Bone Metastases
Robert E. Coleman and Ingunn Holen
• Bone metastasis is a major cause of (often prolonged) morbidity in persons with cancer.
• Bone metastasis is especially prevalent in persons with breast and prostatic cancer.
• Tumor cells are attracted to particular niches in bone and may remain dormant for prolonged periods, as controlled by microenvironmental cues.
• Osteolytic damage is mediated largely by stimulation of osteoclasts via tumor-derived cytokines; intermediary cells, including immune cells and osteoblasts, are involved.
• Differential diagnosis includes osteoporosis, degenerative disease, and Paget disease.
• The isotope bone scan is a sensitive test to detect the presence of skeletal pathology but gives little information about its nature.
• Structural information on skeletal damage from metastatic bone disease is best obtained by skeletal radiography supplemented with computerized tomography and magnetic resonance imaging.
• Positron emission tomography provides functional information that may aid in diagnosis.
• An assessment of the patient’s symptoms and activity status is essential.
• Skeletal radiography assesses response to treatment, but the information is delayed and the method is insensitive.
• Early indications of response of bone metastases to treatment can be obtained by monitoring biochemical markers of bone metabolism.
• Isotopic bone scanning is not useful in monitoring response to treatment.
• Antitumor treatments such as external beam radiotherapy, endocrine therapy, cytotoxic chemotherapy, targeted biological agents, and radioisotope therapy have a role in the multidisciplinary palliative treatment of metastatic bone disease.
• The bisphosphonates and the receptor activator of nuclear factor κ ligand inhibitor denosumab are inhibitors of osteoclast activity and have become important agents for the treatment of metastatic bone disease because they relieve symptoms, allow bone healing, and delay complications.
• Complications include pain, impaired mobility, pathological fracture, spinal cord compression, cranial nerve palsies, nerve root lesions, hypercalcemia, and suppression of bone marrow function.
• The treatment of choice for hypercalcemia is bisphosphonates in combination with intravenous rehydration.
• Orthopedic surgery has an important role in the treatment and prophylaxis of pathological fractures and spinal decompression and stabilization to relieve spinal cord compression or instability.
Incidence
Primary Tumors Leading to Bone Metastases
Primary bone cancer occurs predominantly among children and adolescents and is rare. By contrast, secondary bone cancer—particularly from carcinomas of the breast, lung, prostate, kidney, and thyroid—is common. The incidence of bone metastases from different primary sites recorded in postmortem studies is summarized in Table 51-1. Although the variability in these metastatic patterns is probably related to molecular and cellular biologic characteristics of both the tumor cells and those of the tissues to which they metastasize, other factors, such as vascular pathways and blood flow, are also important.1
Table 51-1
Incidence of Skeletal Metastases from Autopsy Studies
Incidence (%) of Bone Metastases | |||
Primary Tumor | No. of Studies | Median | Range |
Breast | 5 | 73 | 47-85 |
Prostate | 6 | 68 | 33-85 |
Thyroid | 4 | 42 | 28-60 |
Kidney | 3 | 35 | 33-40 |
Bronchus | 4 | 36 | 30-55 |
Esophagus | 3 | 6 | 5-7 |
Gastrointestinal tract | 4 | 5 | 3-11 |
Rectum | 3 | 11 | 8-13 |
Modified from Coleman RE. Clinical features of metastatic bone disease and risk of skeletal morbidity. Clin Cancer Res 2006;12(Suppl.):6243–9.
Breast cancer is the most common malignancy in most parts of the world, accounting for some 10% to 15% of all cancers, and it is the tumor most often associated with metastatic bone disease. Because of the potentially long clinical course of this disease, even after metastases have developed, morbidity from bone deposits presents a major problem for health care systems. Approximately 70% of patients dying from breast cancer will have radiologic evidence of skeletal metastases before death, and bone is the first metastatic site in more than 40% of persons with distant relapse. Median survival after a first relapse in bone is around 2 to 3 years and is significantly longer than that seen after a first relapse in visceral sites such as the liver.1 Although the management of metastatic visceral disease has improved in recent years, the prognosis after visceral relapse remains relatively poor, whereas bone metastases can be a protracted problem for many years. Favorable factors for a longer survival after first relapse in bone are low histologic grade, positive estrogen receptor (ER) status, long postoperative disease-free interval, and lack of development of extraosseous disease.2
The skeleton is by far the most common site of metastatic disease in persons with prostatic cancer. Unlike breast cancer, in which the lesions frequently show a mix of osteoblastic and osteolytic appearances radiologically, osteosclerotic disease predominates in prostate cancer. Nevertheless, computed tomography (CT) often identifies lytic areas within these ostensibly sclerotic lesions. Histologic and biochemical studies also have demonstrated increased bone resorption in metastatic prostate cancer.3 As in breast cancer, metastatic bone disease in persons with prostate cancer can follow a relatively long course over several years. Several studies have attempted to correlate the extent of skeletal metastatic involvement with survival in patients with advanced prostate cancer. A system based on the number of lesions identified by bone scintigraphy was predictive for survival,4 and a bone scan index to more accurately estimate the extent of skeletal involvement by tumor has been developed. When this index was used in 191 patients with androgen-independent prostate cancer, patients with low, intermediate, or extensive skeletal involvement had median survivals of 18.3, 15.8, and 8.1 months, respectively.5
Interest in personalized medicine is increasing, whereby biologic factors are used to predict the probability or distribution of recurrence. The association between ER expression and bone metastasis from breast cancer has been appreciated for >20 years.1 However, other markers, especially the noncollagenous bone proteins, have more recently been suggested as predictors of bone recurrence. These markers have included bone sialoprotein6,7 and C-terminal propeptide of type I collagen.8 Parathyroid hormone–related peptide (PTHrP) is expressed by a very high proportion of breast cancers in bone, but paradoxically, expression of PTHrP in the primary tumor is associated with a better prognosis and a lower incidence of metastasis.9
Gene expression profiling has identified genetic signatures that are strongly associated with prognosis, possibly outperforming conventional panels of clinical and pathologic criteria. However, the risk of metastasis per se does not necessarily predict for metastasis to specific sites. Several gene signatures have been described that appear predictive of metastasis to bone as opposed to dissemination to other sites.10 However, much more work is required to confirm the usefulness of this use of genetic profiling in clinical management.
Causes
Mechanisms of Metastases
Our view of the role of the primary tumor in metastatic growth fundamentally changed with the introduction of the concept of the “premetastatic niche.”11 In model systems, factors released by the primary tumor were found to direct migration of hematopoietic progenitor cells from the bone marrow to cluster in particular sites for future metastatic growth, preparing the “soil” to create a suitable environment for subsequent colonization by tumor cells. This model supports the notion that initiation of metastatic spread is an early event, probably occurring before the primary tumor becomes clinically detectable, rather than being associated with high tumor burden. The current view is that after leaving the primary site, tumor cells are attracted to particular “metastatic niches” in the hematopoietic bone marrow and that tumor cells may subsequently spread from this bone niche to other organs.12 The precise characteristics of the metastatic niche remain to be established, but prostate cancer models provide convincing evidence to support the notion that tumor cells occupy the hematopoietic stem cell niche.13 Once established in the niche, tumor cells may remain dormant for many years because of the control of the surrounding bone microenvironment.
Pathogenesis
Bone Remodeling
Bone is a highly specialized connective tissue comprising an unmineralized (osteoid) matrix composed predominantly of type I collagen and a mineralized component of hydroxyapatite crystals, which encloses the marrow space that contains a variety of marrow-residing cells (including osteoblasts, osteoclasts, bone marrow stromal cells, immune cells, mesenchymal stem cells, adipocytes, fibroblasts, and endothelial cells), platelets, fat, and interstitial fluid. During childhood and adolescence, bone is constantly shaped and remodeled, with peak bone mass being reached in early adulthood. Bone turnover continues throughout life, with up to 20% of the skeleton undergoing remodeling at any time to replace damaged bone and maintain skeletal integrity. The total duration of a remodeling cycle in young adults is estimated to be around 200 days. This process is essential for providing bone strength and is responsive to mechanical stress.14
Bone resorption is mediated by the osteoclast, a multinucleated giant cell derived from granulocyte-macrophage precursors, whereas bone formation is carried out by osteoblasts, which are derived from mesenchymal fibroblast-like cells. In a healthy person, bone resorption and bone formation are coupled and perfectly balanced in location, time, and amount. Bone remodeling is regulated by complex interactions between hormones, paracrine growth factors, and cytokines and involves interactions between osteoclasts and osteoblasts, as well as other cell types present in the bone microenvironment (Fig. 51-1).
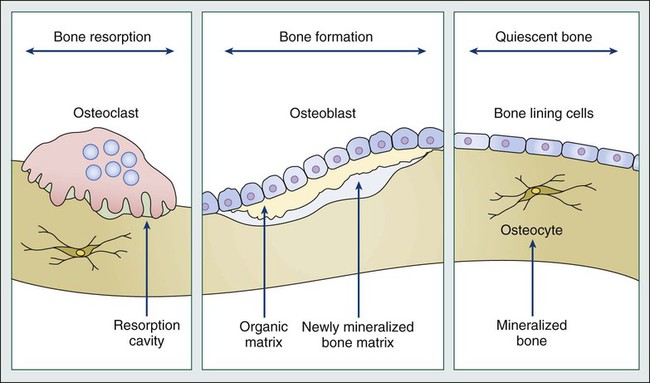
Recent studies in model systems have shown that direct contact with breast cancer cells cause substantial changes to the surrounding bone microenvironment at early stages, prior to the formation of bone lesions.15 The presence of tumor cells ultimately results in the development of cancer-induced bone disease that disrupts the fine-tuned balance between osteoblast and osteoclast activity, either causing excess bone resorption (as associated with lytic bone lesions), or increased levels of bone formation (as in osteosclerotic bone lesions) (Fig. 51-2). In many cases mixed lesions are present, which have both lytic and sclerotic features. In the late stages of cancer, tumor masses may also damage the skeleton by compression of vasculature and consequent ischemia.
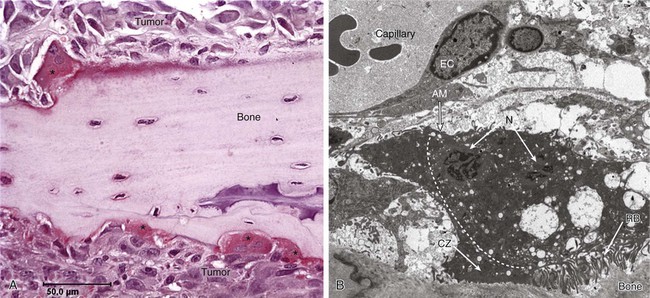
Tumor Cell-Bone Cell Interactions
Malignant cells secrete factors that stimulate osteoclastic activity both directly and indirectly, and the importance of interactions between malignant cells and the bone microenvironment in the development of bone metastases has been the focus of intensive research, as summarized in a recent review.16 Factors produced by tumor cells that cause increased bone resorption include prostaglandin E and a variety of cytokines and growth factors, such as transforming growth factor (TGF) α and β, epidermal growth factor, vascular endothelial growth factor, tumor necrosis factor (TNF), and interleukin (IL) 1, 6, 8, and 11 (Fig. 51-3).
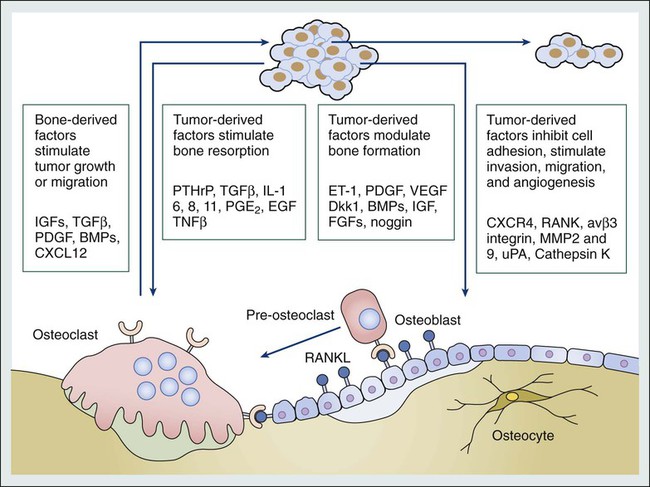
Several proteolytic enzymes are proposed to be involved in the early phases of formation of bone metastases, including matrix metalloproteinases (MMPs; e.g., MMP-2 and MMP-9) and cathepsin K.17 Normal bone trabeculae are lined by a thin layer of uncalcified matrix, which protects the calcified bone from osteoclastic activity, and the action of proteolytic enzymes might be a prerequisite for osteoclastic bone resorption. In addition, MMPs are involved in formation of bone metastases both through their ability to degrade basement membrane and facilitate tumor cell dissemination but also by causing release of growth factors and cytokines bound to the bone matrix, thereby supporting further tumor cell proliferation.18 Malignant cells might also increase bone resorption by stimulating tumor-associated immune cells to release osteoclast-activating factors. It has been shown that human melanoma cells produce a factor that stimulates macrophages to release TNF and IL-1 in vitro. Furthermore, purification of a cytokine-releasing factor from medium conditioned by melanoma cells has identified it as granulocyte-macrophage colony-stimulating factor, which activates osteoclastic bone resorption.19
In addition to the local paracrine factors just described, osteoclastic activity can also be stimulated in malignant disease by systemic factors, particularly PTHrP.20 This peptide is immunologically distinct from parathyroid hormone, but the two hormones have significant homology at the amino terminus of the molecule, which is necessary for osteoclast stimulation. Ectopic production of this hormone, particularly in persons with lung cancer, is a cause of osteoclastic bone resorption and hypercalcemia even in the absence of bone metastases.
The importance of PTHrP in the pathogenesis of osteolysis induced by metastatic breast cancer has been elegantly elucidated.20 In bone metastases, the secreted PTHrP acts in a paracrine manner to stimulate osteoclastic bone resorption, which not only leads to skeletal damage but also appears to confer a selective growth advantage on the tumor cells. This advantage is conveyed by the release of factors for tumor growth from bone during the osteolytic process. Hence a vicious circle is established in which the growth of metastatic cells is potentiated, leading to enhanced osteolysis and secretion of further growth factors to stimulate tumor cell proliferation. This paracrine activity occurs even in the absence of hypercalcemia or increased circulating PTHrP levels. PTHrP stimulates osteoclastic resorption by increasing osteoblast and stromal cell production of receptor activator of nuclear factor–κB (RANK) ligand (RANKL). RANKL binds to its receptor, RANK, on osteoclast lineage cells, resulting in differentiation to mature osteoclasts and stimulation of osteoclast activity.
In the normal bone environment, osteoblast secretion of osteoprotegerin (OPG) neutralizes RANKL, terminating its stimulatory effects on osteoclasts.21 The MCF-7 estrogen-dependent breast cancer cell line has been found to decrease osteoblastic OPG messenger RNA levels, enhancing osteoclast formation. This imbalance is compounded by release of TGF-β and insulin-like growth factor (IGF1) from resorbing bone, which further promotes tumor production of PTHrP, perpetuating the cycle of osteolytic bone destruction. The OPG-RANK-RANKL triad of molecules are central not only to normal bone physiology but have a key role in the development of bone metastasis, with several different tumor cell types producing one or more of these factors.22
Sclerotic Bone Metastases
Although osteolytic disease is usually most evident at sites of bone metastases, osteosclerosis sometimes can predominate, particularly in prostate cancer. Prostate cancer, in contrast to breast cancer, tends to cause osteoblastic lesions in bone, leading to dense, sclerotic-looking metastases on plain radiographs, and osteoblast growth factors such as TGF-β and platelet-derived growth factor have been purified from prostatic tumor cells. One of the factors that might be involved in prostatic bone lesions is the growth factor endothelin-1 (ET-1), which is produced by prostate cancer cells.23 Circulating levels have been found to be increased in patients with osteoblastic bone metastases from androgen-refractory prostate cancer, compared with patients whose cancer is confined to the prostate and normal control subjects. ET-1 has been found to stimulate osteoblast activity in animal models and to inhibit osteoclast activity, whereas antagonists of endothelin have been found to inhibit bone formation in vivo.24 Furthermore, a recent study found that ET-1 production by prostate cancer cells is reduced by androgens but is stimulated in androgen-insensitive prostate cancer cells by factors such as TGF-β.25 This finding is clinically relevant, because metastatic prostate cancer typically develops androgen resistance. It is possible that PTHrP is also involved in the pathogenesis of prostate cancer bone metastases, because co-expression of PTHrP and its receptor has been found in both the primary tumor and in bone metastases of patients with prostate cancer.26
Evidence from morphometric studies and measurement of urinary markers of osteolytic bone resorption has led to the hypothesis that osteoclastic bone resorption initially is important in prostate cancer and is followed by intense osteoblastic activity.27 This scenario might not always be the case, however. When the prostate cancer cell line PC-3 is implanted into the tibia of severe combined immunodeficiency (SCID) mice, osteolytic lesions develop, possibly through secretion of RANKL.28 However, when another prostate cancer cell line (LAPC-9) was used, osteoblastic lesions developed even when no osteoclasts were present. Therefore these authors concluded that osteoclastic activity might not be a prerequisite for the formation of osteoblastic lesions.
Interest is increasing in the role of the osteoblasts in bone metastases, and it has recently been established that the Wnt signaling system plays a key role in bone development and turnover.29 Subsequent studies have found that several molecules in this system are implicated in the development of bone metastases.30 In prostate cancer, tumor-derived Wnt induces osteoblastic activity in bone metastases, which in the early stages of disease may be counteracted by the presence of the Wnt agonist dickopf-1 (DKK1), thereby favoring lytic lesions. In the later stages of progression of prostate cancer bone metastases, the balance between Wnt and its inhibitors appears to be shifted toward Wnt, favoring osteoblastic lesions.
In myeloma bone disease, bone marrow stromal cells are important for the pathogenesis of multiple myeloma. IL-6 appears to be an important growth and survival factor for myeloma cells and for conferring resistance to treatment with dexamethasone, a commonly used treatment for multiple myeloma. MMPs, which are known to be important in normal and malignant remodeling, also contribute to the pathogenesis of myeloma. Bone marrow stromal cells secrete interstitial collagenases (MMP-1) and gelatinase A (MMP-2). MMP-1 initiates bone resorption, degrading type I collagen, which becomes a substrate for MMP-2. Malignant plasma cells have been found to upregulate MMP-1 and activate MMP-2.31
Dysregulation of the Wnt signaling system has also been implicated in myeloma bone disease. The production of DKK1 (an inhibitor of osteoblast differentiation) by myeloma cells is reported to be associated with the presence of lytic bone lesions,32 whereas inhibition of DKK1 prevents the development of lytic bone disease in models of multiple myeloma.33
Diagnosis
Differential Diagnosis
In all cases, imaging tests are necessary and must be interpreted in conjunction with the clinical picture and—when appropriate—serum tumor markers. Occasionally, performing a bone biopsy under radiologic control or through an open operation is necessary. The typical clinical, radiologic, bone scan, and biochemical abnormalities of the more common skeletal pathologies are summarized in Table 51-2.
Table 51-2
Clinical, Radiologic, and Biochemical Features of Common Skeletal Disorders That May Mimic Bone Metastases
Diagnosis | Clinical Features | Radiologic | Biochemical |
Bone metastases | Pain common | Bone scan very rarely completely normal | Alkaline phosphatase usually elevated |
Usually multiple sites | Discrete lytic or sclerotic lesions on radiographs | Increased urinary markers of bone resorption | |
Axial skeletal involvement typical | Fracture/vertebral pedicle destruction | Hypercalcemia common | |
Soft tissue extension on CT/MRI | |||
Degenerative disease | Elderly | Spinal involvement results in symmetric increased tracer uptake on scan | Usually normal |
Limb involvement common | Radiograms usually confirmatory | ||
Pain and stiffness | |||
Long history | |||
Osteoporosis | Elderly female | Normal scan unless recent fracture or vertebral collapse | Usually normal serum parameters |
Painless unless fracture or vertebral collapse | Diffuse osteopenia on radiograms | Slight elevation of urinary indices | |
Normal marrow on MRI | No hypercalcemia | ||
Paget disease of bone | Elderly | Diffuse involvement of bone on scan | Alkaline phosphatase greatly elevated |
Bone deformity common | Sclerotic expanded appearance on radiograms | Increase in urinary hydroxyproline excretion | |
Involved site warm as a result of increased blood flow | Hypercalcemia very rare | ||
Skull often involved and enlarged | |||
Traumatic fractures | History of trauma usual | Intense linear uptake on scan | Usually normal |
Spontaneous rib fractures after chest wall irradiation common | Rib lesions typically aligned, not randomly distributed | ||
No evidence of destruction around fracture site on radiographs (unless radiation induced) |
Diagnostic Methods
Skeletal Radiography
Lytic metastases are the most common type arising from breast, lung, thyroid, renal, melanoma, and gastrointestinal malignancies (Fig. 51-4). Thinning of trabeculae occurs, and the margins are usually ill defined, representing regions of partially destroyed trabeculae between the central destruction and the radiologically normal bone. The width of the margin reflects the aggressiveness of the lesion, with a narrow zone of transition in the less aggressive lesions. If the metastasis is in the medulla, endosteal scalloping could be present, whereas cortical lesions produce subperiosteal scalloping or a focal cortical defect.
Sclerotic metastases are usually from prostate cancer but also arise from breast, lung, and carcinoid tumors (Fig. 51-5). Excessive new bone formation gives rise to thickened, coarse trabeculae, which usually appear on radiograms as nodular, rounded, fairly well-circumscribed sclerotic areas. Sometimes less well-defined, mottled, irregular areas of increased bone density appear, which can coalesce to produce a diffuse sclerotic appearance to the skeleton.
Radionuclide Bone Scan
A bone scan is generally performed by acquiring multiple images of the skeleton 3 to 4 hours after the intravenous injection of technetium-99m–labeled bisphosphonate. If a lesion is identified, further investigation is necessary, particularly when it is solitary. A suggested protocol for investigation is shown in the accompanying algorithm (Fig. 51-6). Appropriate plain radiographs of a focal lesion should be obtained in the first instance. If findings of these radiographs are normal and yet clinically a metastasis is likely, CT or magnetic resonance imaging (MRI) of the area could be diagnostic.
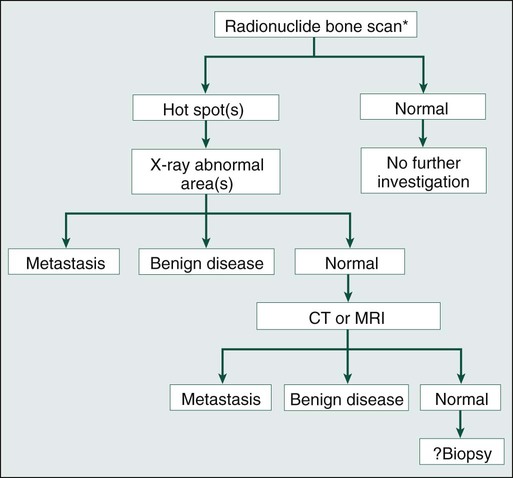
Although bone scan appearances are nonspecific, recognizable patterns of bone scan abnormalities might suggest a specific diagnosis. Metastases are usually multiple, irregularly distributed foci of increased tracer uptake that do not correspond to any single anatomic structure (Fig. 51-7). Generally they affect the axial skeleton, but metastatic disease can involve the appendicular skeleton, and approximately 7% of patients have involvement of the distal skeleton, with the proportion increasing in certain tumor types such as renal cell carcinoma.34
Because detection of a lesion depends on the presence of a focal increase in osteoblast activity, a false-negative scan will occur when pure lytic disease is present. A false-negative scan is typical of multiple myeloma, which is best investigated radiographically, but a false-negative scan also can occur in other tumors when rapidly growing lytic lesions are present. In extreme cases where significant bony destruction has occurred, a photon-deficient area (cold spot) can develop (Fig. 51-8). Sclerotic metastases, on the other hand, are generally clearly visualized on the bone scan, with the only exception being very slow-growing metastases, in which the alteration in metabolic activity is so subtle that it might not be distinguishable from normal background activity.
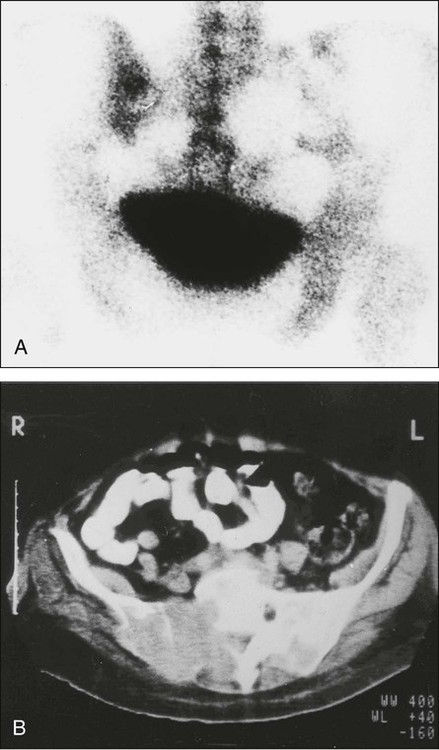
When extensive skeletal metastases are present, the focal lesions can coalesce to produce diffusely increased uptake—the so-called “super scan” of malignancy (Fig. 51-9). This scenario occurs most often in prostate cancer but also is seen in other tumors, such as breast cancer. Increases in the contrast between bone and background soft tissue and faint or absent renal images are the typical appearances.
Computed Tomography
A CT scan produces images with excellent soft tissue and contrast resolution. Bony destruction and sclerotic deposits are well visualized (Fig. 51-10), and any soft tissue extension of bone metastases is demonstrated clearly. CT can help with the diagnosis of spinal metastases and is particularly useful to localize lesions for biopsy.35
Magnetic Resonance Imaging
MRI permits imaging of the entire spine in the sagittal plane (Fig. 51-11). With a T1-weighted scan acquisition, the solid constituents of cortical bone give no signal on MRI and appear black, whereas the high water content of fat and bone marrow results in strong signals, making these tissues appear white. This signal is variable, depending on the pulse sequence used. Detection of bone metastases by MRI depends on differences in MR signal intensity between tumor tissue and normal bone marrow. Metastatic tumor is therefore visualized directly, in contrast to the indirect changes observed by x-ray or radionuclide bone scanning. Like CT, MRI has proved useful for evaluating patients with positive bone scans and normal radiograms and for elucidating the cause of a vertebral compression fracture. MRI is excellent for demonstrating bone marrow infiltration and is more sensitive than a bone scan for the early detection of spinal metastases.36
Positron Emission Tomography
Scanning with fluorine 18–labeled fluorodeoxyglucose (18F-FDG)-positron emission tomography (PET) provides the opportunity to visualize function. However, its role in the routine identification of bone metastases is far from clear. FDG has the advantage of demonstrating all metastatic sites, and in the skeleton it is assumed that its uptake occurs directly into tumor cells. For bone metastases from breast and lung cancer, FDG-PET has similar sensitivity, though poorer specificity, as the isotope bone scan. FDG-PET is less sensitive than a bone scan for prostate cancer, whereas for multiple myeloma (presumably because FDG is identifying marrow-based disease at an early stage), FDG-PET is clearly better than a bone scan.36
Biochemical Markers of Bone Metabolism
The effects of tumor cells on bone cell function can influence serum and urinary levels of biochemical markers of bone metabolism. In recent years, the number of available markers and the clinical relevance has increased rapidly; their value in the diagnosis of bone metastases has been studied in several tumor types. Table 51-3 lists some of the currently available bone resorption and formation markers. Their potential roles in malignancy have been extensively reviewed.39–39
Table 51-3
Biochemical Markers of Bone Resorption and Formation
Resorption | Formation |
URINE | SERUM |
Calcium | Alkaline phosphatase |
Hydroxyproline | Osteocalcin |
PYD | PICP |
DPD | PINP |
NTX | |
Crosslaps | |
Free DPD | |
Free PYD | |
Galactosyl hydroxylysine | |
SERUM | |
Calcium | |
ICTP | |
Galactosyl hydroxylysine |
Bone Markers as Predictive and Prognostic Indicators
Because commonly used radiographic methods do not detect bone metastases in the very early stages of development, the question arises whether serial measurements of bone markers might identify the impending development of bone metastases. Thus far, data relating to this question are inconclusive. Elevated levels of bone markers, notably bone sialoprotein,6,7 N-terminal propeptide of procollagen type 1,40 and C-terminal telopeptide of type 1 collagen,8 have been shown to have prognostic value; patients with levels above a defined threshold had a significantly greater probability of bone recurrence. However, in an individual patient, bone marker levels are unlikely to be particularly useful in making a diagnosis of metastatic bone disease before there is imaging evidence or helping to establish the nature of a solitary imaging abnormality.
Once bone metastases have developed, bone markers can provide useful prognostic information. Clinical evidence of correlations between bone marker levels and patient outcomes have been reported from retrospective analyses of several large trials in patients with bone metastases.3,41 Elevated bone marker levels during the course of the study correlated with negative clinical outcomes from a range of solid tumors or myeloma. Specifically, patients with elevated urinary N-telopeptide of type I collagen (NTX) levels either at baseline or at their most recent assessment had an approximate twofold increase in their risk of disease progression, an approximate two- to threefold increase in their relative risk of skeletal-related events (SREs) compared with patients with low NTX levels, and a two- to fivefold increased risk of death. In contrast, other than in prostate cancer, bone-specific alkaline phosphatase levels were not a consistently strong prognostic indicator.3
NTX levels have also been shown to provide valuable prognostic information in a larger analysis of patients receiving bisphosphonate treatment for bone metastases from a wide range of solid tumors and multiple myeloma.41 Among patients with solid tumors, elevated NTX levels were associated with a twofold increased risk of disease progression (P < .001). Furthermore, elevated NTX levels were associated with a four- to sixfold increased risk of dying upon study in all patients with solid tumors (P < .001 for all).
Evaluation of the Patient
A variety of treatments including radiotherapy, endocrine treatment, chemotherapy, and bone-targeted agents are used for the treatment of metastatic bone disease, and evaluation of their effects is important for both routine clinical practice and research. The current imaging methods used to assess response to these treatments are qualitative and routinely include plain radiographs, radionuclide bone scans, and, in particular situations, CT scans. Assessing the response of bone metastases to therapy is notoriously difficult; the events in the healing process are slow to evolve and are quite subtle, with sclerosis of lytic lesions only beginning to appear 3 to 6 months after the start of therapy and taking more than a year to mature.42
Bone is the only site of metastatic disease that has separate criteria for evaluation of response to treatment, based on bone repair and destruction rather than on changes in tumor volume. A complete review of the bone radiographs since the start of treatment is necessary to evaluate response, which is a slow and imprecise process. Radiologic assessment of response is based on radiographic evidence of bone healing.42 It is generally accepted that sclerosis of lytic metastases with no radiologic evidence of new lesions constitutes tumor regression (a partial response). Confounding factors include the appearance of sclerosis in an area that was previously normal on the radiograph. This finding could represent progression of a new metastasis but could also indicate a response, reflecting a radiographic example of the healing flare phenomenon within a lesion that was present at the start but was not destructive enough to be radiographically visible. Interpretation is further complicated by variations in film exposure and the effects of overlying bowel gas. The evaluation of response in osteosclerotic lesions is even more difficult; most patients with sclerotic metastases are eventually classified as either having “no change” in response to therapy or as being unassessable. In these cases, decisions about the efficacy of treatment must be based on symptomatic response, changes in tumor markers, or (when present) change in extraskeletal disease.
Although we recognize that the changes seen on serial radiograms remain the “gold standard” for evaluating response to therapy, new methods of assessing response are needed, both to improve patient management and to evaluate specific treatments. Several alternatives or adjuncts to assessment based on plain radiograms have been suggested.38,42,43 None is ideal, each having advantages and disadvantages as outlined in the following sections.
Assessment of Symptoms and Activity Status
The relief of symptoms is the principal aim of palliative therapy and rationally should be the most important marker of response to treatment. The use of pain as a marker of response in clinical trials has not found universal acceptance, however, and there is still no single internationally accepted pain questionnaire in the field of oncology, although the Brief Pain Inventory is frequently used in clinical trials. Subjective response to treatment for bone disease requires information on pain intensity, analgesic consumption, and mobility.42
Quality of life (QOL) assessment is now an important aspect of clinical trials methodology and, notwithstanding all the difficulties of analysis and interpretation, well-validated generic tools such as the Functional Living Index in Cancer patients and the European Organization for Research and Treatment of Cancer (EORTC) QOL-C30 questionnaire can provide useful information on subjective response to treatment in the routine clinical setting. A specific QOL tool for patients with bone metastases has recently been validated (EORTC-BM22)44 and could be considered for assessment of patient outcomes in routine clinical practice and in clinical trials.
Imaging of Bone Metastases
Although the plain radiograph remains the assessment tool used to judge response in many clinical trials, it is clearly an inadequate technique. The lack of precision for radiographic assessment of response is exemplified by the observation that, in terms of survival, patients with radiographic evidence of sclerosis (partial response) and patients with no change in radiographic appearance for at least 3 months have a similar outcome.42
The use of bone scanning for assessment of response to therapy has always been contentious and is certainly unreliable when lytic metastases predominate. A reduction in the intensity and number of lesions (hot spots) on the bone scan was previously considered to represent response, and progressive disease was assumed if an increase in intensity or number of hot spots was seen. This interpretation is too simplistic, however. After successful therapy for metastatic disease, the healing processes of new bone formation cause an initial increase in tracer uptake (akin to callus formation), and scans performed during this phase are likely to show increased intensity and an increased number of hot spots. After treatment for 6 months, the bone scan appearances might improve as the increased production of immature new bone ceases and isotope uptake gradually falls. This “deterioration” followed by subsequent “improvement” in the bone scan appearances after successful therapy has been termed the “flare response” and is now a well-recognized phenomenon in both breast and prostate cancers (Fig. 51-12). Conversely, a reduction in isotope uptake can occasionally be seen in rapidly progressive disease, when overwhelming bone destruction allows little chance for new bone formation, sometimes culminating in a photon-deficient (cold spot) lesion on the bone scan.
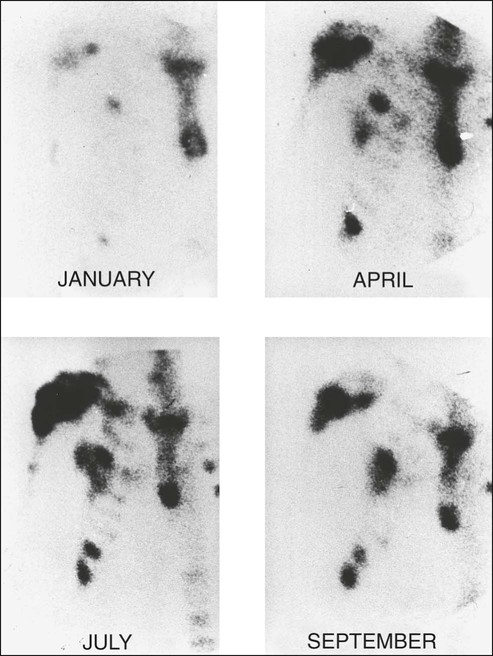
The role of PET scanning in assessment of response has become clearer in recent years.45 The regular, well-defined tumor margins that are necessary for reproducible anatomic measurements are of lesser importance in functional imaging. Functional imaging criteria, such as the recently developed Positron Emission Tomography Response Criteria in Solid Tumors, have allowed response to be measured in the absence of anatomic change through assessment of metabolic activity but have not yet been accepted for routine use.45
Tumor Markers
In breast cancer, unlike in germ cell malignancies, no highly specific tumor marker is available for either diagnosis or monitoring of disease. However, some breast tumors do produce tumor antigens that can be detected by radioimmunoassay. The most widely studied are carcinoembryonic antigen, which is elevated in 50% to 80% of patients with metastatic breast cancer, and carcinoma antigen (CA) 15-3, which is elevated in 60% to 90% of persons with advanced disease. Dynamic changes must be interpreted with caution. Although some patients show the expected increase in markers with progression and decrease in markers with regression, others who respond show an initial surge in tumor marker followed later by the expected decline. A combination of carcinoembryonic antigen, CA 15, and erythrocyte sedimentation rate may provide an indication of response. In a study in patients with metastatic breast cancer, a significant correlation with clinical assessment of response was found using Union Internationale Contre Cancer criteria at 2, 4, and 6 months after systemic endocrine treatment. A multicenter evaluation of the same index confirmed that changes in the markers were in line with and often predated therapeutic outcome criteria for both remission and progression.46
Prostate-specific antigen (PSA) is a marker of prostatic pathology and can be elevated in any prostate disease, although the highest tissue production occurs in prostate cancer. PSA has proved useful in the early diagnosis, staging, and follow-up of patients.47 The level of PSA is dependent on the volume of cancer, the volume of benign prostatic hypertrophy in the prostate, and the differentiation of the tumor, with less production of PSA from poorly differentiated tumors. PSA levels usually decline after androgen deprivation therapy (ADT) is initiated and generally provide a reliable guide to response; elevations of PSA usually antedate other clinical evidence of progression by at least 12 months.48 The PSA doubling time is a useful predictor of overt metastases in castrate-resistant prostate cancer (CRPC)48 and has been used to select suitable patients to enable evaluation of new treatments developed to delay or prevent metastasis.
Biochemical Assessment of Response
The stimulation of osteoclast function that results in osteolysis and disruption of the normal coupling between osteoblast and osteoclast function leads to changes in a variety of biochemical parameters. When treatment is prescribed for a patient with bone metastases, the effects of that treatment on the tumor cell population will influence bone cell activity. These changes can be appreciated within the first few weeks of starting effective therapy; for this reason, biochemical markers that reflect the rates of bone formation and resorption, respectively, can provide an early assessment of response to treatment.38,39
More recently, attention has turned to the possible use of collagen cross-link measurements for the assessment of response in bone. Several pilot studies have shown that bone resorption markers will usually fall if disease is controlled, whereas bone formation markers show an initial rise in the first 1 to 3 months of successful treatment because of the flare response followed by a fall as the response is consolidated.39,42
Although it is now well accepted that bone-targeted systemic therapy—particularly bisphosphonates—can reduce morbidity of skeletal metastases of breast cancer substantially, the optimization and timing of these therapies remain to be established. Bone markers potentially offer a powerful and relatively simple tool to assist the clinician in developing the most appropriate treatment strategies. Moreover, there is a prospect of using bone markers to tailor treatment to the individual patient. However, this prospect remains a research question, and use of bone markers is not recommended by current guidelines.49,50
Evidence indicates that the individual pretreatment values of a bone marker correlate with response to treatment. In patients with metastatic bone pain, normalization of bone resorption markers correlated with response to treatment.51 Clinical benefit, as indicated by an improvement in a pain score, was seen only among the patients achieving a normal bone resorption rate after administration of pamidronate. No response was seen in the patients with persistently elevated levels, which suggested that the aim of bisphosphonate therapy should be to produce a fall in marker levels, preferably into the normal range. A subsequent study has shown that this principle may be extended to the use of bone markers to distinguish between the benefits of different bisphosphonates.52
The ability of bisphosphonate therapy to reduce the frequency of skeletal complications also seems to be correlated with a reduction in bone resorption markers. Retrospective analyses of large randomized trials with zoledronic acid have shown that among patients with solid tumors, elevated NTX levels despite treatment were associated with a significant two- to threefold increased risk of both skeletal complications and death (P < .001).53
Treatment
In general, the treatment of bone metastases is aimed at palliating symptoms, with cure only rarely a realistic aim (e.g., in lymphoma); treatment varies depending on the underlying disease. External beam radiotherapy, endocrine treatments, chemotherapy, and radioisotopes are all important. In addition, orthopedic intervention may be necessary for the structural complications of bone destruction, and some patients with bone metastases experience hypercalcemia, which requires specific treatment (see Chapter 37). Optimal management requires a multidisciplinary team that includes not only medical and radiation oncologists, orthopedic surgeons, general physicians, radiologists, and nuclear medicine physicians but also palliative medicine specialists and the symptom control team.
A schema for the management of bone metastases is shown in the accompanying algorithm (Fig. 51-13). Treatment decisions depend on whether the bone disease is localized or widespread, the presence or absence of extraskeletal metastases, and the nature of the underlying malignancy. Radiotherapy is frequently relevant throughout the clinical course of the disease. Resistance to systemic treatments can be expected to develop, necessitating periodic changes of therapy in an effort to regain control of the disease.
External Beam Radiation Therapy
Shortly after the discovery of x-rays by Roentgen in 1895, radiation therapy was tried as an empiric treatment for bone metastases, and relief of bone pain was observed. Since these early reports, radiotherapy has become established as the treatment of choice for the palliation of painful single sites.54
There is no doubt that local irradiation is effective in relieving bone pain. Overall, response rates of around 85% are reported, with complete relief of pain achieved in half of patients.54 Pain relief usually occurs rapidly, with more than 50% of responders showing benefit within 1 to 2 weeks. If improvement in pain has not occurred by 6 weeks or more after treatment, it is unlikely to be achieved.
Traditionally, treatment techniques and doses have varied considerably, with prolonged fractionated treatments preferred in North America and single treatments or short fractionation schedules most frequently used in Europe. A number of randomized trials have been performed comparing fractionation schedules. No particular approach seemed to be superior in terms of pain relief. Several trials have shown no difference in outcome between fractionated treatment and a single treatment, notably a large Dutch trial of 1157 patients with painful bone metastases who were randomized to receive either a single fraction of 8 Gy or a treatment schedule of six fractions of 4 Gy each.55 No statistically significant differences in pain response, analgesic consumption, treatment adverse effects, or QOL were identified.
Subsequently, a metaanalysis of eight trials comparing single versus multiple fractions revealed similar response rates; 1011 of 1391 (73%) treated with a single fraction and 958 of 1321 (73%) who received multiple fractionation achieved a symptomatic response.56 Hence accumulating evidence strongly favors single-fraction radiotherapy as the treatment of choice for many patients with painful bone metastases.57
The option of retreatment of a painful site requires careful review of previous treatment fields, dose, and fractionation and the time since the initial treatment to ensure that normal tissue tolerance, particularly of the spinal cord, is not exceeded. When higher radiation doses have been administered, retreatment may be precluded; targeted radioisotope therapy is an alternative approach in such patients. After a single 8-Gy fraction, however, retreatment is usually possible, with more than 50% of patients who have recurrence of bone pain responding to a second 8-Gy treatment—a finding that further supports the move occurring in many centers toward single-fraction treatments for routine palliation of metastatic bone pain.57
When painful bone metastases are present at multiple scattered sites, wide-field/hemibody irradiation is an alternative to treating each site individually with local irradiation, although this approach is not commonly used. With use of single fractions of 6 to 7 Gy to the upper hemibody and 6 to 8 Gy to the lower hemibody, pain relief is reported in about 75% of patients, often occurring rapidly and sometimes within 24 to 48 hours of treatment.54 Hemibody irradiation is inevitably more toxic than localized external beam treatment. Virtually all patients who receive lower hemibody irradiation experience gastrointestinal toxicity. The serious toxicities of hemibody irradiation are bone marrow suppression and occasional radiation pneumonitis. Significant bone marrow suppression is seen in about 10% of patients receiving a single half-body treatment and in most patients who have sequential upper and lower hemibody treatment, potentially compromising the use of subsequent chemotherapy. Despite these adverse effects, wide-field irradiation is probably underused in the palliation of advanced stages of malignancy.
Targeted Radioisotope Therapy
Therapeutic radionuclides have characteristics different from those used for diagnostic purposes. Ideally, radionuclides for therapy should have a fairly long half-life, allowing adequate accumulation of the radiopharmaceutical within the target tissue. The γ-particle emission should be small to reduce unwanted radiation to nontarget tissues but present in sufficient amounts to permit localization of the radionuclide by gamma camera imaging. Predominantly α- or β-emitting radionuclides are the most suitable, but apart from 131I, they have been very expensive to produce. Although many α- and β-emitting radionuclides exist, relatively few have been evaluated clinically.
Because follicular carcinoma of the thyroid commonly metastasizes to bone, the treatment of bone metastases with 131I is now well established, and excellent results can be obtained. In patients with a significant uptake of 131I into the metastases, long-term palliation is usually possible.58 Treatment is generally well tolerated, although some patients experience a radiation reaction in the salivary glands. More important, however, is the 1% to 2% incidence of radiation-induced leukemias.
Neuroblastomas, and tumors of neuroectodermal origin in general, frequently metastasize to bone. Some 90% of these neoplasms incorporate the radiopharmaceutical agent 131I-meta-iodo benzyl guanidine (131I-MIBG), which has now been used to treat many children with metastatic neuroblastoma. Bone marrow suppression is often severe, and ideally, bone marrow harvesting should be performed as a precautionary measure for patients with extensive bone metastases.
Strontium-89 is a β emitter that imitates calcium and is taken up preferentially at sites of new bone formation. It has been shown to localize at the sites of prostatic bone metastases, with greater accumulation occurring in the metastatic lesions than is observed in normal bone, and whole-body retention of 89Sr treatments ranging from 11% to 88% depending on the degree of skeletal involvement.59 The biological half-life of strontium in metastases is long compared with that of normal bone. The radiation dose to individual vertebral metastases from a single 150-MBq dose of 89Sr has been shown to vary from 9 Gy to 92 Gy depending on the extent of metastatic spread. Of great clinical importance is the low dose of radiation to the bone marrow, which is only about one tenth of that to the bone metastases. The selective β-particle irradiation provides pain relief in up to 80% of patients, with 10% to 20% becoming pain free. On average, the response lasts for 6 months, with only mild hematologic toxicity.59
89Sr has been compared with conventional radiotherapy (hemibody or local) for the palliation of bone metastases from prostate cancer and was at least as effective as external beam radiotherapy in achieving palliation and prevention of new sites of pain.60
Samarium-153 is both a β- and a γ-ray emitter, making it suitable for combined therapy and imaging. It has a short half-life (46 hours) and, linked to ethylene diamine tetramethylene phosphonate (EDTMP), concentrates preferentially in skeletal metastases. Clinical reports indicate that 153Sm-EDTMP can provide excellent palliation of pain in both breast and prostate cancer.61 The short half-life of 153Sm also makes 153Sm-EDTMP suitable for repeated treatments.
Rhenium-186, an investigational radiopharmaceutical, is also a β- and a γ-ray emitter that is complexed with hydroxyethylidene diphosphonate for therapeutic use. Encouraging clinical results with mild and reversible bone marrow toxicity suggests that the agent may be suitable for repeated administration.62 However, comparative studies of this agent with other therapeutic radionuclides are needed before its clinical role can be defined clearly.
Most recently the bone-seeking, α particle–emitting radiopharmaceutical Alpharadin 223RaCl2 (radium chloride) has been developed. The high-energy α particles provide a high dose of radiotherapy to cells within 1 µm of the bone surface with minimal systemic effects. In addition to effective palliation of bone pain, declines in PSA levels in a population of patients with advanced prostate cancer were seen in a small phase 2 study.63 Recently, a phase 3 study in persons with advanced prostate cancer that evaluated the addition of Alpharadin to best supportive care in persons with advanced CRPC was reported. In addition to beneficial effects on quality of life and the incidence of skeletal morbidity, there was a 3.6-month significant improvement in overall survival (OS).64
Bisphosphonates
In the past two decades the bisphosphonates have become established as a valuable additional approach to the range of current treatments. All bisphosphonates are pyrophosphate analogs, characterized by a P-C-P–containing central structure rather than the P-O-P of pyrophosphate and a variable R′ chain that determines the relative potency, adverse effects, and precise mechanism of action.65 The P-C-P backbone renders bisphosphonates resistant to phosphatase activity and promotes their binding to the mineralized bone matrix. The structures of the commonly used bisphosphonates are illustrated in Figure 51-14.
After administration, bisphosphonates bind avidly to exposed bone mineral around resorbing osteoclasts, leading to high local concentrations of bisphosphonate in the resorption lacunae (up to 1000 mM). During bone resorption, bisphosphonates are internalized by the osteoclast, where they cause disruption of several biochemical processes involved in osteoclast function, ultimately leading to apoptotic cell death. These processes include destruction of the cytoskeleton, disruption of the sealing zone at the bone surface, and loss of the ruffled border across which the hydrolytic enzymes and protons necessary for bone dissolution are normally secreted. The molecular mechanisms of action of the bisphosphonates are now established, with nitrogen-containing bisphosphonates shown to inhibit enzymes of the mevalonate pathway that are responsible for events that lead to the posttranslational modification of a number of proteins, including the small guanosine triphosphatases such as Ras and Rho.65 Bisphosphonates that do not contain nitrogen, such as clodronate, induce osteoclast apoptosis through the generation of cytotoxic adenosine triphosphate analogs. Recent studies suggest that bisphosphonates could have direct apoptotic effects on tumor cells and that this effect may be enhanced by combination with other anticancer agents.66
After intravenous administration of a bisphosphonate, the kidney excretes approximately 25% to 40% of the injected dose, and the remainder is taken up by bone. All bisphosphonates have poor bioavailability when they are taken by mouth, and they must be taken on an empty stomach to minimize binding to calcium in the diet.67
Irrespective of the mechanism(s) of action, bisphosphonates have been used successfully in the treatment of a wide range of bone conditions characterized by increased osteoclast-mediated bone resorption. In oncology they have become the standard treatment for tumor-induced hypercalcemia and part of routine medical therapy to prevent morbidity from bone metastases, and they may have a role in prevention of metastasis in the adjuvant setting.68
Bisphosphonates to Prevent Skeletal Morbidity and Relief of Bone Pain
Although radiotherapy is the treatment of choice for localized bone pain, many patients have widespread, poorly localized bone pain, whereas others experience recurrence of bone pain in previously irradiated sites. The bisphosphonates provide an additional treatment approach for the relief of bone pain across a range of tumor types, and the effects seems to be independent of the nature of the underlying tumor or radiographic appearance of the metastases, with sclerotic lesions responding similarly to lytic metastases.69
Additionally, based on the results of large randomized controlled trials conducted in the late 1990s, the bisphosphonates became the standard of care for the treatment and prevention of skeletal complications associated with bone metastases in patients with breast cancer70,71 and multiple myeloma.72 More recently, they have demonstrated benefits in patients with bone metastases resulting from other cancers including prostate cancer,73 lung cancer,74 and other solid tumors (Table 51-4).74,75
Table 51-4
Effects of Bisphosphonates on Skeletal Morbidity: Results of Randomized Trials
Agent and Route | N | Results | Investigators |
BREAST CANCER | |||
Clodronate 1600 mg PO vs. placebo | 173 | Reduced SMR | Paterson et al.77 |
305 vs. 219 events/100 woman yrs (P < .001) | |||
Pamidronate 45 mg IV vs. control | 295 | Increased time to bone progression | Conte et al.79 |
168 vs. 249 days (P = .02) | |||
Pamidronate 90 mg IV vs. placebo | 382 | Reduced proportion experiencing SRE | Hortobagyi et al.71 |
65% vs. 46% (P < .001) | |||
Delay in first SRE | |||
7.0 vs. 13.1 mo (P = .0005) | |||
Pamidronate 60 mg IV vs. control | 401 | Median time to skeletal progression | Hultborn et al.80 |
9 vs. 14 mo (P < .01) | |||
Pamidronate 90 mg IV vs. placebo | 374 | Reduced proportion experiencing SRE | Theriault et al.72 |
67% vs. 56% (P = .027) | |||
Delay in first SRE | |||
6.9 vs. 10.4 mo (P = .049) | |||
Ibandronate 2/6 mg IV vs. placebo | 467 | Reduced SMR with 6-mg dose, 2 mg ineffective | Body et al.85 |
SMR 2.18 vs. 1.61 (P = .03) | |||
Ibandronate 50 mg PO | 564 | Reduced SMPR compared with placebo (0.95 vs 1.18, P = .004) | Body et al.78 |
Zoledronic acid 4 mg IV vs. placebo | 227 | Reduced risk of SRE compared with placebo (hazard ratio 0.62, 95% CI = 0.48, 0.79; P = .0001) Reduced proportion experiencing SRE 50% vs. 30% (P = .003) |
Kohno et al.82 |
Reduced SMR by 43% (P = 0.016) | |||
MULTIPLE MYELOMA | |||
Clodronate 1600 mg PO vs. placebo | 350 | Improved 2-year progression-free survival | Lahtinen et al.86 |
24% vs. 12% (P < .05) | |||
Pamidronate 90 mg IV vs. placebo | 392 | Reduced proportion experiencing SRE | Berenson et al.73 |
24% vs. 41% (P < .001) | |||
Clodronate 1600 mg PO vs. placebo | 614 | Less skeletal morbidity and pain on progression | McCloskey et al.87 |
Zoledronic acid 4mg IV vs. clodronate 1600 mg PO | 1960 | Zoledronic acid reduced mortality by 16% (95% CI = 4 to 26%) compared with clodronic acid, and extended median OS by 5.5 months (P = .04). Zoledronic acid reduced incidence of SRE more than clodronate (265 [27%] vs. 346 [35%], respectively; hazard ratio 0.74, 95% CI 0.62-0⋅87; P = .0004) |
Morgan et al.89,90 |
MULTIPLE MYELOMA AND BREAST CANCER | |||
Zoledronic acid 4/8 mg IV vs. pamidronate 90 mg | 1648 | Zoledronic acid (4 mg) showed clinical activity equivalent to that of pamidronate 90 mg IV | Rosen et al.83,84 |
In patients with breast cancer, 43% had an SRE with 4 mg zoledronic acid, compared with 45% with pamidronate | |||
In patients with myeloma, 47% had an SRE with zoledronic acid, compared with 49% with pamidronate | |||
PROSTATE CANCER | |||
Clodronate (4 × 520) mg oral vs. placebo | 311 | Reduction in number of SREs vs. placebo not significant (49% vs. 41%, P = NS) | Dearnaley et al.91 |
Pamidronate 90 mg IV vs. placebo | 378 | Number of SREs equal in pamidronate and placebo arms, P = 1.0 | Small et al.92 |
Zoledronic acid 4/8 mg vs. placebo | 643 | Proportion of patients experiencing at least one SRE during the study was 25% lower in the zoledronate arm than in the placebo arm (P = .021) | Saad et al.74,93 |
OTHER TUMOR TYPES | |||
Zoledronic acid 4/8 mg vs. placebo | 773 | Significant delay to time of first skeletal event in zoledronic acid arm compared with placebo (P = .023) | Rosen et al.75 |
Significant reduction in proportion of patients having an event (47% vs. 38%, P = .039) |
Breast Cancer
The greatest experience with bisphosphonates is in the management of bone metastases from breast cancer, and the value of the agents is undisputed.76 The absorption of oral bisphosphonates from the gut is poor, variable, and dramatically inhibited by food intake. To make matters worse, the absorbed fraction of oral bisphosphonates decreases even further when the absolute ingested amount is lower; thus the more potent bisphosphonates are even less well absorbed than etidronate and clodronate. Nevertheless, it has been shown in randomized trials that both oral clodronate and ibandronate have some clinical efficacy (see Table 51-4).77,78
Oral ibandronate is the newest and most potent oral agent and is available in Europe and many other countries outside North America.78 This agent has obvious attractions to both patients and health care providers, but the place of ibandronate cannot be clearly defined until comparative data with other bisphosphonates are available.
Pamidronate was the first intravenous bisphosphonate to be systematically evaluated. In an early randomized study, a 48% increase in the median time to progression in bone in favor of the patient group that received pamidronate (249 vs. 168 days, P = .02) was seen and was associated with a marked improvement in bone pain.79 Similar results were reported in a Scandinavian trial.80
Subsequently, the results of two double-blind, placebo-controlled trials of 90-mg pamidronate infusions every 3 to 4 weeks in addition to cytotoxic or endocrine treatments for patients with breast cancer and lytic bone metastases established bisphosphonate treatment as the standard of care in breast cancer.70,71 These two studies were of similar design, with the exception of the systemic anticancer treatment at study entry. As in all subsequent bisphosphonate trials, the primary end point of these studies was the influence of bone-targeted treatment on the number of patients experiencing SREs, as well as the time to the first SRE and the rate of SREs as determined by either a simple annual rate or more complex multiple event analysis techniques. SREs were defined as:
• Occurrence of pathological long bone and vertebral fractures
• Development of spinal cord compression
• Need for radiation for pain relief or to treat or prevent pathological fractures or spinal cord compression
Zoledronic acid is the most potent bisphosphonate available. A phase 1 study of 30 patients with hypercalcemia indicated that dose levels as low as 0.02 mg/kg (1-2 mg total dose) were effective in achieving normocalcemia.81 After a dose-finding, phase 2 study, 4 mg of zoledronic acid was selected for phase 3 evaluation. A placebo-controlled trial of zoledronic acid was performed in Japan, in which women with bone metastases from breast cancer were randomly assigned to treatment with zoledronic acid (n = 114) or placebo (n = 114) every 4 weeks. After 1 year, the percentage of patients with at least one SRE was significantly reduced by 20% with zoledronic acid (30% vs. 50% for placebo; P = .003). In comparison with placebo, zoledronic acid also significantly delayed the time to first SRE (P = .007) and reduced the overall risk of SREs by 41% (P = .019).82
Zoledronic acid has been compared with pamidronate in a randomized, double-blind, phase 3 noninferiority trial (n = 1130) in which the primary efficacy end point was the proportion of patients experiencing at least one SRE during up to 2 years of the study.83 The overall proportion of patients with an SRE and the skeletal morbidity rate were similar with both treatments. However, in a multiple event analysis, zoledronic acid reduced the risk of developing skeletal complications by an additional 20% compared with pamidronate (P = .025).84 Thus the risk of skeletal complications with zoledronic acid is approximately half the rate experienced in the prebisphosphonate era. All markers of bone resorption or formation decreased from baseline to the end of the study, but at all time points the urinary marker of bone resorption NTX was significantly less in the zoledronic acid group than in the pamidronate group. Median OS was similar at approximately 2 years. The most common adverse events were bone pain, nausea, fever, and fatigue, and as with the other adverse effects, they occurred with similar frequency in each group. The incidence of renal dysfunction among the patients receiving 4 mg of zoledronic acid (given on a 15-minute schedule) was indistinguishable from that for the patients receiving pamidronate.
Ibandronate is licensed in Europe for the treatment of metastatic bone disease. A phase 3 placebo-controlled trial of monthly ibandronate 6-mg infusions in persons with breast cancer has shown a significant reduction in skeletal-related morbidity.85 Additionally, improvements in pain and quality of life were clearly demonstrated at this dose.
Multiple Myeloma
Early studies in persons with myeloma evaluated oral clodronate and showed beneficial effects on disease progression86 and a reduction in skeletal morbidity.87 In the past 15 years, intravenous bisphosphonates have become routine clinical management for most patients with multiple myeloma, following the very clear results demonstrated in a placebo-controlled trial of pamidronate, 90 mg, conducted in 392 patients.72 The proportion of patients developing SRE(s) was significantly lower in the group receiving pamidronate than in the group receiving placebo (24% vs. 41%, P < .001). QOL scores, performance status, pain scores, the incidence of pathological fractures, and the need for radiotherapy were all favorably influenced by pamidronate therapy.
Zoledronic acid has also been evaluated in persons with multiple myeloma; 450 patients with advanced myeloma were included in a randomized trial comparing zoledronic acid with pamidronate. No significant differences between the two agents were identified. The overall risk of an SRE was 7% lower with zoledronic acid, but this difference was not statistically significant.83,84
In multiple myeloma, there is preclinical evidence to suggest that amino-bisphosphonates such as zoledronic acid have anticancer activity including inhibition of angiogenesis, enhancement of antitumor immune responses, and direct or indirect modulation of the proliferation and survival of myeloma cells.88 Bone-targeted treatments may also affect progression of myeloma by blocking the release of cytokines and growth factors from the bone matrix, thereby breaking the vicious cycle of bone destruction and cancer growth. Based on these preclinical theories, a large randomized trial was conducted in the United Kingdom by the Medical Research Council to evaluate the role of bisphosphonates in 1960 newly diagnosed patients with myeloma who were receiving either intensive (i.e., high-dose) chemotherapy with stem cell rescue or nonintensive (i.e., standard dose) chemotherapy regimens.89 Patients were randomly assigned to receive either monthly zoledronic acid or daily oral clodronate. Patients treated with zoledronic acid had a better chance of survival, with an improvement in median OS of 5.5 months compared with patients treated with clodronate (P = .04). Notably, the survival benefit with zoledronic acid, observed within the first 6 months, remained statistically significant after adjustment for SREs90 and thus was consistent with clinically meaningful antimyeloma activity.
Prostate Cancer
Bisphosphonates have been shown to reduce biochemical markers of bone resorption in patients with osteoblastic bone lesions that are associated with advanced prostate cancer. Additionally, several phase 2 studies have assessed bone pain and analgesic use with some benefit in these acute end points.68 However, until recently, randomized, placebo-controlled trials of bisphosphonates had failed to demonstrate a significant reduction in skeletal complications from bone metastases in patients with advanced prostate cancer.
The Medical Research Council performed a phase 3 trial of oral clodronate in 311 men with metastatic bone disease from prostate cancer.91 A slight reduction in the proportion of patients receiving clodronate who experienced an SRE and an improvement in time to progression and increased median survival were observed, but none of these differences was statistically significant. Pamidronate has been studied in a placebo-controlled trial of 236 patients with far-advanced prostate cancer and bone metastases. This trial assessed bone pain as the primary end point and included an assessment of SREs as a secondary end point. In this population, pamidronate did not reduce the incidence of SREs and had only a slight effect on bone pain.92
Despite the disappointing results with other bisphosphonates, zoledronic acid was investigated in patients with CRPC and bone metastases to determine whether the increased potency of this compound would translate into improved clinical benefit. In this study of 643 patients, zoledronic acid, 4 mg every 3 to 4 weeks, was significantly more effective than placebo across all primary and secondary end points.73 The group treated with 4 mg of zoledronic acid experienced significantly fewer SRE(s) (33% vs. 44% with placebo; P = .021), and the time to first skeletal complication increased by more than 4 months (P = .011). Using the Andersen-Gill multiple-event analysis, zoledronic acid reduced the overall risk of skeletal complications by 36% and reduced bone pain at all time points.93 However, despite the favorable effects on skeletal morbidity, there were no significant effects on disease-related end points such as time to progression and survival. Treatment was generally well tolerated, and in particular the risk of renal function deterioration in patients treated with zoledronic acid was found to be similar to that of patients who received a placebo.
Other Tumors
The pathophysiology of bone metastases is broadly similar in all tumor types, and bisphosphonates could thus be expected to be of value in preventing skeletal morbidity across the range of tumors involving the skeleton, especially if metastatic bone disease was a patient’s dominant site of disease. As part of the development program for zoledronic acid, a phase 3 randomized, placebo-controlled trial was performed in patients with bone metastases from a wide range of solid tumors other than breast or prostate cancer.74 Zoledronic acid significantly reduced the proportion of patients with at least one SRE (39% vs. 48%, P = .039) and prolonged the time to the first SRE compared with placebo (314 days vs. 168 days, respectively, P = .021). Overall, zoledronic acid reduced the risk for SRE(s) by about 30% (P = .003) compared with placebo. Patients with renal cancer appeared to gain the greatest benefit.75
Adverse Events
When compared with many other therapies, especially in the cancer setting, the severity of adverse events related to bisphosphonates is generally mild. With the exception of the acute phase response, causing transient fever, myalgia, and bone pain, adverse effects after administration of intravenous bisphosphonates are infrequent, provided the drug is infused at the recommended dose and duration. With oral agents, upper gastrointestinal toxicity (esophagitis and dyspepsia) or diarrhea may occur but only occasionally lead to treatment discontinuation. Thus the benefits of treatment with bisphosphonates within their licensed indications almost always outweigh the risks.94
Long-term treatment with bone-targeted agents is associated with the development of osteonecrosis of the jaw (ONJ), particularly when given on a prolonged monthly schedule. ONJ is a relatively recently recognized condition characterized by painful bone destruction, secondary infection, and delayed healing in the mandible and/or maxilla.95 The risk of ONJ appears to be related to duration of treatment, occurring at around 1% per year on therapy.94 Comprehensive guidelines now exist for prevention, diagnosis, and management of the disease,96 including good dental hygiene and pretreatment restorative treatment to reduce the risk of this unpleasant complication of treatment.
RANKL Inhibition to Prevent Skeletal Morbidity
RANKL is a member of the TNF superfamily and is the ligand for OPG. RANKL is expressed on the surface of osteoblasts and released into the bone microenvironment, where it binds to and activates its receptor, RANK, on immature osteoclasts. Mice deficient in either RANKL or RANK lack osteoclasts and exhibit severe osteopetrosis. OPG is also expressed on osteoblasts, and this protein inhibits osteoclast formation and is the key regulator of bone mass. Overexpression of OPG in transgenic mice leads to a profound reduction in the number of mature osteoclasts and markedly increases bone density, whereas OPG-knockout mice have increased bone resorption and severe osteoporosis.97
Denosumab is a fully human, synthetic antibody that binds to RANKL with high affinity, preventing its interaction with RANK in a way similar to that of osteoprotegerin. In early clinical development, a single subcutaneous dose of denosumab caused rapid suppression of bone turnover in patients with multiple myeloma and breast cancer. The maximal amount of urinary NTX suppression (about 70%) was reached after 7 days and was sustained over the 84 days of the study.98 In a dose-finding study in patients with breast cancer and bone metastases,98 the onset of inhibition of bone resorption was rapid, and after 13 weeks of treatment, the median reduction in urinary NTX levels was 71% for all patients treated with denosumab versus 79% for those treated with bisphosphonates.99 Denosumab treatment at a dose of 120 to 180 mg every 4 weeks provided the most reliable and consistent suppression of urinary NTX. Transient hypocalcemia occurred in 8% of patients treated with denosumab, most commonly in those treated with 180 mg of the drug every 4 weeks. As a result, treatment with 120 mg of denosumab every 4 weeks was selected to provide the optimal balance of efficacy and tolerability.
The first evidence suggesting that denosumab might be superior to a bisphosphonate for prevention of skeletal morbidity emerged from a randomized, phase 2 study conducted in patients with bone metastases and urinary NTX levels >50 nmol/mmol creatinine, despite treatment with an intravenous bisphosphonate.100 The patients either continued to receive intravenous bisphosphonate therapy (pamidronate or zoledronic acid) every 4 weeks or were switched to subcutaneous denosumab treatment (180 mg) every 4 weeks (six doses) or every 12 weeks (two doses) for 25 weeks. Normalization of urinary NTX levels (that is, to <50 nmol/mmol creatinine) was achieved in 49 of 69 patients treated with denosumab (71%) and in only 10 of 35 patients treated with a bisphosphonate (29%; P < .001). Moreover, considerably fewer patients treated with denosumab experienced a first SRE during the 25 weeks of the study: 8% (6 of 73 patients) versus 17% (6 of 35 patients, odds ratio 0.31, 95% confidence interval [CI] 0.08-1.18).
Subsequently, three identical double-blind, phase 3, registration studies of denosumab were performed.103–103 These studies included a total of 5723 bisphosphonate-naive patients with bone metastases resulting from breast cancer (n = 2046),101 CRPC (n = 1901),102 and other advanced solid tumors, including non–small-cell lung cancer (n = 702), myeloma (n = 180), and other types of solid tumors (n = 894).103 The patients were randomly assigned to receive four weekly subcutaneous injections of denosumab (120 mg) or intravenous zoledronic acid (4 mg), with supplements of calcium and vitamin D (Table 51-5). The primary end point was the time to first SRE.
Table 51-5
Randomized Clinical Trials Assessing Impact of Bisphosphonates on Disease-Free Survival
Trial | DFS-ITT Analysis | DFS—“Postmenopausal” Subset | P Value |
ITT ANALYSIS | |||
AZURE (N = 3360)116 | 0.98 (0.85,1.12) | .79 | |
ABCSG XII (N = 1803)114 | 0.71 (0.55,0.92) | .013 | |
ZO-FAST (N = 1065)115 | 0.66 (0.44,0.97) | .04 | |
NSABP-B34 (N = 3323)117 | 0.91 (0.79,1.07) | .27 | |
CLODROPLAC* (N = 1047)108 | 0.77 (0.59,1.00) | .048 | |
GAIN (N = 2994)118 | 0.95 (0.77,1.16) | .59 | |
POSTMENOPAUSAL SUBGROUP | |||
AZURE† (N = 1041) | 0.75 (0.59,0.96) | .02 | |
ABCSG XII‡ (N = 1390) | 0.66 (0.48,0.92) | .013 | |
ZO-FAST§ (N = 1065) | 0.66 (0.44,0.97) | .04 | |
NSABP-B34¶ (N = 2139) | 0.68 (0.5,0.92) | .013 | |
CLODROPLAC*§ (N = 539) | 0.66 (0.49,0.93) | .007 | |
GAIN¶ (N = 1557) | 0.75 (0.49,1.14) | .17 |
In patients with bone metastases resulting from breast cancer, denosumab was statistically superior to zoledronic acid in delaying the first SRE (hazard ratio [HR] 0.82, 95% CI 0.71-0.95, P = .01): the median time to a first SRE was 26.4 months for patients treated with zoledronic acid, whereas the median time to first SRE was not reached during the study in subjects treated with denosumab.101 Similar results were reported in patients with bone metastases resulting from prostate cancer: the median time to a first SRE was 17.1 months for patients treated with zoledronic acid versus 20.7 months for those treated with denosumab (HR 0.82, 95% CI 0.71-0.95, P = .008).102 In patients with bone metastases resulting from advanced solid tumors and myeloma, denosumab was not inferior to zoledronic acid (HR 0.84, 95% CI 0.71-0.98, P = .03) but failed to demonstrate statistical superiority over bisphosphonates.103 Denosumab treatment delayed the occurrence of all types of SREs. However, no differences in OS or investigator-reported disease progression were found between the two treatment groups in any of the studies.
A key difference between the safety profiles of denosumab and zoledronic acid is the lack of an effect of denosumab on renal function, which obviates the need for assessment of the patient’s renal function before each denosumab dose. Acute-phase reactions were less common but hypocalcemia was more frequent in patients treated with denosumab. Most episodes of hypocalcemia were generally mild and easily managed, but. all patients should be encouraged to take calcium and vitamin D supplements, and monitoring of serum calcium levels is advised, particularly in patients with impaired renal function. As with intravenous bisphosphonates, the most important adverse event associated with the use of denosumab in oncology settings is ONJ. A similar proportion of patients treated with zoledronic acid and denosumab experienced ONJ (1.3% vs. 1.8%, respectively); in total, 89 confirmed cases of ONJ occurred among 5677 patients who received one or more doses of either agent.104
Optimum Use of Bone-Targeted Agents in Persons with Metastatic Bone Disease
Consensus guidance recommendations indicate that all patients with radiologically confirmed bone metastases from breast cancer should start taking a bisphosphonate or denosumab at the time of diagnosis of bone metastasis and continue with this treatment indefinitely.49,50 The development of an SRE is not necessarily a sign of treatment failure or a signal to stop treatment; evidence is now available to confirm that bone-targeted agents delay second and subsequent complications, not just the first event. However, for persons with multiple myeloma, only bisphosphonate treatment is recommended, because denosumab is not approved for use in persons with multiple myeloma, pending the results from specific ongoing trials.
Despite the obvious clinical benefits of bone-targeted treatments, it is clear that only a proportion of events is prevented, and some patients do not experience a skeletal event despite the presence of metastatic bone disease. It is currently impossible to predict accurately whether an individual patient needs or will benefit from bone-targeted treatments, although elevated bone marker levels, a previous SRE, increasing numbers of lesions, and lytic rather than sclerotic appearance of lesions on radiograms are associated with a higher risk for SREs.41 Overall, the frequency of skeletal events is reduced by 30% to 50%. Bisphosphonates or denosumab are relatively costly additional interventions in cancer care that are now potentially applicable to a very large proportion of patients with advanced malignancy, and the cost-effectiveness of routine long-term treatment has been questioned. Evidence-based guidance on optimum duration, schedule, and dose is becoming essential. Uncertainty remains about the appropriate duration and schedule of treatment. Bone marker–directed therapy is under evaluation in randomized clinical trials and may help personalize treatment according to the rate of bone turnover, and the value of maintenance therapy after 1 to 2 years of treatment may also become clearer when results are available from the ongoing clinical trials.
New Targeted Therapies in the Treatment of Metastatic Bone Disease
As our understanding of the signaling mechanisms between bone cells and tumor cells increases, several new targeted agents have entered clinical development. These agents include cathepsin K inhibitors (an osteoclast-derived enzyme that is essential for the resorption of bone), an antibody to PTHrP, Src kinase inhibitors (a key molecule in osteoclastogenesis), and various anabolic agents including inhibitors of DKK1 that could have a valuable role in increasing bone mass and could encourage healing of lytic lesions.105 The role of these agents in the prevention and treatment of bone metastases should emerge during the next 5 years.
Protecting the Skeleton
Prevention of Bone Metastases
In addition to the well-recognized release of bone cell–activating factors from the tumor, it is now appreciated that release of bone-derived growth factors and cytokines from resorbing bone can both attract cancer cells to the bone surface and facilitate their growth and proliferation.106 Inhibition of bone resorption could therefore have an effect on the development and progression of metastatic bone disease and is an adjuvant therapeutic strategy of potential importance.
Encouraging animal studies with a variety of tumor models and a range of bisphosphonates have shown inhibition of bone metastasis development and a reduction in tumor burden within bone.107 More recently, several clinical trials have been reported suggesting treatment benefits, at least in postmenopausal women.
The early adjuvant studies evaluated oral clodronate: 1079 women with primary operable breast cancer were randomly assigned to receive either clodronate, 1600 mg daily, or placebo for 2 years in addition to standard adjuvant systemic treatment. With a median follow-up time of 5 years, a modest reduction in the frequency of bone metastases in the patients treated with clodronate (63 patients [12%] vs. 80 patients [15%]) was seen (HR for bone metastasis–free survival = 0.692, P = 0.043).108 No significant effect on non–bone recurrence was observed (112 patients [21%] vs. 128 patients [24%]), but patients randomly assigned to the clodronate arm had a higher probability of survival (5-year survival of 82% vs. 77%, P = .047).
A second study included 302 patients with early breast cancer who were selected on the basis of immunocytochemical detection of tumor cells in the bone marrow. The incidence of osseous metastases was significantly lower in the clodronate group, and a large reduction in the incidence of visceral metastases also occurred in the clodronate group.109 Survival was significantly extended. These results have subsequently been updated and show similar results, although the striking effect on extraskeletal visceral relapse was no longer statistically significant.110 A third study produced conflicting results; the overall 5-year survival was significantly lower in the clodronate group (70% vs. 83%, P = .009), although prognostic imbalances favoring the control group may explain this result.111
In the Austrian Breast and Colorectal Cancer Study Group (ABCSG)-12 trial, striking anticancer effects with zoledronic acid were seen in premenopausal women with ER+ breast cancer who were undergoing ovarian suppression therapy and endocrine treatments. Patients who received zoledronic acid had fewer recurrences at all sites, including visceral metastases and locoregional recurrence, compared with those who did not receive zoledronic acid.112,113 Moreover, after longer term follow-up (median of 84 months), a persistent 29% benefit in disease-free survival (DFS) (P = .011) more than 3 years after completion of treatment was seen, suggesting a sustained, long-term “carryover” benefit from adding zoledronic acid to endocrine therapy. Zoledronic acid also significantly improved OS in this study by 39% (P = .033).114
The Zometa-Femara Adjuvant Synergy Trials (ZO-FAST/Z-FAST/EZO-FAST) were initiated primarily to investigate the efficacy of zoledronic acid in preventing bone loss during adjuvant therapy with aromatase inhibitors. In ZO-FAST, at 60 months follow-up, in addition to the beneficial effects on bone mineral density (BMD) seen with immediate zoledronic acid, this group had a significant 34% reduction in the risk of DFS events compared with the delayed zoledronic acid group (P = .0375).115 Similar to the findings in the ABCSG-12 trial, zoledronic acid initiation at the start of adjuvant endocrine therapy was associated with a reduction in breast cancer recurrence both in and outside bone. However, significant benefits were not observed in the companion Z-FAST and EZO-FAST studies.
In a large randomized adjuvant trial (AZURE) that recruited 3360 patients across a wide age range and with ER+ and ER− tumors, zoledronic acid did not significantly improve DFS compared with standard therapy alone in the overall population (HR = 0.98; P = .79).116 However, prespecified analyses evaluating treatment benefits in patient subgroups revealed a significant difference in zoledronic acid effects on disease outcome based on the menopausal status of the patients at study entry. In patients who were postmenopausal for at least 5 years before study entry, zoledronic acid significantly reduced the risk of DFS events by 25% (P = .02) and the risk of death by 26% (P = .04). Most interestingly, although the effects of zoledronic acid on distant skeletal recurrence did not differ significantly by menopausal groups (heterogeneity test χ21 = 0.14, P = .70), for the other components of invasive DFS, there was a significant difference in treatment effect according to menopausal status, with an apparent benefit in postmenopausal women and potential harm in all other women (heterogeneity test χ21 = 14.00, P = .001). Although the underlying mechanisms for the benefit seen in an estrogen-depleted patient subset have yet to be entirely elucidated, they are consistent with the benefits seen in the ABCSG-12 trial in which all patients underwent a treatment-induced menopause with goserelin.114
Data with other bone-targeted agents are limited, but preliminary results from randomized trials evaluating oral clodronate (NSABP-B34—NCT00009945)117 and oral ibandronate (GAIN—NCT00196872)118 have been presented. In NSABP-B34, with a median follow-up of 8.4 years, oral clodronate had no significant effect on DFS or OS in the total study population. However, similar to the findings in AZURE, a significant 38% reduction in distant metastasis was seen in patients older than 50 years (a surrogate for postmenopausal status) treated with clodronate (P = .003).117 Again the benefit was greatest in preventing recurrence at extraskeletal sites. In the GAIN trial, median follow-up is short at 31 months, and no significant differences in DFS (P = .80) between the groups treated with ibandronate and placebo were seen. A trend in favor of ibandronate in postmenopausal women or those older than 60 years was observed, but insufficient events have occurred for reliable subgroup analyses.118 The results of the main studies are summarized in Table 51-6. A recent metaanalysis of trial results in postmenopausal women suggested a clinically and statistically significant 18% improvement in DFS (P < .001).119
Table 51-6
Key Trials In The Clinical Development Of Denosumab
Indication | No. of Patients | Design | Dose (All SC) | Key Outcomes | Reference |
Breast cancer—bone metastases | 255 | Phase 2: randomized, active control (IV bisphosphonate) | 30-180 mg every 4 wk or 60-180 mg every 12 wk | At week 13, reduction in uNTX of 71% (denosumab) vs. 79% (IV bisphosphonate); no SAEs; SREs experienced during the study by 9% of patients treated with denosumab vs. 16% of patients treated with bisphosphonate | 99 |
Prevention of bone loss in patients with breast cancer taking AIs | 252 | Phase 3: double-blind, randomized to denosumab or placebo | 60 mg every 6 mo × 4 | Lumbar spine BMD increased by 5.5% and 7.6% vs. placebo at 12, 24 mo respectively (P < .001); sCTX reduced by 91% in denosumab group vs. 9% in placebo (P < .0001); P1NP reduced by 29% vs. 2% for placebo (P < 0.001). | 127 |
Prevention of bone loss in patients with prostate cancer taking ADT | 1468 | Phase 3: double-blind, randomized to denosumab or placebo | 60 mg every 6 mo × 4 | At 24 mo, lumbar spine BMD increased by 5.6% in denosumab group vs. placebo (P < .001); vertebral fracture incidence at 36 mo: 1.5% vs. 3.9% in placebo group | 132 |
Prevention of metastases in prostate cancer | 1432 | Phase 3: double-blind, randomized to denosumab or placebo | 120 mg every 4 wk | Denosumab increased bone metastasis-free survival by 4.2 mo over placebo (HR 0.85, 95% CI 0.73-0.98, P = .028); ONJ cumulative incidence of 4% at 3 yr | 119 |
Various tumor types | 104 | Phase 2 with uNX >50 nmol/mmol creatinine, receiving bisphosphonates randomized, to continue or switch to denosumab | 180 mg every 4 wk | 71% patients receiving denosumab normalized vs. 29% in bisphosphonate arm (P < .001); % patients experiencing first SRE during the study was 8% over 25 wk | 100 |
Breast cancer | 2026 | Phase 3 double-blind, double-dummy, randomized to denosumab or zoledronic acid | 120 mg every 4 wk | Denosumab was superior to zoledronic acid in delaying time to first SRE (HR 0.82, 95% CI 0.71-0.95, P = .01); bone resorption marker suppression significantly greater with denosumab | 101 |
Castration-resistant prostate cancer | 1904 | Phase 3: double-blind, double-dummy, randomized to denosumab or zoledronic acid | 120 mg every 4 wk | Denosumab was superior to zoledronic acid (by 18%) in delaying time to first SRE, 17.1 mo zoledronic acid; 20.7 mo denosumab (HR 0.82, 95% CI 0.71-0.95, P = .01) | 102 |
Advanced cancer (excluding breast and prostate) but including zoledronic acid | 1786 | Phase 3: double-blind, double-dummy, randomized to denosumab or zoledronic acid | 120 mg every 4 wk | Denosumab was superior to zoledronic acid in delaying time to first SRE and superior in delaying first and subsequent SREs (HR 0.82; P = .001) | 103 |
Prostate cancer spreads almost exclusively to bone and provides the ideal clinical setting for the evaluation of bone-targeted treatment to modify the course of the disease. Smith and colleagues119 studied 1432 men with nonmetastatic CRPC who were at high risk for bone metastasis by virtue of either a PSA of ≥8.0 ng/mL and/or PSA doubling time ≤10.0 months. Patients continued with ADT and were randomized to receive monthly denosumab, 120 mg, or placebo. Denosumab significantly increased bone metastasis-free survival by a median of 4.2 months compared with placebo (HR 0.85, 95% CI 0.73-0.98, P = .028) and delayed time to symptomatic first bone metastases. However, this effect on the disease process has not translated into an improvement in overall survival and, considering the relatively high cumulative incidence of ONJ (4% at 3 years), it is unlikely the efficacy will be considered sufficient to change clinical practice.120
Effects of Cancer Treatments on Skeletal Health
There are now increasing numbers of long-term survivors from cancer who have received combination chemotherapy, radiotherapy, and hormonal cancer treatments. Many of these persons are at increased risk of osteoporosis, largely because of the endocrine changes induced by treatment. Clinically relevant, direct effects of cytotoxic drugs on bone also might exist. Cancer treatment–induced bone loss is a particularly important long-term problem for women with breast cancer and men receiving ADT.121 Peak bone mass minus the bone loss associated with age and estrogen deficiency are the main determinants of osteoporotic fracture risk. However, other factors, including genetics, lifestyle, concomitant medication, and nutrition, influence the risk for bone loss.
Bone Loss in Breast Cancer
Cancer treatment–induced bone loss is an increasingly recognized complication in women receiving long-term estrogen-reducing therapies. Estrogen is critical in the maintenance of normal bone mass in women. After menopause a reduction in BMD occurs, with the loss most pronounced during the first 3 years, when the rate averages 2% to 5% annually, before reducing to a rate of about 0.5% annually thereafter. In the adjuvant setting, all third-generation aromatase inhibitors have demonstrated increased loss of BMD, which may lead to osteoporosis and skeletal complications.121 Compared with naturally occurring bone loss associated with ageing, aromatase inhibitor–associated bone loss may result in greatly increased BMD loss at 1 year, with rates averaging 5% to 6% in the immediate postmenopausal period.121
Several clinical trials have demonstrated increased fracture rates in postmenopausal women with breast cancer who received aromatase inhibitors.122 However, a significant proportion of this excess rate of fractures may be due to the absence of the bone-protective effects of tamoxifen. Tamoxifen is known to have a modest estrogenic effect on bone, at least in postmenopausal women, and placebo-controlled trials, for instance, in the breast cancer prevention setting, have shown a modest reduction in fracture incidence compared with placebo.121
Intravenous bisphosphonate administration is used widely in oncology to treat metastatic disease and could be an attractive option for preventing bone loss in a cancer population. Among premenopausal patients with breast cancer, 6 monthly treatments with zoledronic acid has also been shown to reverse the bone loss induced by a combination of the LHRH analog goserelin plus further estrogen suppression with anastrozole. In this study, the mean loss of bone in the lumbar spine over 3 years in the absence of a bisphosphonate was 17%. However, the bone loss was abrogated by administration of 6 monthly treatments with zoledronic acid.122
In postmenopausal women, the strategy of immediate treatment with zoledronic acid for 6 months alongside aromatase inhibitor therapy has been compared with initiation of bone protection treatment if osteoporosis or significant bone loss occurs upon treatment.115,123 In the Z-FAST study, the differences in lumbar spine and hip BMD at 5 years between patients receiving immediate or delayed treatment were approximately 9% and 6%.123 However, despite this impressive effect on BMD, no significant difference in fracture rates have yet to be demonstrated.
Other bisphosphonates, including oral daily clodronate (1600 mg),124 weekly risedronate (35 mg),125 and oral monthly ibandronate (150 mg),126 as well as monthly subcutaneous denosumab (60 mg for 6 months),127 have all been shown to have significant beneficial effects on BMD. The choice of agents in established postmenopausal patients is broad. However, for the rapid bone loss associated with chemotherapy-induced menopause or ovarian suppression, the more potent agents such as zoledronic acid or denosumab appear to be necessary.128
Emerging guidance suggests that overall fracture risk in women with breast cancer should be determined using clinical risk factors and BMD when available. A panel of experts from the United Kingdom developed two treatment algorithms, one for premenopausal patients and one for postmenopausal patients.129 Although both algorithms use fracture risk factors as part of the criteria for categorizing patients into low-, medium-, and high-risk groups, BMD is still an integral part of the equation. Hadji et al.121 recommend treating patients receiving aromatase inhibitors who have ≥two identified risk factors (age >65 years, body mass index <20 kg/m2, family history of hip fracture, personal history of fragility fracture after age 50 years, corticosteroid use by mouth >6 months, and smoking). The concept of using validated fracture risk factors with or without BMD to assess overall fracture risk in women with breast cancer is consistent with the FRAX algorithm developed for healthy women. Similar guidance is under development for men with prostate cancer who are undergoing ADT.
Bone Loss in Prostate Cancer
Many men with prostate cancer are at risk of the development of osteoporosis, largely because of the ADT they receive for their cancer.130 These treatments are being introduced earlier and earlier in the course of the disease, and as a result, men may experience many years of androgen suppression. ADT results in substantially reduced serum concentrations of testosterone (to less than 5% of normal level) and estrogen (to less than 20% of normal level) with consequent adverse effects on bone turnover.
ADT leads to accelerated bone loss and an increase in fracture rate, as evidenced by large retrospective epidemiological studies.130 As the potential scale of the problem is being realized, attention is focusing on measuring bone density in patients starting ADT to assess those at risk and on therapeutic options such as bisphosphonates to prevent or treat therapy-related bone loss in persons with prostate cancer. Alendronate, risedronate, pamidronate, and zoledronic acid have all been shown to prevent loss in BMD in patients with locally advanced prostate cancer.131 In one study of zoledronic acid, BMD increased by 5.3% at the lumbar spine and 1.1% in the hip in men treated with zoledronic acid but declined by 2% in the lumbar spine and 2.8% in the hip with placebo.132
Denosumab is the only agent to have a specific license for treatment-induced bone loss associated with ADT. In a placebo-controlled trial of denosumab in 1468 men receiving ADT for nonmetastatic prostate cancer,133 36 months of denosumab treatment was associated with a significantly reduced incidence of new vertebral fractures (1.5% with denosumab vs. 3.9% with placebo; relative risk [RR] 0.38; 95% CI 0.19-0.78). The incidence of fracture at any site was also reduced in the denosumab group, although this difference was not significant: 38 fractures (5.2%) with denosumab, versus 53 (7.2%) with placebo (RR 0.72, 95% CI 0.48-1.07). BMD increased from baseline at all sites in the denosumab group, whereas it declined in the placebo group, leading to substantial absolute differences in BMD between the two groups (6.7% at the lumbar spine and 4.8% at the total hip) after 36 months of study.
Complications of Bone Metastases
Bone metastases cause considerable morbidity: pain, impaired mobility, hypercalcemia, pathological fracture, spinal cord or nerve root compression, and bone marrow infiltration. In two large randomized trials that included patients with breast cancer and multiple myeloma receiving chemotherapy, the mean skeletal morbidity rates (number of skeletal events per year) in the absence of bisphosphonates were 3.5 and 2.0, respectively, indicating that a skeletal event occurs in persons with metastatic bone disease from breast cancer on the average of every 3 to 4 months and in persons with multiple myeloma every 6 months.70,72 However, despite the clinical importance of metastatic bone disease and the huge expenditure on medical care for skeletal complications, until recently relatively little thought has been given as to how best to coordinate clinical management and deliver optimum care for patients with bone metastases.
Bone Pain
Spinal instability is the cause of back pain in 10% of patients with cancer.134 This instability can cause excruciating pain that is mechanical in origin. The patient is comfortable only when lying still, and any movement reproduces severe pain. Consequently, the patient might not be able to sit, stand, or walk. Because the pain is mechanical in origin, radiation therapy or systemic treatment cannot help; the only solution is stabilization of the spine. Stabilization requires major surgery, with risks of significant morbidity and mortality, but with careful selection of patients, excellent results can be obtained.
Hypercalcemia of Malignancy
Hypercalcemia (see Chapter 37) is another emergency associated with metastatic bone disease. Its clinical features include nausea, vomiting, dehydration, and confusion. Although malignant hypercalcemia is usually associated with demonstrable bone metastases, this is not always the case. Hypercalcemia causes a number of signs and symptoms that vary considerably from patient to patient. These signs and symptoms are often nonspecific, affecting many systems in the body, and can be mistaken for symptoms of the underlying cancer or associated treatment if there is not an astute awareness of the possibility of hypercalcemia. If untreated, a progressive rise in serum calcium leads to deterioration in renal function and level of consciousness. Death ultimately ensues as a result of cardiac arrhythmias and renal failure.
Intravenous bisphosphonates, in conjunction with rehydration, are now established as the treatment of choice for hypercalcemia. Approximately 70% to 90% of patients will achieve normocalcemia, resulting in relief of symptoms and improved QOL. Zoledronic acid is the most effective bisphosphonate for the acute treatment of this metabolic emergency.135
Pathological Fractures
The incidence of pathological fracture in patients with bone metastases is somewhat uncertain and is dependent on whether rib and vertebral fractures are included and the method of evaluation. In recent series in which regular skeletal surveys were performed, the annual risk of a pathological fracture (in the absence of bisphosphonates) was between 20% in persons with prostate cancer73 and 40% in persons with breast cancer.70 Not all of these fractures are symptomatic, and undoubtedly some are due to treatment-induced osteoporosis rather than metastatic infiltration. Nevertheless, structural damage to bone is extremely common in metastatic bone disease.
The probability of developing a pathological fracture increases with the duration of metastatic involvement and is therefore, somewhat paradoxically, more common in patients with bone-only disease who have a relatively good prognosis.136
Radiologic assessment also yields information about the size of a lesion and the extent to which the bone is destroyed. When less than two thirds of the diameter of a long bone is affected, pathological fracture is relatively unusual, but above this limit, the fracture rate increases markedly, with an incidence of approximately 80% for such lesions. A practical scoring system incorporating anatomic, radiographic, and symptom-related factors has been described to give valuable guidance in the selection of patients for prophylactic fixation.137
Spinal Instability
Spinal instability can cause excruciating pain that is mechanical in origin and not relieved by radiotherapy or systemic treatment.134 As with pathological fractures of long bones, stabilization is required for pain relief and involves major surgery. Several methods can be used to achieve spinal stabilization, but in general, the posterior approach is technically easier and allows stabilization of a larger area of the spine. With careful selection of patients, excellent results can be obtained. An associated neurologic deficit is not a contraindication to these procedures.
Percutaneous vertebroplasty and kyphoplasty, a new approach to treating spinal pain and instability, involves injecting an acrylic polymer into a diseased vertebral body. The technique was developed initially for the treatment of painful vertebral hemangiomas, and considerable experience with it has been obtained in the treatment of osteoporotic compression fractures. Its use has now been extended to the treatment of malignant spinal disease.138 The technique provides effective pain relief, which is achieved more rapidly than with radiotherapy, and it confers the added benefit of providing structural support to the spinal column, thus reducing the risk of vertebral collapse and instability. Although generally a safe procedure, vertebroplasty can be complicated by leakage of the polymer, which predisposes to spinal cord or nerve root compression. The risk of this complication is less with kyphoplasty. The technique seems to have the potential for wider use, particularly among patients with limited vertebral disease and those for whom major surgical spinal stabilization procedures are unsuitable.
Compression of the Spinal Cord or Cauda Equina
Back pain is the most common initial symptom of spinal cord compression. Two types of pain can occur: local spinal or radicular. Radicular pain varies with the location of the tumor, being common in the cervical (79%) and lumbosacral (90%) regions and less common with thoracic lesions (55%). Both local spinal and radicular pain are experienced close to the site of the lesion identified at myelography. Motor weakness, sensory loss, and autonomic dysfunction are all common at presentation of spinal cord or cauda equina compression. The development of back pain in a patient with cancer, coincident with an abnormality on a plain spinal radiogram, should serve as a warning for the possible development of spinal cord compression and prompt rapid referral for imaging and assessment by a specialist spinal team.139 Spinal cord compression is covered in detail in Chapter 49.