Cancer of the Lung
Non–Small Cell Lung Cancer and Small Cell Lung Cancer
Leora Horn, Rosana Eisenberg, David Gius, Katherine N. Kimmelshue, Pierre P. Massion, Joe Bill Putnam, Clifford G. Robinson and David P. Carbone
• Constitutes 80% to 85% of new cases of lung cancer in North America.
• Most frequent histologies: adenocarcinoma, squamous cell carcinoma, and large cell carcinoma.
• Leading cause of cancer-related death in the United States for both men and women; represents one of the most preventable forms of cancer death.
• Tobacco use causes 80% of lung cancer deaths.
• Low-dose computed tomography (CT) scan in high-risk individuals is associated with high sensitivity but low specificity for detection of early stage disease.
• Screening remains controversial.
• History and physical examination, routine hematologic and biochemical testing.
CT scan (with contrast) evaluating lungs, mediastinum, liver, and adrenals
Positron emission tomography (PET) scan
If patient has locally advanced or metastatic disease, add magnetic resonance imaging (MRI) of brain
If PET is not available, add bone scan
• Bronchoscopy and/or CT-guided and/or ultrasound-guided biopsy.
• If disease is clinically more advanced, use the least invasive biopsy procedure and sample to confirm advanced disease.
• Mediastinal node evaluation:
If disease is in early stage (I or II) and PET of mediastinum is negative, proceed directly to surgery. If PET is positive, proceed to biopsy via endobronchial ultrasound (EBUS), or endoscopic ultrasound (EUS), or mediastinoscopy, depending on node location.
Role of preoperative or postoperative chemotherapy is unclear. Often recommended in patients with stage IB tumors ≥4 cm.
Stereotactic body radiotherapy in selected cases.
Postoperative chemotherapy prolongs survival in patients with a good performance status.
Stereotactic body radiotherapy in selected cases.
Postoperative chemotherapy useful in resected IIIA in patients with a good performance status.
Role of preoperative chemotherapy ± radiotherapy for stage IIIA is unknown except for Pancoast tumors where it is recommended.
Concurrent chemotherapy combined with radiation therapy in patients with a good performance status.
Concurrent chemotherapy combined with radiation is superior to radiation alone; increased toxicity limits this approach in frail patients.
Clinical trials of new approaches should be a high priority.
Primary chemotherapy with platinum-based doublet improves the quality and quantity of life.
All tumors should be sent for molecular testing.
Targeted therapy with epidermal growth factor receptor tyrosine kinase inhibitors (EGFR TKI) as first-line therapy in patients with known EGFR mutations.
No advantage for triplet therapy.
Non–platinum-based therapy is appropriate in selected patients.
Single-agent therapy reserved for select populations.
There is a clear need for more clinical trials at this stage.
• Second- or third-line therapy
Further treatment with any of several agents is appropriate with progression after chemotherapy if patient sustains a good performance status; docetaxel, pemetrexed, erlotinib, and crizotinib are all U.S. Food and Drug Administration (FDA)-approved.
Local recurrence after surgery can be treated with combined chemotherapy and radiation after complete restaging.
• Accounts for approximately 15% of new cases of lung cancer each year in United States; incidence is decreasing.
• Subtypes include small cell carcinoma and combined small cell carcinoma (most often combined with squamous cell carcinoma, adenocarcinoma or large cell carcinoma).
• History and physical examination, routine histologic and biochemical testing.
CT scan (with contrast) evaluating lungs, mediastinum, liver, and adrenals.
PET useful with clinically early stage disease.
Brain evaluation (MRI preferred).
If there are signs or symptoms of bone involvement, a bone scan or PET scan is recommended.
Mediastinal node evaluation is not required unless as a convenient site for biopsy.
If peripheral smear or hemogram is abnormal, a bone marrow biopsy may be obtained if no other site of metastatic disease has been confirmed.
Concurrent etoposide plus cisplatin and radiation to the intrathoracic disease.
Prophylactic cranial irradiation in complete (or near complete) responders.
Etoposide (or irinotecan) plus cisplatin or carboplatin.
No evidence a third agent or dose intensification improves outcome.
Research studies are a clear priority given the lack of progress in this arena.
Prophylactic cranial irradiation in complete (or near complete) responders.
Consider consolidative radiotherapy (RT) to primary in complete responders.
Second-line therapy useful mainly in patents who have experienced a longer treatment-free interval and a good performance status.
Topotecan FDA-approved; however, any of several chemotherapy agents offer short-term benefit.
Research protocols are a preferred choice for patients with disease recurrence.
Introduction
Although reports of pulmonary malignancies date to antiquity, lung cancer is largely a disease of modern man. Prior to 1900, lung cancers were viewed as “matters of medical curiosity not known to be in any degree influenced by medicine and too rare to be of much practical importance.”1 By the mid-20th century, following the introduction of cheap, mass-produced cigarettes, however, lung cancer had become epidemic and firmly established as the leading cause of cancer-related death in North America and Europe.2 It should not be forgotten that lung cancer is potentially one of the most preventable of all of the major malignancies afflicting man. Its primary cause is tobacco smoke.3 King James I was among the first to chronicle the adverse health effects of tobacco smoke,4 but it was Raymond Pearl’s landmark 1938 report that conclusively established the devastating impact smoking has on longevity.5 It would be another decade before tobacco smoking was firmly established as a causative agent of lung cancer6,7 and nearly 30 more years before the emergence of the U.S. Surgeon General’s initial report of the ill effects of tobacco smoking. Regrettably it would be yet another 3 decades before the tobacco industry publicly acknowledged this obvious truth, but only after a long, drawn out battle of misinformation and deception,8 ironically helped along by the unwitting (perhaps) complicity of physicians.9 With the belated recognition of the etiologic role of tobacco smoke the incidence of lung cancer has started to decline in North America and parts of Europe. For the most part the decline is seen most clearly in men. Only recently has this decline become apparent in women in the United States, following a similar decline among men 15 to 20 years prior.10 In short, the story of lung cancer is replete with controversy,11 politics,12 pessimism,13 and, more recently, guarded optimism.14 This chapter focuses on our perceptions of modern-day management of lung cancer with an emphasis on advances made over the past 5 years.
Epidemiology
Lung cancer was an uncommon disease in the early part of the 20th century but then began an epidemic rise to far surpass all other cancers in numbers and rates of death.15 Lung cancer is the leading cause of cancer-related mortality with an estimated 244,180 new cases and 164,770 deaths anticipated in 2012.16 Lung cancer is relatively rare in persons younger than age 40 years, but rates rise steadily until age 80 years and then taper off; the projected lifetime probability of developing lung cancer is estimated to be approximately 8% among males and approximately 6% among females.17 The incidence of lung cancer varies by racial and ethnic group, with the highest age-adjusted incidence rates among African American men (Fig. 72-1). The excess in age-adjusted rates among African Americans occurs only among men, but examinations of recent age-specific rates show that before age 50 years, mortality from lung cancer is more than 25% higher among African American women compared with white women.17 Incidence and mortality rates among Hispanic, Native, and Asian Americans are only 40% to 50% those of whites.
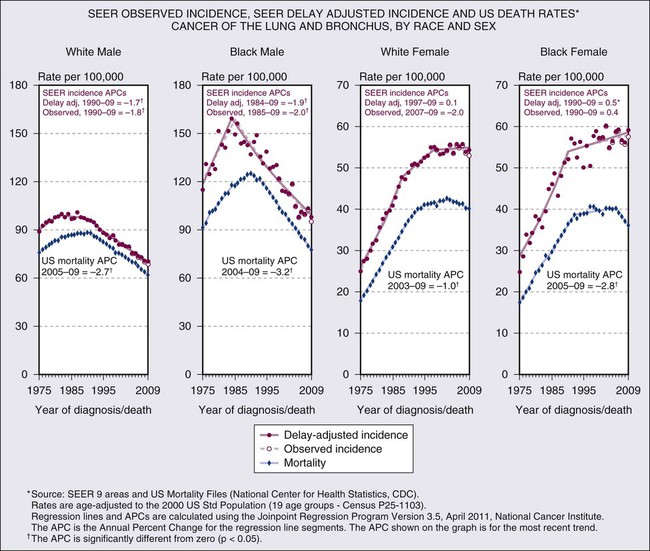
The trends in overall lung cancer rates mask differences in temporal patterns according to lung cancer cell type. Figure 72-2 shows changes in age-adjusted incidence rates for squamous cell carcinoma and for adenocarcinoma during 1973 to 2003 based on data from nine continuing cancer registries in the National Cancer Institute’s Surveillance, Epidemiology, and End Results (SEER) program of cancer registration. Among men, squamous cell cancers predominated in the first two thirds of this period. The decline in incidence of lung cancer among men was first apparent for squamous cell tumors. Decreases began in the early 1980s so that by the mid-1990s rates of squamous cell carcinoma among men had dropped below those for adenocarcinoma, which did not peak until over a decade later. Among women, adenocarcinomas have been more common than squamous cell carcinomas during the past 3 decades. The adenocarcinoma excess among women has become more pronounced over time, as rates of squamous cell cancers increased steadily through the 1980s before beginning to decline, whereas adenocarcinoma did not plateau until about a decade later. Although not shown, rates of small cell carcinoma, the third most frequent cell type, tended to parallel those for squamous cell cancer among both sexes. Trends for other cell types of lung cancer, including large cell carcinomas, tend to be intermediate between those of squamous cell carcinomas and adenocarcinomas. As discussed later in the chapter, although 5-year relative survival rates for lung cancer have improved over time, the survival rates are low, currently approximately 15% overall.17 Some variation exists by sex, race, cell type, and molecular status, with slightly higher survival among whites than blacks and females than males, but for no group does the overall 5-year relative survival exceed 20%.
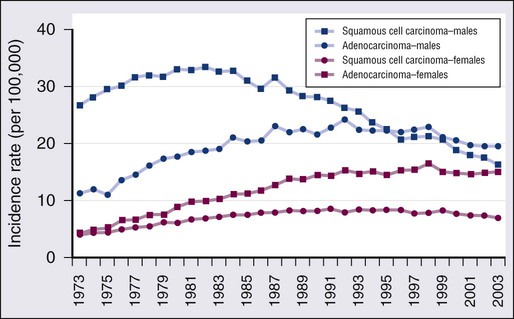
Risk Factors
The cause of the large majority (80%) of lung cancers is cigarette smoking.18,19 There is a massive compilation of scientific evidence from epidemiological studies conducted around the world since the 1950s demonstrating the link between smoking and lung cancer. Epidemiological research has also revealed that several other factors have been implicated as causes of lung cancer, although none to the extent of tobacco.20,21 Cigarette smokers have been shown to have large increases in the risk of lung cancer. A recent deep-sequencing study suggested that one genetic mutation is induced for every 15 cigarettes smoked. Numerous investigations typically show 10 fold or greater increases in risk of this cancer among smokers compared with those who have never smoked.18,19 One of the largest studies is the American Cancer Society’s prospective cohort study of more than 1 million Americans in which a more than 20 fold increase of lung cancer has been observed among men who were current smokers at the start of the followup in the early 1980s.18,19 In contrast, even the most prolonged and intense exposures to asbestos, perhaps the most prominent occupational cause of lung cancer, are associated with no more than about fivefold increase in risk of lung cancer.22
Risks of lung cancer are lower among persons who quit smoking than among those who continue smoking.18,19 The reductions in risk indicate that quitting smoking is beneficial (and conversely that continuing to smoke is harmful). Risk among former smokers on average is less than one-half that of those who continue to smoke. In the American Cancer Society cohort study cited above, former smokers had a ninefold increase in lung cancer compared with men who had never smoked versus the 20 fold excess in those who continued to smoke.18,19 Such relative reductions have been consistently seen, with the size of the reduction in risk increasing the longer the person has quit smoking, although generally even long-term former smokers have higher risks of lung cancer than those who never smoked.18,19
Cigarette smoking has been shown to increase risk of all the major lung cancer cell types.18–21 The magnitude of the increase varies by histologic type, however, with highest risks for squamous cell, small cell, and large cell carcinomas of the lung. Some early studies tended to show only small increases in risk of adenocarcinoma among smokers, but more recent studies indicate that the excess of lung adenocarcinoma is substantial.18,19,21
Cigarette smoke has also been implicated in increasing risk of lung cancer among nonsmokers. A 2006 update of the Surgeon General’s report declared that there is sufficient evidence to list passive smoking as an established cause of lung cancer and called for further control of environmental tobacco smoke (ETS) exposures.23 The risk from ETS is far less than from active smoking, with approximately a 20% to 30% increase in lung cancer observed among nonsmokers married for many years to smokers, in comparison to the 2000% increase among continuing active smokers. Nevertheless, cancer-control activities based on the knowledge that ETS exposure may convey an increased risk of lung cancer have helped reduce exposures in public places and have also provided additional incentive for smokers to quit the habit.
Although cigarette smoking is the dominant cause of lung cancer, several other risk factors for this cancer have been identified.20,21 These include occupational exposures to asbestos and other workplace agents, some of which have been evaluated for nearly as long as cigarette smoking. Among the occupational agents considered as known lung carcinogens are arsenic, bischloromethyl ether, hexavalent chromium, mustard gas, nickel (as found in certain nickel refining processes), and polycyclic aromatic hydrocarbons.20,21,24 Several other occupational exposures have been associated with increased rates of lung cancer, but the causal nature of the association is not clear. Epidemiological studies have attempted to assess the potentially synergistic interrelationship between certain workplace exposures and smoking. Risk of lung cancer among men exposed to asbestos who also smoked was originally thought to be exceptionally high (with early reports of 50 fold or greater excesses compared with unexposed nonsmokers), but recent modeling of larger data pools suggests that asbestos and tobacco combine to enhance lung cancer risk in a less than multiplicative manner.25 Occupational observations have also provided clues to the mechanisms of lung cancer induction. Risk of lung cancer among asbestos-exposed workers, for example, is increased primarily among those with underlying asbestosis, raising the possibility that the scarring and inflammation produced by this fibrotic nonmalignant lung disease may, in many cases (though likely not in all), be the trigger for asbestos-induced lung cancer.26
Increased risks of lung cancer have also been associated with other factors. Diet and nutrition are thought to be involved, as numerous investigations have shown somewhat higher risks of this cancer among those with low fruit and vegetable intake during adulthood.20,21 The early observational studies led to hypotheses that specific nutrients, in particular retinoids and carotenoids, might have chemopreventive effects for lung cancer. Randomized clinical trials were launched, but failed to validate this hypothesis when reports from interventions involving supplementation with beta-carotene in trials both in Finland and the United States found increased rather than decreased incidence of lung cancer among the supplemented.27,28 The current consensus regarding diet and lung cancer remains muddled, with a minor role for nutritional factors likely, but difficult to assess epidemiologically. Ionizing radiation has been established as a lung carcinogen, most convincingly demonstrated from studies showing modestly increased rates of this cancer among persons exposed to the atomic bombs of Hiroshima and Nagasaki and large excesses among workers exposed to α irradiation from radon in underground uranium mining.20,21 Extrapolations from the high exposures in mines to low-level radon exposures in homes, as well as direct observations from case-control studies assessing measured levels in homes, suggest that prolonged radon exposures above the recommended remedial levels might impart a risk of lung cancer equal to or greater than that of ETS.29 Prior lung diseases, such as asbestosis (mentioned above), chronic bronchitis, emphysema, and tuberculosis have also been linked to increased risks of lung cancer. Although smoking itself is a cause of the chronic obstructive pulmonary diseases (COPDs), the link between chronic bronchitis and emphysema and lung cancer persists after adjustment for smoking, with as much as double the smoking-adjusted cancer risk among those with COPD.20,21,30
Familial clustering of lung cancer has been observed, raising the possibility of inherited traits that may increase risk among some individuals, with risk about doubled in families with prior lung cancer.20,21 Individuals with inherited mutations in retinoblastoma and p53 (Li-Fraumeni syndrome) genes may develop lung cancer. Genome-wide association studies have recently identified four genetic loci for lung cancer risk, including two on 5p15 (TERT-CLPM1L), 15q25.1 (CHRNA5-CHRNA-3 nicotinic acetylcholine receptor subunits) and 6p21 (BAT3-MSH5).33–33 A rare germline mutation involving the epidermal growth factor receptor (EGFR) within exon 20 that results in an amino acid substitution at position 790 from threonine to methionine (T790M) has been linked to lung cancer susceptibility in never smokers.34,35 Smoking also clusters within families, so some of the familial aggregation of lung cancer may be smoking related. Nevertheless, there appear to be multiple genetic factors that help determine the way in which individuals metabolize, detoxify, repair, or otherwise respond to lung carcinogens, including the carcinogens in cigarette smoke, as discussed in more detail later in this chapter.
Smoking Cessation
Given the undeniable link between cigarette smoking and lung cancer,3 it is incumbent upon physicians to promote tobacco abstinence and help their patients who smoke to stop smoking.36 Smoking cessation, even well into middle age, can minimize an individual’s subsequent risk of lung cancer, and stopping before middle age avoids more than 90% of the risk attributable to tobacco.37,38 By contrast, there is little health benefit realized by simply “cutting back.”39 Among victims of lung cancer smoking cessation is associated with improved survival,40–43 fewer side effects from therapy,44 and an overall improvement in quality of life.45 Often forgotten is the fact that smoking alters the metabolism of many chemotherapy drugs, potentially adversely altering the toxicities and therapeutic benefits of the agents.46 Therefore, it is important to promote smoking cessation even after the diagnosis of lung cancer is established.47,48 Although smoking cessation is extremely difficult, patients with lung cancer tend to be highly motivated and success rates mirror that of other disease states.49 However, the individual must want to stop smoking and must be willing to work hard to achieve the goal of smoking abstinence. Nicotine replacement therapies, bupropion and varenicline (an α4β2 nicotinic acetylcholine receptor partial agonist), are approved by the U.S. Food and Drug Administration (FDA) as first-line treatments for nicotine dependence.50 Varenicline has been demonstrated to be significantly more efficacious than bupropion alone for smoking cessation.51 Furthermore, prolonged use of varenicline beyond the initial induction phase has proven useful in maintaining smoking abstinence.52 Clonidine and nortriptyline are recommended as second-line treatments.47 A systematic review of extant smoking cessation studies indicates self-help strategies alone only marginally affect quit rates, whereas individual and combined pharmacotherapies and counseling either alone or in combination can significantly increase rates of cessation.53
Biology of Lung Cancer
The specific events that trigger malignant transformation of bronchoepithelial cells are unknown in the vast majority of cases. However, it is clear that exposure to environmental carcinogens, such as those found in tobacco smoke or asbestos fibers, induce or facilitate the transformation (extrinsic component).54 The contribution of the extrinsic carcinogen on transformation is modulated by genetic variations in genes (intrinsic component) that affect aspects of carcinogen metabolism, such as the conversion of procarcinogens to carcinogens and their subsequent inactivation.55 These genetic variations occur at relatively high frequency in the population. Their contribution to an individual’s lung cancer risk is generally low, but because of their population frequency, their overall impact on lung cancer risk could be high. Epidemiological studies further suggest that a familial predisposition to lung cancer exists that is independent of tobacco smoke exposure. One study found evidence for an autosomal dominant model linked to 6q23-25,56 but other studies have proposed a complex multigene model for inherited risk.57 As mentioned above, familial clustering of lung cancer cases has been reported with an inherited T790M mutation in the EGFR gene.34,35 The identification of individuals at particularly high risk for the development of lung cancer could justify more intense screening regimens, and the identification of the responsible chromosomal loci for lung cancer susceptibility genes could allow the development of specific chemopreventive strategies.
Environmental factors, as modified by inherited modulators, likely affect specific genes by deregulating important pathways to enable the cancer phenotype. Particularly important in lung cancer are acquired abnormalities in ras, Rb, p53, Akt, LKB, and BRAF (reviewed in Fong et al58). However, the most clinically significant acquired genetic abnormalities in lung cancer are the mutation of the EGFR59–62 and the anaplastic lymphoma kinase (ALK) fusion.65–65 EGFR mutations occur in approximately 20% of lung cancer patients and are primarily in exons 19 (in-frame deletions usually of four amino acids LREA) and 20 (L858R point mutants), and result in constitutive signaling and AKT activation66 and are associated with very high response rates (60% to 90%) to the specific tyrosine kinase inhibitors gefitinib and erlotinib.67 ALK fusions have been reported in 3% to 7% of lung cancer patients and are associated with a 57% response to crizotinib.68 The EGFR and ALK abnormalities are non–overlapping. Interestingly, both EGFR mutations and ALK fusions are associated with younger age, light (<10 pack-year) and nonsmokers and adenocarcinoma histology, and the frequency of EGFR mutations is much higher in Asian than Western populations (30% to 70% vs. 10%).68–72 Response to specific EGFR or ALK inhibitors are often dramatic, and occur even in heavily pretreated patients, demonstrating that tumors with these mutations are “addicted” to the activation of this pathway. Almost all patients with these dramatic responses, however, develop progressive, resistant disease. The most common mechanism of acquired resistance to EGFR tyrosine kinase inhibitors is a second-site mutation within exon 20 that results in an amino acid substitution at position 790 in EGFR, from threonine to methionine (T790M).73,74 To date, several second-site mutations within the ALK tyrosine kinase domain have been reported as mechanisms of acquired resistance to the ALK tyrosine kinase inhibitor crizotinib.75
In spite of the incontrovertible high response rate in patients with EGFR mutations, analysis of samples from BR.21, a placebo-controlled, randomized, clinical trial of erlotinib in second-line non–small cell lung cancer (NSCLC) showed that the presence of an EGFR mutation was not associated with prolonged survival,76 but in univariate analysis, amplification of the receptor was associated with improved survival.77 Interestingly, there was a survival benefit in the entire erlotinib arm compared with placebo, as well as every subset of patients, including smokers and patients with squamous cell cancers,78 suggesting that factors other than mutation may contribute to clinical benefit, and that this was not necessarily related to objective response rates. Other assays, including serum proteomics, show potential for selecting patients likely to achieve clinical benefit.79,80
The histologic sequence of events that leads to the various forms of lung cancer is not well understood, and it is clearly different for the various histopathologic entities.81–84 Current knowledge suggests that squamous cell carcinoma arises in a relatively ordered progression that includes squamous metaplasia and carcinoma in situ (CIS). Peripheral adenocarcinomas are thought to arise from atypical adenomatous hyperplastic lesions, but this process is much more obscure, largely because this type of lesion is much less accessible by bronchoscopy. Small cell carcinoma might arise from neuroendocrine hyperplasia, but evidence in support of this hypothesis is scarce.
The pathology of premalignancy is discussed in more detail in the next section, but the molecular biology of premalignancy is of great clinical importance, not only to better understand the process of cancer development, but to provide potential therapeutic targets to intervene in this process and intermediate biomarkers to assess risk and evaluate candidate chemoprevention strategies. Premalignant lesions of the lung have been investigated for molecular alterations in NSCLC, but much less information is available for small cell lung cancer (SCLC).85 Microdissected specimens derived from normal, hyperplastic, metaplastic, dysplastic, and CIS, as well as invasive neoplastic foci of patients with lung cancer, were studied for gene mutations, promotor hypermethylation, and allele loss. Results obtained to date suggest that allele loss on chromosome 3p is the earliest event, followed by allele loss/hypermethylation on chromosome 9p and, subsequently, on chromosome 8p.84 Loss of heterozygosity at the p53 gene locus (17q13.1) is relatively rare (10%) and occurs predominantly at the dysplasia or CIS stage. Point mutations in the p53 gene86 and the EGFR gene,87 however, have been observed in morphologically normal bronchial epithelium obtained from the airways of patients with lung cancer. In contrast, K-ras gene mutations represent a late event, found only in CIS or invasive cancers.88 In addition to copy number changes and mutations, alterations in the expression of the retinoic acid receptor β have also been used as a molecular marker for premalignancy and as an intermediate biomarker for chemoprevention trials.89
The fate of morphologically or molecularly abnormal areas is currently an area of intense research. One study showed that 54% of patients with high-grade dysplastic lesions developed lung cancer within 2 years, but 80% of these arise in a different part of the lung than the CIS.90 None of the patients with low-grade dysplastic lesions progressed to cancer in this study. Of the low-grade lesions in this study, 82% spontaneously regressed, and 18% remained unchanged, and none progressed to CIS. Preliminary data also suggest that abnormal regions display reproducible molecular changes on repeat biopsies over time, and that these and additional abnormalities can be observed in tumors arising from these lesions.91
Part of the problem with the studies of preneoplasia is that we may be evaluating the microscopic appearance and molecular characteristics of the wrong population of cells. The vast majority of the respiratory epithelium (and most other tissues) is thought to be terminally differentiated and incapable of sustained replication; however, one theory holds that a small subset of the cells have unlimited, but normally tightly regulated, replicative potential. These are the so-called pulmonary stem cells,92,93 whose biology is very poorly understood. The stem cell concept may also underlie the failure of standard medical therapies to eradicate lung cancers, even when there is a clinical complete response. The theory is that therapies have been refined to induce measurable reductions in the bulk tumor mass, whereas true replicative potential exists only in a small subset of “cancer stem cells” that are not effectively targeted by current therapies. Specific isolation of these cells and development of stem cell–targeted therapies may thus not cause rapid tumor regressions, but rather result in significantly increased long-term survival.
Pathology
In recent years, significant advances in the treatment of pulmonary adenocarcinoma have affected the pathological diagnosis of lung cancer. The latest World Health Organization (WHO) classification of lung tumors from 200494,95 (Box 72-1) is a purely morphologic classification and geared towards resection specimens. Most pathological diagnoses of lung cancer, however, are rendered on small biopsies or cytologic specimens. A new multidisciplinary classification of lung adenocarcinoma has been proposed jointly by the International Association for the Study of Lung Cancer (IASLC), American Thoracic Society (ATS), and European Respiratory Society (ERS) (Box 72-2).96 Although based predominantly on histology, its purpose is to provide an integrated clinical, molecular, radiologic, and pathological approach to the classification of lung adenocarcinoma. In addition, this classification addresses the diagnosis of lung cancer in small biopsies and cytologic specimens and emphasizes the use of immunohistochemistry for the subtyping of non–small cell carcinoma.
Tissue and Cytologic Diagnosis of Lung Cancer
The diagnosis of lung cancer can be made on tissue specimens such as endobronchial and transbronchial biopsies or resected specimens, or by assessment of cytological specimens such as transbronchial or transthoracic fine-needle aspirates, bronchial brushing and washings, bronchoalveolar lavage or sputum or pleural fluid. The diagnostic yield depends on several factors including location (accessibility) of the tumor, tumor size, tumor type, or technical aspects of the diagnostic procedure, including the experience level of the bronchoscopist and pathologist.96 In general, central lesions such as squamous cell carcinomas, small cell carcinoma, or endobronchial lesions such as carcinoid tumors, are more readily diagnosed by bronchoscopic examination, whereas peripheral lesions, such as adenocarcinomas, and large cell carcinomas are more amenable to transthoracic fine-needle aspiration (FNA) or biopsy. Diagnostic accuracy for most specimens in distinguishing small cell carcinoma and non–small cell carcinomas is excellent, 97 with less accuracy for subtypes of non–small cell carcinoma96 if immunohistochemical staining is not used.
Bronchoscopic specimens include bronchial brushings, washings, bronchoalveolar lavage, and transbronchial fine needle aspiration. Of these, transbronchial FNA consistently demonstrates the highest sensitivity, surpassed only by the use of a combination of bronchoscopic specimens.96–100 Overall sensitivity for combined use of bronchoscopic methods is approximately 80%, and with tissue biopsy, the yield increases to 85% to 90%.96,97,99 In fact, transbronchial FNA is more often diagnostic than transbronchial biopsy when the lesion of interest is submucosal.96,101
Like transbronchial fine-needle aspirate specimens, transthoracic fine-needle aspirate specimens are also diagnostically useful, yielding diagnostic material in 70% to 95% of cases. Sensitivity is highest for larger lesions and peripheral tumors.102 In general, fine-needle aspirate specimens, whether transbronchial, transthoracic, or endoscopic ultrasound-guided, are superior to other specimen types.103 The superiority of fine-needle aspirate specimens is primarily a result of the higher yield of lesional tumor cells with fewer confounding factors, such as obscuring inflammation and reactive nonneoplastic cells.
Sputum cytology is inexpensive and noninvasive but has a lower yield than other specimen types because of poor preservation of the cells and more variability in acquiring a good-quality specimen. The yield for sputum cytology is highest for larger and centrally located tumors, such as squamous cell carcinoma and small cell carcinoma, although occasionally an accurate diagnosis is possible with tumors located more peripherally within the lung.96 The specificity for sputum cytology averages close to 100%, although sensitivity is generally less than 70%. The accuracy of sputum cytology improves with increased numbers of specimens analyzed and consequently, analysis of at least three sputum specimens is recommended. Sputum cytology has also been extensively studied with varying success as a screening tool for early detection of lung cancers. Sputum cytology is not currently recommended as a routine screening tool, but recent advances in molecular diagnostic techniques may result in a resurgence of its use.104–107