Tumor Reseeding
Until recently, it was thought that tumor metastasis occurred in a unidirectional manner by which tumor cells leave the primary tumor site and colonize distant organs. Evidence now suggests that circulating tumor cells can also reseed the primary tumor. Kim and colleagues86 were among the first to demonstrate that circulating tumor cells return to the primary tumor site. Furthermore, they observed that the presence of circulating tumor cells in the primary tumor stimulated tumor growth, angiogenesis, and stromal cell recruitment.86 These data demonstrate that cells that have undergone metastatic selection respond to chemoattractant signals produced by the primary tumor and represent an aggressive subpopulation of tumor cells that promote tumor progression.
Tumor Microenvironment and Metastasis
Although the cellular intrinsic traits acquired by tumor cells as previously described are required for successful metastatic colonization, cellular and molecular factors within the tumor microenvironment also significantly contribute to metastatic progression. The tumor microenvironment contains a number of cell types that promote tumor progression, including cancer stem cells, endothelial cells and pericytes, bone marrow–derived and local stromal cells, immune cells, and fibroblasts (Fig. 3-3).87 Additionally, extracellular factors within the tumor microenvironment such as hypoxia promote tumorigenic phenotypes within these cell types.
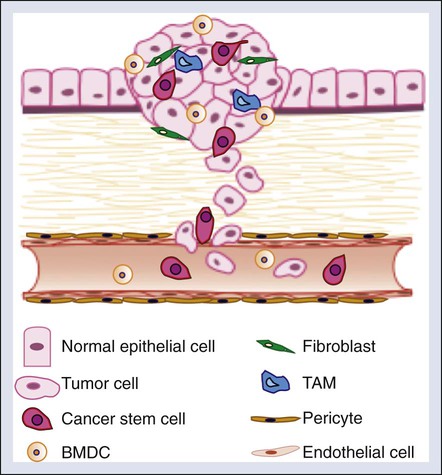
Cancer Stem Cells
The recent discovery of cancer stem cells has revolutionized our way of thinking about cancer. Our traditional view of tumors with a relatively homogenous population of tumorigenic cells has been significantly revised with the isolation and characterization of cancer stem cells. Stem cells are primal cells that retain the ability to renew themselves through cell division and can differentiate into a wide range of specialized cell types. Cells with stem cell properties have been identified in a variety of solid tumors including colon, breast, head and neck, and pancreatic tumors, glioblastomas, medulloblastomas, and melanoma.88 This often rare subpopulation of tumor cells exhibits enhanced tumor-initiating potential, and the ability to self-renew and differentiate into multiple cell types.
The origin of cancer stem cells remains unclear and may differ between tumor types. It has been hypothesized that cancer stem cells arise from either transformed resident stem and/or progenitors cells or may represent a dedifferentiated epithelial tumor cell. In intestinal cancer, mouse modeling studies have identified intestinal stem cells as the cells of origin. Cell type–specific deletion of APC in mice revealed that intestinal stem cell–specific deletion of APC leads to rapid cellular transformation with uncontrolled cellular growth and solid tumor formation. In contrast, deletion of APC in short-lived transit-amplifying cells was not sufficient to induce long-term tumor growth.89 Stem cell properties can also be acquired by tumor cells through EMT. Induction of EMT in immortalized human mammary epithelial cells was sufficient to induce the expression of stem cell markers, enhance self-renewal, and increase the number of tumor-initiating cells.90 Although the molecular mechanisms that drive the cancer stem cell phenotype in tumor cells remains largely unknown, a recent study shows that the transcription factors Slug and Sox9 drive EMT and the cancer cell phenotype in breast cancer cells.91 Future studies are eagerly awaited to further delineate the intracellular signaling pathways driving the cancer stem cell phenotype in vivo.
The role of cancer stem cells in multistage tumor progression, particularly with respect to metastasis, is poorly defined. Given that metastasis is an infrequent event that is achieved by only a small portion of cancer cells that reach distant sites, it has been hypothesized that cancer stem cells may be responsible for metastatic disease. Evidence to support this hypothesis has been generated in models of breast and pancreatic cancer. In the MMTV-PyMT model of breast cancer, Malanchi et al.92 observed that only the CD90+CD24+ population of cancer stem cells isolated from primary breast tumors contained cells with the ability to form metastases in the lung when introduced into the tail vein. Furthermore, in this study it was found that the extracellular matrix protein called periostin (POSTN), which is produced by fibroblasts and stromal tumor cells, is a critical factor in maintaining the cancer stem cell phenotype and metastatic potential in these cells.92 Similarly, in pancreatic cancer, lineage tracing studies showed that circulating pancreatic tumor cells exhibit EMT and cancer stem cell properties and initiate tumor formation. A critical role of inflammation in maintaining the ability of cancer stem cells to metastasize was observed in this study.93 Additionally, the extracellular protein tenascin C (TNC), which is expressed by stem cell niches and cancer cells, was also found to promote stem cell signaling and lung metastases in breast cancer cells.94 Collectively, these data strongly implicate the tumor microenvironment in promoting the cancer stem cell phenotype and the initiation of tumor metastasis.
Endothelial Cells and Pericytes
Tumor angiogenesis facilitates the hematogenous spread of metastatic tumors. Endothelial cells and pericytes are important structural and functional components of the tumor vasculature and thus both have been considered as potential targets in metastatic therapy.95 In addition to the important role that endothelial cells play in forming the framework of the vasculature, endothelial cells also play an active role in promoting metastasis by upregulating the expression of adhesion molecules, including E-selection, P-selectin, and vascular cell adhesion molecule 1, to induce tumor cell adhesion and migration.96
Similar to endothelial cells, pericytes are thought to play a protumorigenic role by supporting blood vessel maturation and function. Pericytes promote endothelial cell survival and stabilize the tumor vasculature.97 Therefore it has been proposed that targeting pericytes alone or in combination with endothelial cells may be an effective strategy in the treatment of cancer.98 However, clinical data indicate that low pericyte coverage is associated with metastasis and poor prognosis in a number of cancers.98 A recent study demonstrated that depletion of pericytes suppressed tumor growth and enhanced metastasis murine models of breast cancer.98 Enhanced metastasis was associated with increased levels of tumor hypoxia, EMT, and Met receptor activation.98 These findings indicate that pericyte coverage may be important to stabilize the tumor vasculature and prevent the hypoxic selection of aggressive tumor cells (see the discussion on hypoxia and metastasis later in this chapter). These findings raise concerns when considering antipericyte treatments in cancer therapy, especially in the setting of metastasis.