Cancer of the Breast
Antonio C. Wolff, Susan M. Domchek, Nancy E. Davidson, Virgilio Sacchini and Beryl McCormick
• Breast cancer is the most frequently diagnosed cancer in women in the United States, accounting for an estimated 226,870 new cases (163,570 invasive cancers and 63,300 in situ carcinomas) and 39,510 deaths in 2012.
• In the United States, the age-specific incidence of breast cancer increases with age, to a lifetime risk of breast cancer of 1 in 8 (to 110 years of age); by age 40 years, approximately 1 in 203 women will have been diagnosed with breast cancer annually; at 60 years of age, the figure is 1 in 28 women.
• Incidence rates rose 21% from 1973 to 1990, but then began to decline; mortality rates stayed relatively constant until recently, when annual decreases were seen.
• A sharp decline in the incidence of breast cancer in the early 2000s followed a decrease in the use of postmenopausal hormone replacement therapy.
• Age, family history, and both endogenous and exogenous ovarian hormone exposure have an important effect on risk and have been incorporated into models that predict individual risk of breast cancer; diet, alcohol use, and other factors play a smaller role.
• Inherited mutations in BRCA1, BRCA2, PTEN, and TP53 play a role in the development of breast cancer and can be directly tested in individuals.
Biology and Estimation of Risk
• The expression of nuclear estrogen and progesterone receptors plays an important role in the differentiation and growth of normal breast epithelium and the response of breast cancer cells to hormonal therapeutics.
• ERBB2 (human epidermal growth factor receptor 2 [HER2]) is a growth-signaling molecule on the surface of normal breast cells that is overexpressed in approximately 20% of breast cancer tumors, contributing to growth autonomy and genomic instability.
• Molecular analyses have defined at least four biological subtypes of breast cancer, including the basal type, two luminal types (so-called luminal-A and luminal-B), and the HER2-positive type.
• BRCA1 and BRCA2 are tumor suppressor genes that play a critical role in the cellular response to DNA damage; inherited mutations in these genes are associated with an increased risk of breast cancer.
• Regardless of the criteria used by an individual physician and patient to define high risk, four possible actions may be taken, some of which can be used simultaneously: (a) enhanced surveillance, (b) behavioral modification, (c) chemopreventive strategies, and (d) prophylactic mastectomy or oophorectomy.
• Screening guidelines currently differ between organizations. The 2009 United States Preventive Service Task Force breast cancer screening guideline recommends biennial screening mammography for women ages 50 to 74 years and an individualized (rather than standardized) approach for women ages 40 to 49 years. The American Cancer Society continues to recommend yearly mammogram starting at age 40 years. The American Cancer Society also recommends that breast magnetic resonance imaging (MRI) be used in addition to mammographic screening in women with a lifetime risk of breast cancer greater than 20%.
• Microcalcification and soft-tissue density are the major indications for biopsy in mammographic screening; the mammographic abnormality with the highest rate of malignancy is a mass density with associated calcification.
• For patients with breast symptoms or palpable abnormalities, mammography characterizes the suspicious area, evaluates the remainder of the breast for occult lesions, and assesses the contralateral breast.
• Malignant breast masses classically are nontender and firm, with irregular borders.
• Diagnostic methods include fine-needle aspiration cytology, needle-core biopsy with ultrasound or stereotactic guidance, and excisional biopsy, with or without wire or tack localization.
Management of Noninvasive Disease
• Lobular carcinoma in situ (LCIS) is a nonpalpable lesion that usually is discovered with another indicator for biopsy; it is more common in premenopausal women and accounts for 30% to 50% of cases of carcinoma in situ.
• LCIS has a propensity for multicentricity and bilaterality; it is an indicator of risk of subsequent invasive breast cancer. The rare pleomorphic variant of LCIS seems to have a more aggressive behavior and is considered more as a precursor of invasive lobular cancer rather than a marker of breast cancer risk. Some groups advocate complete excision with free margins.
• Management of LCIS has shifted toward observation after biopsy rather than mastectomy; increasing evidence shows that tamoxifen should be considered as a preventive approach.
• The multifocal nature of LCIS makes margin clearance an unrealistic and unnecessary goal.
• Unlike LCIS, ductal carcinoma in situ (DCIS) is almost always first identified by mammography; the peak incidence is between 51 and 59 years of age, and it accounts for most of the increasing number of carcinoma in situ lesions diagnosed.
• DCIS is more likely to be localized to one area of the breast; thus, most patients are candidates for breast conservation. Tamoxifen should also be considered following lumpectomy and radiation to reduce the risk of another ipsilateral or a new contralateral event, invasive or noninvasive.
Management of Early Stage Breast Cancer
• Patients should undergo a complete history and physical examination.
• Hemogram and renal and hepatic function tests as well as serum alkaline phosphatase evaluation should be performed in all patients.
• Bilateral mammography is indicated for all patients, and other breast imaging (ultrasound and MRI) should be used as needed for each individual patient; other imaging studies are recommended only to evaluate specific signs or symptoms or in patients with locally advanced disease.
• Prognostic factors include pathological tumor size, hormone receptors, axillary nodal status, histologic subtype, tumor grade, and perhaps age.
• HER2 is a strong predictive marker and accurate determination of HER2 status identifies patients with T1cN0 tumors and above who should be considered candidates for adjuvant therapy with a trastuzumab-based regimen.
• Expression of estrogen receptor and/or progesterone receptor is accepted as a predictive marker for response to endocrine therapy.
• Multiple gene expression profiling assays have been developed as prognostic tests and two of them are undergoing further prospective testing as a predictive marker for therapy selection.
• The current American Joint Committee on Cancer TNM staging system takes into account the increasing use of advanced imaging and pathology techniques, such as sentinel node biopsy and immunochemistry; it also considers the number of involved nodes as a strong prognostic factor.
• Sentinel lymph node mapping is now the standard of care for many women with early breast cancer as it is more accurate and less morbid than axillary dissection.
• For patients with stages I and II disease, breast conservation and modified radical mastectomy are the major therapeutic options; for most patients, breast conservation is the preferred approach.
• Factors that affect local control in breast conservation include pathological margin involvement, extensive intraductal component, age, and the presence of multiple tumors.
• Adjuvant therapy with cytotoxic drugs or endocrine treatment, or both, is recommended for patients with node-positive disease; anti-HER2–targeted therapy should also be considered for HER2-overexpressing tumors.
• Pathological tumor size, hormone-receptor and HER2 status, histologic subtype, and nuclear grade are used to select patients with node-negative disease for adjuvant therapy.
• The results of the serial meta-analyses performed by the Early Breast Cancer Trialists’ Collaborative Group (EBCTCG) show that the survival benefits of adjuvant chemotherapy and endocrine therapy persist after 15 years of follow-up.
• Challenges that require resolution include identification of biological parameters that more precisely predict the natural history of disease and the response to systemic therapy; more effective treatments are clearly needed.
Management of Locally Advanced Disease
• Patients with locally advanced disease are a heterogeneous group, including those with T3N1, T0-3N2-3, and T4N0-3 disease.
• Multimodality therapy is recommended for virtually all patients with locally advanced disease; the sequence of systemic therapy, surgery, and radiation depends largely on the operability of the primary disease.
• For women with inoperable or inflammatory breast cancer (or both), preoperative chemotherapy is recommended, followed by surgery, radiation, and endocrine therapy, if appropriate. Patients with HER2-positive disease may benefit from addition of anti-HER2–targeted therapy. Preoperative endocrine therapy for patients with receptor-positive disease is also a reasonable option.
Management of Locally Recurrent Disease
• Local recurrence is an indicator of systemic relapse in most cases; an exception may be local relapse in a breast that has undergone conservation therapy.
• Selection of the treatment modality depends largely on the extent of local and regional failure and the presence of distant metastases.
• Surgical removal alone may be sufficient in some cases, but it often is combined with locoregional radiation and/or systemic therapy.
Management of Metastatic Disease
• Although a small percentage of patients with metastatic breast cancer achieve long-term disease-free survival, this stage of disease is generally not curable, and therapy is largely palliative.
• A wide range of systemic, local, and supportive therapies are available for the palliation of metastatic breast cancer. Primary breast surgery may be indicated in selected patients who are seen with stage IV disease.
• The selection of endocrine, cytotoxic, or biological therapy usually is based on disease-free interval, receptor status, HER2 status, site of metastasis, performance status, age, and previous exposure to systemic therapy.
• Anti-HER2 agents, such as trastuzumab, lapatinib, and pertuzumab, have changed the natural history of patients with HER2-positive metastatic breast cancer. Treatment with bisphosphonates or denosumab is indicated to minimize bone complications in patients with bone metastases.
Epidemiology
Incidence
Worldwide, breast cancer is the most common type of cancer among women.1 In the United States, approximately 163,570 new cases of invasive breast cancer, 63,300 in situ cases, and 39,510 deaths were expected in 2012.2 In women, breast cancer accounts for 29% of new cases of cancer and 14% of cancer deaths, second only to lung cancer as a cause of cancer-specific death. Approximately 1% of breast cancers occur in males and 90% of these are estrogen receptor (ER)-positive.3 Incidence rates of breast cancer decreased by 2% per year from 1999 to 2005, and then remained relatively stable from 2005 to 2008. The decline appears to be partly the result of a decrease in the use of postmenopausal hormone replacement therapy.4 Although incidence rates (all races combined) are substantially higher for women older than age 50 years, approximately 23% of breast cancers are diagnosed in women younger than age 45 years (Table 91-1).
Table 91-1
Risk of Breast Cancer in U.S. Women
Age Range (years) | Breast Cancer Risk |
30–40 | 1 : 227 |
40–50 | 1 : 68 |
50–60 | 1 : 42 |
60–70 | 1 : 28 |
Lifetime (to age 110 years) | 1 : 8 |
Data from www.cancer.gov/cancertopics/factsheet/Detection/probability-breast-cancer. Accessed 10/1/2012
Although breast cancer is the leading cause of cancer for women in the United States (overall incidence 121 per 100,000 women) followed by lung cancer (overall incidence 55.8 per 100,000 women), lung cancer was the leading cause of cancer death (40.1 per 100,000 women) followed by breast cancer (23.5 per 100,000 women) between 2004 and 2008.5 Around the world, there are large variations in incidence, mortality, and survival, which may be a result of several underlying complex factors, including age, ethnicity, diet, and lifestyles (including reproductive issues such as age at first birth and breastfeeding).1 Breast cancer is increasing in less-developed countries, supposedly related to changes in lifestyle factors. Most of our knowledge regarding specific risk factors has been derived from large observational studies in developed countries, such as the Nurses’ Health Studies (NHS)6 in the United States, and the “Million Women Study” in the United Kingdom (www.millionwomenstudy.org).7 Some of these risk factors are briefly summarized in the following sections.
Diet
Alcohol consumption is the best-established dietary factor associated with increased risk for breast cancer, although there is considerable interest in other dietary components.8 Initial studies correlated fat intake and decreased intake of fruit and vegetables with an increased risk for breast cancer, but more recent prospective studies have failed to confirm those observations.9–12 One randomized study (Women’s Healthy Eating and Living) in breast cancer patients of a low fat/high fruit and vegetable diet did not demonstrate a decreased risk of breast cancer recurrence.11 However, a second randomized study (Women’s Intervention Nutrition Study) did demonstrate a lower risk of recurrence with a decreased intake of dietary fat associated with modest weight loss in breast cancer survivors.12
Dietary phytoestrogens, such as those found in soybeans, have a chemical structure that is similar to that of 17β-estradiol and can bind to the ER to compete with estrogen. Their consumption may have a weak protective effect against breast cancer. More recent studies suggest an inverse association of soy intake and breast cancer in Asian populations (in which soy intake is generally high from birth) but not in non-Asian populations.13 Although there is significant interest in the association of vitamin D intake and breast cancer risk, there are insufficient data to draw conclusions regarding potential benefit at this time.14
Ionizing Radiation
The accumulated knowledge about radiation-related breast cancer risk in women derives mainly from epidemiological studies of patients exposed to diagnostic or therapeutic radiation and of the Japanese atomic bomb survivors. Low-dose radiation exposure to the breast is carcinogenic, and risk increases as a linear function of increasing dose. The risk is particularly high in the developing breast (i.e., before the age of 20 years), and is minimal for women exposed after menopause.15 Therapeutic radiation, particularly extended field radiation for Hodgkin lymphoma, has been associated with an increased risk of breast cancer. The age of exposure is important with the highest breast cancer risk seen in women undergoing mantle radiation around puberty.16
Exogenous Hormones
The NHS originally was set up to determine whether oral contraceptive usage was associated with an increase in breast cancer. An analysis of data from that study and more than 50 additional studies has confirmed that current users of exogenous hormones have a slightly increased risk of developing breast cancer.17 However, the mortality associated with this risk appears to be offset by a decreased risk of colon and ovarian cancer, such that there is no increase in overall cancer mortality associated with their use.6 The Women’s Health Initiative randomized study of hormone replacement therapy (HRT) in postmenopausal women demonstrated an increased risk of invasive breast cancer with the combination of estrogen plus progesterone therapy,18 but not with estrogen alone.19 Other studies have also demonstrated that breast cancer risk depends on type of HRT (estrogen alone vs. combination) and time of initiation (with an increased risk seen when HRT was started soon after menopause).20 Age-adjusted incidences of invasive breast cancer decreased in the United States between 2002 and 2003 by 7%, reflecting an absolute decrease of approximately 14,000 breast cancer cases (Fig. 91-1). This decrease was limited to women 50 years of age or older, occurred primarily in ER-positive tumors, and coincided closely with a dramatic decrease in the use of HRT following the publication of the Women’s Health Initiative results that demonstrated no cardiovascular benefit for HRT.4
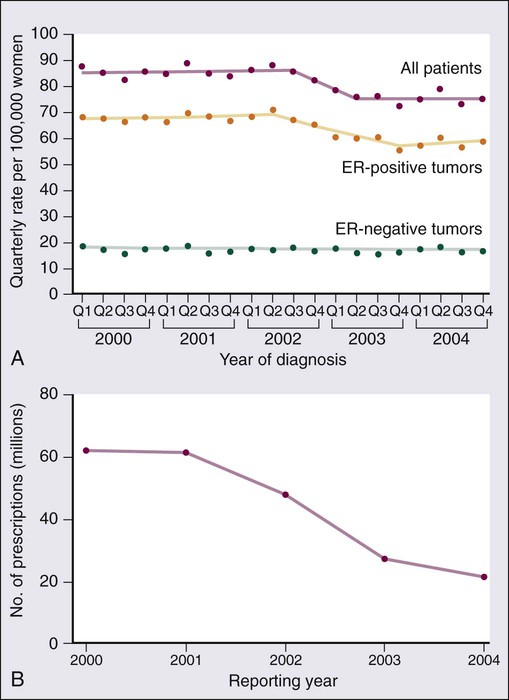
Reproductive Factors and Endogenous Hormones
Breast cancer risk consistently has been correlated with an earlier age of menarche, later age of menopause, nulliparity, and late age of first birth, all of which determine the cumulative number of ovarian cycles. This finding is consistent with the correlation between estrogen levels and breast cancer risk. In postmenopausal women, an increased risk of breast cancer has been demonstrated in those with estradiol levels in the highest quartile.21
Obesity and Body Habitus
There is a clear association between obesity and breast cancer risk. Women with a body mass index (BMI) of 25 to 30 (overweight) or BMI greater than 30 (obese) have been shown to have an elevated risk of breast cancer. In addition, an increased risk of breast cancer death has been seen with increasing BMI.22,23 The increased risk of breast cancer with obesity in postmenopausal women appears to be mediated, at least in part, by elevated endogenous estrogen and, possibly, insulin levels.24 An increased risk of breast cancer has also been associated with increasing height, with a relative risk of 1.17 (95% confidence interval [CI], 1.15 to 1.19) for every 10 cm of additional height.25
Familial History and Predictive Models of Breast Cancer Risk
Breast cancer is approximately twice as common among first-degree relatives of breast cancer patients as in those women with no family history of the disease. The two most important breast cancer susceptibility genes, BRCA1 and BRCA2, were identified by linkage analyses in the mid-1990s but account for less than 20% of familial clustering of breast cancer.26 Since then, rare germline mutations in several other genes, such as TP53 and PTEN, have been shown to confer a substantially increased risk of breast cancer. Given that linkage analyses have failed to yield additional susceptibility loci, it is likely that most of the remaining genetic susceptibility is a result of a number of moderate and low-penetrance alleles that coexist in highly penetrant combinations in a polygenic model, although some familial risk may still be a result of rare, undiscovered, high-penetrance gene mutations.27
Multiple moderate penetrance alleles including CHEK2, PALB2, ATM, BRIP, BARD, and others28 have been identified; mutations in these alleles are associated with a relative risk of breast cancer of 2 to 5. The clinical utility for testing for mutations in these genes is currently unclear. Recently breast cancer has been found to be a component of hereditary nonpolyposis colorectal cancer syndrome (HNPCC or Lynch) although the cumulative risk for breast cancer is less than for the classic component tumors (i.e., colon and uterine cancer).29
Apart from specific genetic susceptibility alleles, an increasing understanding of relevant risk factors has led to integrated efforts at evaluating a women’s individual risk,30 and several individualized risk-assessment tools are available, such as the Gail, Claus, Breast and Ovarian Analysis of Disease Incidence and Carrier Estimation Algorithm (BOADICEA), BRCAPRO, and Tyrer Cuzick models.31 One such tool is available on the National Cancer Institute (NCI) website at http://www.cancer.gov/bcrisktool/. These tools are being used for clinical counseling for healthy women perceived to be at risk and to determine candidates for chemoprevention with tamoxifen, raloxifene, and, most recently, exemestane. Given that for women living in the Western hemisphere the lifetime risk of breast cancer is approximately 11%, even a small relative risk reduction could translate into many thousands of lives saved.
Biology
Estrogen and Progesterone Receptors
Estrogen plays a key role in the development of both normal breast epithelium and breast cancer, and the modulation of estrogen levels is a key therapeutic modality for breast cancer. Estrogens interact with mammary epithelial cells via specific ERs that function as nuclear transcription factors.32 The two known receptors, ERα and ERβ, are encoded by different genes and share an overall sequence homology of approximately 30%, although the homology is higher in the DNA- and hormone-binding regions. ERα was the first ER to be isolated and is the receptor most closely associated with breast cancer; it is expressed mainly in the breast, uterus, and ovary. ERβ is more widely expressed and its relationship to breast cancer is less clear.33 Most of the literature that refers to ER (e.g., the staining of breast cancer cells as “ER-positive”) refers to ERα unless otherwise specified. The major ligand-dependent transcriptional activation activity (so-called AF-2) function of ER dislodges from a complex with heat shock proteins upon binding specific ligands, undergoes a conformational change, and homodimerizes. This homodimer then binds to the estrogen-responsive elements of the target genes, and recruits various coactivators (or corepressors) that further influence transcription.34 The participation in a multimeric signaling process likely contributes to the differential biological effects that estrogen and estrogen-related compounds (e.g., tamoxifen) can have in different target organs. ER also has a minor ligand-independent transcriptional activating function, which is modified by phosphorylation and can facilitate “cross talk” between ER activity and receptor tyrosine kinase signal transduction pathways. Approximately 70% of breast cancers express ER (i.e., they are ER-positive); these tumors tend to grow more slowly and appear better differentiated than ER-negative tumors. Moreover, antiestrogen therapy is effective both in preventing recurrence in the adjuvant setting and in prolonging survival once metastases have developed. However, the relationship between ER expression and cancer initiation is not clear, because ER-positive cells are nonproliferating in the normal breast epithelium that is found adjacent to proliferating cells, suggesting a paracrine effect. The paracrine effect hypothesis is further supported by evidence that even in most ER-positive tumors, only a minority of cells express ER by immunohistochemistry (IHC), and breast cancer stem cells do not express ER.
The progesterone receptor (PR), itself an estrogen-regulated gene, gives rise to two distinct isoforms, PRA and PRB, by alternative splicing. PRB appears to be more specific to breast cancer, whereas PRA is more widely expressed. Interestingly, a polymorphism in the PR promoter (+331 G/A) that increases transcription of PRB is associated with an elevated risk of endometrial and breast cancer.35 PR is variably expressed in ER-positive tumors, and this variability has prognostic relevance. ER-positive/PR-negative tumors occur more commonly in women older than 50 years, tend to be more aneuploid, and present as larger tumors with more frequent nodal involvement than ER-/PR-positive tumors. Furthermore, ER-/PR-positive tumors may be more likely to respond to antiestrogen therapy than ER-positive/PR-negative tumors.36
BRCA1, BRCA2, and Hereditary Susceptibility to Breast Cancer
It is estimated that 5% to 10% of all breast and ovarian cancer cases occur in the setting of inherited susceptibility because of a high-penetrance gene mutation, and the breast cancer susceptibility genes BRCA1 and BRCA2 have been identified as responsible for many of these cases.26 Women who carry a germline mutation in BRCA1 have a cumulative lifetime incidence of 50% to 85% of breast cancer and a 40% to 60% incidence of ovarian cancer, although estimates are somewhat lower in population-based series.26,37 There are a large number of BRCA1-interacting proteins including BARD, BRIP1, ABRAXAS, PALB2, RAD50, NBS1, and BRCA2. Germline mutations in the genes coding these proteins have also been associated with an increased risk of breast cancer, although the risk is significantly lower than with germline mutation in BRCA1 and BRCA2.38
The BRCA1 complex is integral in maintenance of genome integrity through roles in DNA damage repair, cell-cycle regulation, transcriptional regulation, and chromatin remodeling.39,40 Tumors that arise in heterozygote carriers of germline mutations usually have an inactivated wild-type allele and do not express a functional protein. Cells that lack BRCA1 or BRCA2 protein have a specific deficiency in the repair of DNA double-strand breaks by the conservative and relatively error-free mechanism of homologous recombination by gene conversion.40,41 Evidence for this defect is further supported by the finding that BRCA2 functions as part of the Fanconi anemia complex and actually is FANCD1. Fanconi anemia complementation group FA-N results from biallelic mutations in PALB2 (“partner and localizer of BRCA2”),42,43 whereas monoallelic mutations in the same gene are associated with an increased susceptibility to familial breast cancer.44 Moreover, several additional genes function in the same DNA repair pathway where germline mutations carriers are at increased risk of developing breast cancer—CHEK2, ATM, NBS1, RAD50, and BRIP1.45 Why these genes predispose primarily to breast and ovarian cancers remains unclear, and somatic mutations in them are not commonly found in sporadic breast cancers.
BRCA1-associated tumors usually are of the basal type (so-called triple negative: HER2-, ER-, and PR-negative). Conversely, sporadic triple-negative breast cancers may share phenotypic similarities with cancers arising in BRCA carriers.46 BRCA1 pathway dysregulation or “BRCAness” does also appear to be common in high-grade epithelial serous ovarian cancer.47,48 The identification of these genes and their function has important implications for prevention, diagnosis, and therapy of breast cancer in carriers and may have implications for sporadic ovarian cancer and, potentially, certain subtypes of triple-negative breast cancers.
Clinical management of carriers with BRCA1 or BRCA2 mutations is geared primarily toward preventing both ovarian and breast cancer.49 Prophylactic mastectomy, albeit an invasive procedure with cosmetic consequences, is the most effective strategy available; it is associated with a 90% reduction in the risk of breast cancer. Oophorectomy, in addition to reducing ovarian cancer risk by more than 80%, reduces the risk of breast cancer by 50%. Risk-reducing surgery may reduce the mortality for breast and ovarian cancer.50 Additionally, tamoxifen has been shown to reduce the risk of contralateral breast cancer by approximately 50% in both BRCA1 and BRCA2 carriers.51
Once cancer is diagnosed, therapy is dictated by standard prognostic and predictive features and no specific regimens are indicated on the basis of genetic predisposition at this time. The involvement of BRCA proteins in DNA repair by homologous recombination suggests that these tumors would be particularly sensitive to chemotherapies that induce DNA interstrand cross-links such as platinum salts. This hypothesis is supported by in vitro observations in both BRCA-null and FA cell lines. Significant responses to preoperative chemotherapy with cisplatin have been seen in a small number of BRCA1 mutation carriers52; however, it appears that breast cancers in BRCA1 mutation carriers are generally more chemosensitive compared with sporadic tumors.53 Finally, poly (ADP-ribose) polymerase 1 (PARP1) is involved in the base excision repair pathway; when this repair pathway is inhibited, double-strand breaks form at replication forks, and these are lethal to cells that cannot repair those breaks. Accordingly, BRCA-null cells are exquisitely sensitive to PARP inhibitors.54,55 Phase I and phase II studies have demonstrated exciting activity in BRCA1/2-related breast and ovarian cancer.56,57 It is hoped that more efficacious, less toxic treatment may be tailored to tumors that lack functional BRCA proteins.
TP53
TP53 (p53), also termed the guardian of the genome, is the most frequently mutated gene in human cancer. It plays a central role in sensing genotoxic and nongenotoxic stresses and transducing an antiproliferative effect (cell-cycle arrest or apoptosis) in response. It is activated and regulated by posttranslational modifications to the N-terminal region (e.g., phosphorylation and ubiquitination) and, binding as a tetramer to specific DNA sequences via a central DNA-binding core region, exerts its primary biological function by modulating the transcription of dozens of genes.58 In transgenic mice, loss of p53 is associated with multiple spontaneous tumors, although not of the mammary glands.59 However, p53 loss accelerates the appearance of mammary tumors in murine mammary tissue that also overexpresses MYC, HER2, IGF1, and/or WNT1. These genetic studies are consistent with a role for p53 loss late in tumor development.60 Indeed, the transgenic restoration of p53 function leads to tumor regressions.61
Inheritance of a p53 mutant allele causes the rare familial Li-Fraumeni syndrome, characterized by multiple early-onset cancers, including breast cancer in women who survive childhood cancers. Breast cancers in individuals with germline p53 mutations are frequently ER- and HER2-positive.62,63 Thirty percent to 50% of sporadic cases of breast cancers harbor somatic mutations in p53, based primarily on sequencing of exons 5 through 8 (the DNA-binding core), where approximately 90% of mutations are found. Currently, p53 mutations that have been observed in breast cancers are listed in the p53 database maintained by the International Agency for Research on Cancer.
Mutant p53 accumulates in the nucleus of neoplastic cells. Thus initial studies that described only a weak association between aberrant p53 and adverse prognosis in breast cancer relied on IHC detection; more recent analyses based on mutation detection have confirmed a strong association.64 Recently, p53 mutations from 1794 European patients with at least 10 years of follow-up were analyzed. Mutations in exons 5 through 8 were more common in ductal and medullary tumors, tumors with an aggressive phenotype (high-grade, large size, node-positive cases, and low hormone receptor content), and in women younger than 60 years. Furthermore, the presence of a mutation conferred an overall 2.27-fold increased relative risk of breast cancer-specific mortality, independent of other known prognostic markers (e.g., tumor size, node status, and estrogen and PR expression).65 Finally, although not all mutations confer the same biological properties (e.g., missense vs. nonmissense), all have similar prognostic utility.
Initial attempts at correlating p53 expression (by IHC) with resistance to chemotherapy have been supplanted by studies directly evaluating mutations. Some of these studies show breast cancers with p53 mutations to be associated with less benefit from both antiestrogens66,67 and anthracylines,68,69 although conflicting results also are reported.70 An overall analysis is complicated by different p53 assay methods, different disease settings (adjuvant, preoperative, and metastatic), and different combinations of drugs. In contrast, it appears that no clear relation between aberrant p53 and sensitivity to paclitaxel exists.73–73
p53 is an obvious target for cancer therapy, despite the apparent intractability of a loss-of-function genotype. Two approaches are being taken to overcome this limitation—small molecules to restore p53 function74 or inhibit its interaction with MDM2,75 or adenoviral-mediated gene delivery. Although the latter approach is approved for therapy of head and neck tumors in China, no clear activity in breast cancer has been demonstrated to date.76
ERBB2 (HER2)
The protooncogene ERBB2 (HER2) encodes a transmembrane tyrosine kinase receptor, one member of a family of related growth factor receptors that include EGFR (ERBB1), ERBB3 (HER3), and ERBB4 (HER4). The ligands for these receptors, termed heregulins, are a family of growth factors known to bind to ERBB3 and ERBB4, inducing heterodimerization with ERBB2 and subsequent transduction of downstream signals. Because HER2 does not bind ligand directly,77 the ability to heterodimerize with other family members that do bind ligand is crucial for its activity. Of these, HER3 appears to be the most important heterodimerization partner.78
The amplification and/or protein overexpression of HER2 is found in 20% of newly diagnosed breast cancers, and is associated with a more aggressive clinical course and decreased survival time compared with tumors with normal levels of HER2.79 Amplification leads to very high levels of protein expression and constitutive activation of the receptor tyrosine kinase signal transduction pathway, namely RAS-MEK and AKT,80 although the downstream effects may be more pleiotropic than originally believed.81 In contrast to EGFR, in which amplifications in lung cancers frequently harbor activating mutations in the kinase domain, such mutations have not been found frequently in breast cancer (see the Sanger Centre’s COSMIC database, http://www.sanger.ac.uk/genetics/CGP/cosmic/). The identification of HER2 as an oncogene in breast cancer facilitated the development of therapies directed against it. Trastuzumab, a humanized monoclonal antibody that binds to the extracellular domain of HER2, induces clinical responses as a single agent, prolongs survival in combination with chemotherapy, and decreases recurrence rates and improves survival in the adjuvant setting when given to women whose cancers overexpress HER2.84–84 Lapatinib, an oral, small-molecule tyrosine kinase receptor inhibitor active against HER2, and pertuzumab, a monoclonal antibody that inhibits dimerization between HER family members, have also been shown to be of benefit in metastatic, HER2-positive breast cancer. However, not all women with HER2-positive breast cancer respond to these agents, and studies aimed at elucidating mechanisms of resistance are providing further insight into the pathophysiology of HER2-positive breast cancer. For example, increased levels of insulin-like growth factor–1 receptor (IGF1R) may bypass HER2 blockade by trastuzumab and allow growth factor activation of AKT,85 and a similar outcome may result from PTEN loss, as discussed earlier. In addition, a truncated form of HER2 that lacks the extracellular domain but retains the kinase activity has been described to correlate with trastuzumab resistance.86
The amplicon containing HER2 frequently contains additional genes that influence therapeutic efficacy. Although initially it was thought that HER2-positive disease is more sensitive to anthracyclines, recent data suggest that this sensitivity is conferred by the topoisomerase II gene (TOP2A) when it is present in the amplicon.87 Modern genome-wide analysis methods currently are being applied to panels of cell lines,88 as well as to tissues from patients treated with anti-HER2 agents,89 to better understand mechanisms of resistance. The insights gained, in turn, are being exploited for further drug discovery. Both antibody and small molecule inhibitors of IGF1R as well as phosphatidylinositol 3-kinase (PI3K) and AKT kinase inhibitors currently are in clinical development for breast cancer, and synergy with anti-HER2 therapy has been demonstrated in vitro.90
PI3K and PTEN
Phosphatidylinositol-3,4,5-triphosphate (PIP3) is a lipid second messenger formed by PIP3 kinase (PI3K) in response to receptor tyrosine kinase signaling. PIP3 activates AKT and other related pathways to induce proliferation and inhibit apoptosis. Approximately 25% of human breast cancers harbor oncogenic activating mutations in the p110α catalytic subunits of PI3K (PIK3CA).91 This signal is inactivated by the opposing phosphatase action of the tumor suppressor gene PTEN.
Germline PTEN mutations cause a hereditary cancer predisposition syndrome known as Cowden syndrome, characterized by a high incidence of breast, uterine, thyroid, and skin neoplasms.92 PTEN is inactivated in a wide variety of human tumors as a result of either mutation or, more commonly, epigenetic silencing through methylation, including in breast cancer.93 PTEN loss is associated with genetic instability, and primary breast tumors that lack PTEN have increased aneuploidy.94 In addition, PTEN loss has been associated with a decreased likelihood of response to anti-HER2 therapy with trastuzumab.95 Recent data elucidating the relationship between presence of PIK3CA mutations and PTEN status show that they are inversely correlated. Furthermore, PIK3CA mutations are twice as common in HER2-positive or ER-positive tumors, suggesting that more than one aberration upstream of AKT may be necessary to overcome the effect of an intact PTEN.96 The relevance of PIK3CA mutations to the clinical activity of anti-HER2-directed therapy by trastuzumab and lapatinib is under active investigation.97
Breast Cancer Genome
The elucidation of genetic aberrations in cancer genomes (mutations, deletions, and amplifications) has led to a better understanding of cancer pathophysiology and is providing targets for rational drug development. With the determination of the DNA sequence of the normal human genome, efforts have shifted toward a more comprehensive analysis of cancer genomes. The first report of a comprehensive sequence analysis of 21,000 of the best studied and annotated human genes was published, including data from 11 breast and 11 colon cancer samples.98 This screen rediscovered cancer genes known to be associated with breast cancer (e.g., p53 and BRCA1) as well as genes not previously characterized as associated with cancer. This impressive effort suggests that an individual breast cancer harbors an average of about 90 mutant genes, of which a minority (approximately 12) are expected to contribute to the neoplastic process. Unfortunately, the functionally important mutations often differ among tumors (i.e., no tumor had more than six mutated cancer genes in common with any other tumor). However, when the genes were analyzed by functional groups, mutations in signal transduction pathway and transcription factor genes were found in nearly all breast tumor samples. As detailed below, rapid advancements in sequencing technology and bioinformatics have greatly expanded the information available.
Molecular Profiling in Breast Cancer
Simultaneous genome-wide determination of the relative abundance of RNA species (i.e., transcriptional profiling) combined with novel bioinformatic approaches has led to the development of molecular classifications based on continuous expression ranges of thousands of genes (as opposed to a binary determination of discrete factors, i.e., ER/PR and HER2). Tumors can be classified either according to transcripts that tend to segregate together according to underlying biological differences (unsupervised clustering) or sorted by a given end point such as prognosis or response to therapy (supervised clustering). The first of these studies identified an “intrinsic gene list” of 534 genes that defined five biological subtypes of breast cancer including the basal type (HER2-positive, cytokeratins 5 and 17 positive), the “triple negative” type (HER2-, ER-, and PR-negative), two luminal types (so-called luminal-A and luminal-B), and the normal-like type (Fig. 91-2).99 Studies by others have confirmed large-scale gene expression differences between ER-positive and ER-negative tumors, and have shown additional molecular subsets within these categories.100 This molecular classification of breast cancer is robust in that it is observed regardless of microarray platform type, and the various subtypes correlate with overall prognosis.101,102 Luminal-type cancers have the most favorable long-term survival, whereas HER2-positive and basal-like cancers may be more sensitive to chemotherapy but have the worst overall prognosis. Thus breast cancer is not a single disease with heterogeneous ER and HER2 expression but appears to be composed of three to five molecularly and clinically distinct subtypes.
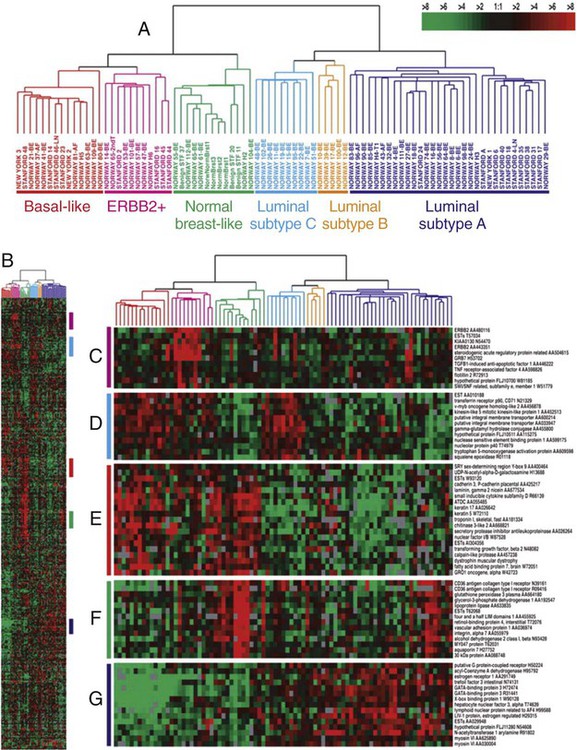
A similar approach can be taken by assaying DNA amplifications and deletions across the genome.103 Such data recently have been mapped against both the “intrinsic gene set” and clinical outcome data, and have the potential to identify high-level DNA amplification that, similar to HER2, may be useful as therapeutic targets.104 However, it should be noted that although these classifications are robust across a population, there is no standardized assay of measurement for assigning a molecular class to a new individual case. Furthermore, much of the molecular heterogeneity overlaps with conventional histopathology and is captured by determination of ER, PR, HER2 status, and tumor grade.100
Several multigene prediction scores that correlate with outcome and provide more accuracy than traditional histopathological markers have been retrospectively derived from microarray data, and, in some cases, prospectively validated on additional datasets. The best known examples are a 70-gene predictor set (Fig. 91-3)105,106 and a 21-gene predictor set,107,108 and both are commercially available. The 21-gene test consists of 16 target transcripts and five reference transcripts, including ER, PR, and HER2, and a “proliferation” group of five genes (which includes Ki-67 and cyclin B1); and a carefully constructed weighted formula is used to determine the overall “recurrence score.”107,108 Although this recurrence score provides a continuous risk variable, for clinical use it is pared down to three categories of low, intermediate, and high (of note, given the weighting schema, it is unlikely that a HER2-positive tumor would be classified as “low risk”). It is interesting that these two gene sets as well as the “intrinsic gene set” described earlier and yet another “wound healing” signature all seem to perform similarly when tested against the same clinical data set, even though they share few to none of the same genes.109 The ability of the 70-gene assay to determine which ER-positive node-negative patients are most likely to benefit from adjuvant therapy is being prospectively tested in the Microarray in Node-Negative Disease May Avoid Chemotherapy (MINDACT NCT00433589) trial. Similarly, the Trial Assigning Individualized Options for Treatment (Rx) (TAILORx) NCT00310180) study, which is testing the utility of adjuvant chemotherapy in patients who have an “intermediate risk” according to the test, has completed enrollment; low-risk patients received hormonal therapy only, whereas all high-risk patients were treated with both chemotherapy and hormonal therapy. The RxPONDER study (NCT01272037) is addressing a similar question in patients with node-positive disease, where patients with a recurrence score <=25 are being randomized to endocrine therapy alone or also receive chemotherapy. In the meantime, the Oncotype assay has been incorporated into the National Comprehensive Cancer Network (NCCN) algorithm for node-090 negative, ER-positive breast cancer patients.
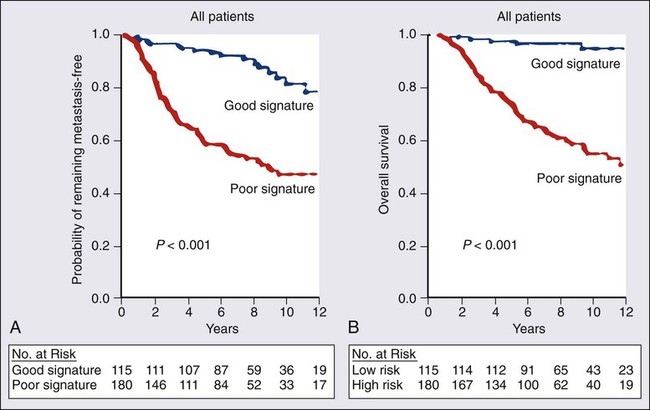
Comprehensive Genomic Analysis
More recently, comprehensive approaches have been undertaken to examine the molecular profiles of breast cancer. A comprehensive and integrated analysis of primary breast cancers by genomic DNA copy number arrays, DNA methylation, exome sequencing, messenger RNA arrays, microRNA sequencing and reverse-phase protein arrays (The Cancer Genome Atlas or TCGA) confirmed the existence of four main subtypes of breast cancer, and provided a wealth of data regarding genetic and epigenetic information for potential therapeutic targeting.110 In addition, the ENCODE project (Encyclopedia of DNA Elements) has recently mapped regions in non–protein-coding DNA of transcription factor association, chromatin structure, and histone modification that will no doubt enhance our understanding of breast cancer.111
Disseminated and Circulating Tumor Cells
From 20% to 45% of patients with primary operable breast cancer and 70% of patients with metastatic disease have disseminated tumor cells (DTCs) that can be detected in bone marrow112 or in lymph nodes.113 Historically, DTCs usually have been evaluated by IHC staining for various cytokeratin markers. Although their existence has been associated with a worse prognosis,112 the need for a relatively invasive procedure (a bone marrow biopsy) and the lack of sufficient specificity and sensitivity, as well as method standardization, have prevented the routine use of such information in clinical decision making.114 However, it is now possible to measure circulating tumor cells (CTCs) accurately by using an automated immunobead enrichment followed by pancytokeratin staining. Using this approach, patients with metastatic breast cancer and five or more CTCs per 7.5 mL of blood are predicted to have a significantly worse disease-free survival (2.1 months vs. 7 months) and overall survival (8.2 months vs. 18 months) than patients with fewer CTCs.115 This measurement may enable routine, minimally invasive assessment of disease progression and response to therapy. Furthermore, the ability to isolate CTCs allows the study of molecular markers such as HER2 amplification and their relationship to the primary tumor in real time. This approach has the potential not just to monitor patients but also to select specific therapy based on a molecular diagnosis obtained at the time of therapy (as opposed to relying on the original diagnostic biopsy). The clinical utility of CTCs is now being prospectively tested in a trial randomly assigning patients with 5 or more CTCs per 7.5 mL after 1 month of therapy to continue the same course or change systemic therapy.
Breast Cancer Stem Cells
Cancer cells have two somewhat contradictory traits. Although they originate from a single clone, they often display marked genetic, biological, and morphologic heterogeneity. Historically, the prevailing hypothesis was that genomic instability contributed to continuing evolution and emergence of different and ultimately more virulent subclones. This theory has been invoked to explain both progression (in terms of metastatic spread) and emerging resistance to therapy. Recently, an alternative explanation was provided by ground breaking work elucidating the “cancer stem cell” model (Fig. 91-4).116 Initially demonstrated in hematologic reconstitution and malignancies, cancer stem cells, although constituting a small minority of the cancer cell mass, retain the capacity for asymmetric division in which they both self-renew and give rise to more differentiated daughter cells. The latter, although forming the bulk of the tumor, are themselves incapable of endless replication. Given that stem cells are long-lived and slow to replicate, this would explain both the accumulation of genetic lesions over time as well as resistance to therapy targeting dividing cells. It would also explain the frequently observed concordance between primary and metastatic cells. For a long time this model for epithelial cancers has been theoretical, as it was technically difficult to identify these cells. However, such cells have been isolated from both normal mammary glands and breast cancers, based on the presence and absence of specific surface markers. Thus in mice, a singleLin+D29 (hi)CD24− cell can reconstitute a complete functional mammary gland in vivo, contributing to both luminal and myoepithelial lineages.117 Similarly, the ability to reconstitute human breast cancers in immunocompromised mice seems to reside within a minority CD44+, CD24(−/low) subclone. Notably, tumors formed by these cells gave rise to mixed populations of epithelial cells recapitulating the morphology of the parent tumor.118 These findings have several important implications for breast cancer therapy. As a first step, the molecular and biochemical pathways that control cancer stem cell division (such as Wnt and Notch pathways) might provide novel targets for therapy.119
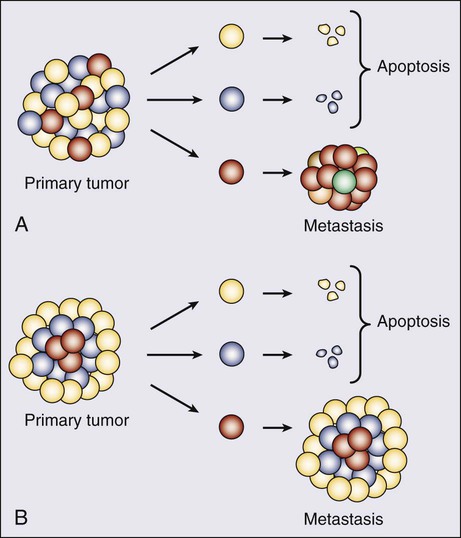
Management of Patients at High Risk for Breast Cancer
Increased Surveillance
As discussed in the section on mammographic screening, there is significant debate about the age at which to begin mammographic screening in average-risk women. Nonetheless, ample evidence supports increased surveillance in women at high risk for breast cancer. A number of prediction models for breast cancer risk are available, and surveillance strategies are developing rapidly, particularly for women who are BRCA1 and BRCA2 mutation carriers.120
There is general agreement that women at high risk for breast cancer are candidates for mammographic surveillance in their 40s, and in some cases, earlier initiation of mammographic screening may be appropriate. Shorter screening intervals may be necessary because of the accumulating evidence of unacceptably high rates of interval breast cancers, even with annual screening, in BRCA1 and BRCA2 mutation carriers. Other imaging modalities, such as magnetic resonance imaging (MRI), may be considered in these high-risk groups. Finally, although controversy remains about the role of breast self-examination in the general population, experts in the surveillance of high-risk women still recommend breast awareness for women with BRCA1 and BRCA2 mutations.121
Chemoprevention
Tamoxifen was studied as a chemopreventive agent for breast cancer in four randomized prospective clinical trials. A significant reduction in breast cancer risk with tamoxifen was seen in the National Surgical Adjuvant Breast and Bowel Project (NSABP) P-1 study122 and the International Breast Cancer Intervention Study (IBIS-I).123 Further analysis of the patients in the NSABP P-1 study showed that tamoxifen reduced the incidence of breast cancer among BRCA2 carriers by 62%, but not among BRCA1 carriers.124 Two European chemoprevention studies performed at the Royal Marsden Hospital125 and by the Italian Tamoxifen Prevention Study Group,126 respectively, did not show a decrease in the incidence of breast cancer in women from high-risk families using tamoxifen.
Based in large part on compelling evidence from the NSABP study, which showed a 49% reduction in overall risk of invasive breast cancer with the use of tamoxifen, a number of review groups, including the American Society of Clinical Oncology Technology Assessment Working Group, recommended that tamoxifen should be considered to reduce the risk of breast cancer in women with a defined 5-year projected risk of 1.66% or greater.127 However, this group of studies had considerable methodologic differences, and further studies are needed to better define the role of chemoprevention in high-risk women. Furthermore, the NSABP study was not designed to show a difference in death rate from breast cancer as a primary end point.
Two selective estrogen-receptor modulators (SERMs), tamoxifen and raloxifene, were compared in the NSABP STAR trial, and long-term follow-up of this trial demonstrated that raloxifene was slightly less effective in decreasing invasive breast cancer than tamoxifen, but was also less toxic.128 Finally, the aromatase inhibitor, exemestane, has been shown to decrease the risk of breast cancer in a randomized placebo-controlled, double-blind trial study. In the MAP.3 trial exemestane significantly reduced invasive breast cancers in postmenopausal women who were at moderately increased risk for breast cancer. During a median follow-up period of 3 years, exemestane was associated with no serious toxic effects and only minimal changes in health-related quality of life. Longer follow-up is required to confirm these data, including possible long-term side effects of the medication.129
For all of these agents it is not known whether they reduce the incidence of breast cancer by preventing the formation of cancer or by treating small, clinically occult cancers. The use of any of these agents requires an in-depth assessment of the risks and benefits of the specific drug in the individual patient; practical algorithms for assessing risk and benefit for tamoxifen and raloxifene chemoprevention are now available.130
Prophylactic Mastectomy or Oophorectomy
Prophylactic mastectomy and oophorectomy are controversial preventive approaches to breast cancer. Current data show that prophylactic mastectomy is effective in preventing breast cancer in patients with either a strong family history of breast and/or ovarian cancer131 or a genetic predisposition to breast cancer.50 Additional data show that prophylactic contralateral mastectomy can reduce the risk of breast cancer in patients with a previous diagnosis of unilateral disease.132 A high prevalence of premalignant lesions is seen in prophylactically removed breasts from women who are at hereditary risk for breast cancer.133 Reduction of breast and ovarian cancer risk with risk-reducing surgery has been shown to reduce the mortality for these diseases.50
The total and incremental life expectancy with prophylactic mastectomy is difficult to demonstrate. Statistical analysis has shown that depending on the assumed penetrance of the BRCA mutation, compared with surveillance alone, 30-year-old patients with early-stage breast cancer who have BRCA mutations may gain 2.9 to 5.3 years from prophylactic bilateral mastectomy and 0.6 to 2.1 years from prophylactic contralateral mastectomy.134 A recent survival analysis suggested that prophylactic mastectomy at age 25 years and oophorectomy at age 40 years maximized survival at 70 years of age; however, substituting enhanced breast cancer screening (with MRI and mammogram) for mastectomy resulted in similar survival.135 Given the impact on quality of life, the choice of prophylactic mastectomy is very personal. Still controversial is the gain in quality of life in these high-risk women who chose to have prophylactic mastectomy.136
Oophorectomy was first performed as a therapeutic procedure for advanced breast cancer more than 110 years ago and as hormonal adjuvant treatment of primary breast cancer more than 50 years ago. Uncertainty remains about the appropriate use of oophorectomy in adjuvant treatment, so it is not surprising that preventive oophorectomy in high-risk women remains controversial. Again, the identification of women with BRCA1 or BRCA2 mutations has provided an opportunity to study this prophylactic procedure. Studies with prospective follow-up have demonstrated that oophorectomy is associated with a decrease in breast and ovarian cancer risk, and enhanced breast cancer-specific survival, ovarian cancer-specific survival and overall survival. 50
Detection of Breast Cancer
Despite a lifetime probability currently estimated as 1 in 8 (in women who live to be 110 years of age) for breast cancer, only 30% of all women have one or more identifiable risk factors. Although these risk factors affect the parameters for breast cancer screening, current screening and education programs must include all women. The goal of breast cancer screening is early detection that will lead to a reduction in mortality. For breast cancer, the ideal screening program is sensitive enough to detect occult cancer with a minimum of false-positive findings. In addition, a screening program for breast cancer should be safe, relatively easy to provide to the public, acceptable to both patients and physicians, and cost-effective. A lower stage at diagnosis is associated with improved 5-year survival (Tables 91-2 and 91-3).
Table 91-2
Cancer of the Female Breast: Number of Cases and 5-Year Relative Survival Rates (%) by Age (20+ Years) and American Joint Committee on Cancer Stage
Histology* | AJCC Stage | |||||||||||
Total | I | II | III | IV | Unknown | |||||||
Cases | 5-Year RSR (%) | Cases | 5-Year RSR (%) | Cases | 5-Year RSR (%) | Cases | 5-Year RSR (%) | Cases | 5-Year RSR (%) | Cases | 5-Year RSR (%) | |
Total | 257,730 | 87.1 | 108,346 | 100.0 | 91,989 | 86.2 | 16,928 | 57.2 | 11,222 | 19.9 | 29,245 | 83.4 |
Adeno, NOS | 5559 | 62.2 | 1,137 | 99.0 | 1,341 | 81.4 | 453 | 49.4 | 1,460 | 15.3 | 1,168 | 64.9 |
Tubular adeno | 3771 | 100.0 | 2,939 | 100.0 | 286 | 95.8 | 13 | † | 8 | † | 525 | 99.7 |
Infiltrating ductal | 183,122 | 87.5 | 79,900 | 100.0 | 68,437 | 85.1 | 10,597 | 57.5 | 6,493 | 20.3 | 17,695 | 83.6 |
Scirrhous adeno | 456 | 81.7 | 172 | 94.3 | 188 | 83.9 | 16 | † | 30 | 13.5 | 50 | 71.7 |
Mucinous adeno | 6476 | 98.3 | 3,643 | 100.0 | 1,665 | 94.8 | 176 | 75.0 | 120 | 33.8 | 872 | 95.7 |
Comedo | 5020 | 89.9 | 2,218 | 99.3 | 1,653 | 82.7 | 223 | 51.3 | 82 | 19.4 | 844 | 96.0 |
Lobular | 20,140 | 91.6 | 7,640 | 100.0 | 7,594 | 93.0 | 1,600 | 72.6 | 921 | 30.5 | 2,385 | 87.9 |
Infiltrating duct and lobular | 16,060 | 92.9 | 6,801 | 100.0 | 6,564 | 91.4 | 1,013 | 69.8 | 375 | 29.0 | 1,307 | 89.5 |
Inflammatory carcinoma | 2668 | 34.1 | <5 | † | 25 | 49.5 | 2,003 | 40.9 | 570 | 11.2 | 67 | 21.6 |
Paget | 1937 | 82.6 | 498 | 95.8 | 524 | 77.7 | 193 | 46.3 | 66 | 14.3 | 656 | 93.0 |
Papillary adeno | 1646 | 94.5 | 741 | 100.0 | 463 | 92.3 | 67 | 85.7 | 43 | 34.2 | 332 | 92.6 |
Adenoid cystic/cribriform | 712 | 100.0 | 409 | 100.0 | 177 | 95.3 | 14 | † | 6 | † | 106 | 96.3 |
Other adeno | 4261 | 89.1 | 1,494 | 98.7 | 2,077 | 88.8 | 208 | 57.7 | 96 | 21.2 | 386 | 84.6 |
Medullary | 3122 | 89.5 | 1,037 | 98.2 | 1,703 | 88.8 | 131 | 63.2 | 33 | 29.6 | 218 | 75.1 |
Other non-adeno | 5785 | 64.8 | 699 | 99.2 | 945 | 80.1 | 348 | 42.4 | 952 | 13.8 | 2,841 | 70.1 |
*Excludes 45,033 stage 0 cases.
†Statistics not displayed because there were fewer than 25 cases.
From http://www.seer.cancer.gov/publications/survival/surv_breast.pdf, p 104.
Table 91-3
Cancer of the Female Breast: Number of Cases and 5-Year Relative Survival Rates (%) by Tumor Size and Regional Lymph Nodes Involved, Ages 20+ Years
Tumor Size (mm) | Nodes Involved | |||||||||
Total | 0 Nodes | 1–3 Nodes | 4+ Nodes | Unknown Number | ||||||
Cases | 5-Year RSR (%) | Cases | 5-Year RSR (%) | Cases | 5-Year RSR (%) | Cases | 5-Year RSR (%) | Cases | 5-Year RSR (%) | |
Total | 302,763 | 89.3 | 148,192 | 98.8 | 43,418 | 86.8 | 26,923 | 65.5 | 84,230 | 81.2 |
1–4 | 21,530 | 100.0 | 9,721 | 100.0 | 563 | 89.9 | 197 | 76.6 | 11,049 | 100.0 |
5–9 | 37,075 | 100.0 | 23,816 | 100.0 | 2,261 | 98.1 | 541 | 81.1 | 10,457 | 100.0 |
10–19 | 93,875 | 97.2 | 58,654 | 100.0 | 14,035 | 94.5 | 4,475 | 80.1 | 16,711 | 91.8 |
20–29 | 54,610 | 88.0 | 27,139 | 95.4 | 12,194 | 87.9 | 6,440 | 72.7 | 8,837 | 75.7 |
30–39 | 23,880 | 78.1 | 9,497 | 91.0 | 5,704 | 79.0 | 4,412 | 63.8 | 4,267 | 61.3 |
40–49 | 11,786 | 72.1 | 3,866 | 88.2 | 2,692 | 76.0 | 2,753 | 60.2 | 2,475 | 54.2 |
50–99 | 17,015 | 63.2 | 4,120 | 88.8 | 3,135 | 72.5 | 5,054 | 52.7 | 4,706 | 45.7 |
100+ | 2580 | 46.8 | 382 | 83.0 | 290 | 59.6 | 670 | 46.6 | 1,238 | 32.6 |
Diffuse | 3734 | 35.1 | 218 | 84.0 | 350 | 51.1 | 907 | 39.6 | 2,259 | 27.0 |
Unknown* | 36,678 | 84.9 | 10,779 | 100.0 | 2,194 | 81.8 | 1,474 | 63.1 | 22,231 | 78.4 |
*Unknown size category includes Paget disease of the nipple with no demonstrable tumor.
From http://www.seer.cancer.gov/publications/survival/surv_breast.pdf, p 109.
During the last 40 years, an intense effort has been mounted to evaluate the efficacy of mammography, clinical breast examination, and patient self-examination as tools for breast cancer screening. These efforts have included a number of case-control and cohort clinical trials as well as eight major randomized, prospectively controlled studies. More than 650,000 women have participated in the randomized trials alone.137,138
Film screen mammography was the “gold standard” for many years. Digital imaging offers a lower average dose of radiation, improvement in storage and retrieval, and easier access to images and computer-assisted diagnosis, which must be weighed against the higher cost of digital imaging equipment.139
Computer-aided diagnosis enables a digital reading of the mammogram to identify areas that meet a computer pattern of discrimination. Newer applications “learn” from the radiologist’s over-read of their identification points and are being evaluated.140,141 The goal for optimizing breast cancer screening must be a higher rate of false-positive findings so that the risk of false-negative findings may be virtually eliminated. In breast cancer screening, the discovery of smaller tumors has the added benefit of increasing the number of patients who can be treated by breast-conserving approaches. Although mammography is the best screening tool, it detects only 85% to 90% of biopsy-proven cancers. Mammography is not a substitute for tissue sampling and histologic evaluation of any palpable abnormality, nor is it a substitute for careful physical examination. Other imaging tools are also under evaluation for early detection of breast cancers in young women with dense breasts. These are discussed in the following section.
Screening
The efficacy of screening for occult cancer depends heavily on the following factors: (a) tumor growth rate; (b) the sensitivity of the test related to tumor volume; and (c) the interval between screens. Growth rates of breast cancer vary, and some younger women have more rapidly growing tumors. In determining the efficacy of screening, four biases must be considered: lead-time bias, length bias, selection bias, and overdiagnosis bias. Lead-time bias is the interval that the diagnosis has been advanced by screening. Length bias concerns the timing of detection. When screening is infrequent, fast-growing tumors are not detected as early in their natural history as more slowly growing tumors. Thus the outcome of cancers detected by screening is better than that for interval cancers. Selection bias is an obvious factor. For example, women who participate in breast cancer screening may have been shown to be more health conscious; they also are more likely to obtain Pap smears, use seat belts, and not smoke.142 Thus it is likely that their outcomes would be better, even in the absence of screening. Overdiagnosis has always been a concern in screening for cancer. Detection of breast lesions of questionable malignancy affects mortality data, because these lesions would not be diagnosed without screening. It is not known how many of these very early in situ cancers would progress to become invasive malignancies.
Before 1977, no formal guidelines existed for screening women for occult breast cancer. The publication of the Health Insurance Plan (HIP) of Greater New York Screening Project, conducted in the 1960s, led the NCI and the American Cancer Society to support a nationwide breast cancer screening project to evaluate further the efficacy of screening by mammography and physical examination. The Breast Cancer Detection Demonstration Project took place in 29 centers, and 280,222 women were screened over a period of 5 years.143
For the first time, the results of the HIP study provided strong evidence that early detection of breast cancer increased the duration of survival. Since the HIP trial was published, seven additional randomized trials of mammography and two of breast self-examination have been reported. The results of these trials have been included in a 2002 meta-analysis commissioned by the United States Preventive Service Task Force (USPSTF) (Table 91-4).137 Between the ages of 39 and 74 years, the summary relative risk (RR) for death from breast cancer was 0.84% for the use of screening mammography. Much like the findings of some of the individual studies included in this analysis, greater reduction in risk was seen in women older than the age of 50 years. The summary RR for women 40 to 49 years old was 0.85, compared with 0.78 for those 50 years of age and older. Of interest, this same meta-analysis noted “direct evidence of effectiveness among older women, limited to two trials that included women over 65 years of age.’
Table 91-4
Results of Randomized, Controlled Trials of Mammography Among Women 39 to 74 Years of Age
Study | Age (years) | Median Follow-up (years) | Breast Cancer Deaths/Total Women | Breast Cancer Death Rate per 1000 Women | Relative Risk for Death from Breast Cancer (95% Cl) | Absolute Risk Reduction per 1000 Women | No. Needed to Invite to Screening* | ||
Screened Group | Control Group | Screened Group | Control Group | ||||||
n/n | |||||||||
MAMMOGRAPHY ALONE | |||||||||
Stockholm† | 40–64 | 13.8 | 82/39 139 | 50/20 978 | 2.10 | 2.38 | 0.91 (0.65–1.27) | 0.288 | 3468 |
Gothenburg† | 39–59 | 12.8 | 62/20 724 | 113/29 200 | 2.99 | 3.87 | 0.76 (0.56–1.04) | 0.878 | 1139 |
Malmö† | 45–70 | 17.1 | 161/21 088 | 198/21 195 | 7.63 | 9.35 | 0.82 (0.67–1.00) | 1.712 | 584 |
Swedish Two-County Trial‡ | 40–74 | 17 | 319/77 080 | 333/55 985 | 4.14 | 5.95 | 0.68 (0.59–0.80) | 1.809 | 553 |
MAMMOGRAPHY PLUS CBE | |||||||||
CNBSS-1§ | 40–49 | 13 | 105/25 214 | 108/25 216 | 4.16 | 4.28 | 0.97 (0.74–1.27) | 0.12 | — |
CNBSS-2¶ | 50–59 | 13 | 107/19 711 | 105/19 694 | 5.43 | 5.33 | 1.02 (0.78–1.33) | −0.097 | — |
HIP![]() |
40–64 | 16 | 232/30 239 | 281/30 256 | 5.46 | 6.89 | 0.79 | 1.438 | 883 |
Edinburgh# | 45–64 | 13 | 156/22 926 | 167/21 342 | 6.80 | 7.82 | 0.79 (0.60–1.02) | 1.020 | 980 |
*Number needed to invite to screening to prevent one death from breast cancer 13 to 20 years after randomization.
†Nyström L, Andersson I, Bjurstam N, et al. Long-term effects of mammography screening: updated overview of the Swedish randomized trials. Lancet 2002;359:909–919.
‡Tabár L, Vitak B, Chen HIH, et al. The Swedish Two-Country Trial twenty years later. Updated mortality results and new insights from long-term follow-up. Radiol Clin North Am 2000;38:625–651.
§Miller AB, To T, Baines CJ, Wall C. The Canadian National Breast Cancer Screening Study-1: breast cancer mortality after 11 to 16 years of follow-up. A randomized screening trial of mammography in women age 40 to 49 years. Ann Intern Med 2002;137:305–312.
¶Miller AB, To T, Baines CJ, Wall C. Canadian National Breast Screening Study-2: 13-year results of a randomized trial in women aged 50–59 years. J Natl Cancer Inst 2000;92:1490–1499.
Shapiro S, Venet W, Strax P, Venet L. Current results of the breast cancer screening randomized trial: the health insurance plan (HIP) of greater New York study. In: Day NE, Miller AB, editors: Screening for breast cancer. Toronto: Hans Huber; 1988. pp. 3–15.
#Alexander FE, Anderson TJ, Brown HK, et al. 14 Years of follow-up from the Edinburgh randomized trial of breast-cancer screening. Lancet 1999;353:1903–1908.
Data from Humphrey LL, Helfand M, Chan BK, et al. Breast cancer screening: a summary of the evidence for the U.S. Preventive Services Task Force. Ann Intern Med 2002; 137:347–360.
The controversy about the efficacy of screening for women 40 to 49 years of age is ongoing because breast cancer is less frequent in this age group, and there is a greater likelihood that mammographic abnormalities will prove benign on biopsy. To further address this controversy, the Cancer Screening Evaluation Unit in Sutton, United Kingdom, conducted a trial called Age, directed at women ages 40 to 41 years, comparing annual screening mammogram with routine medical care. In selecting this age group, the trial targeted women who would still be younger than 50 years through 10 years of follow-up screening. This trial did demonstrate “a reduction in breast cancer mortality” in the intervention group, in relative and absolute terms, which did not reach statistical significance.144
Because of the continued controversy about the value of mammography, especially in younger and older women, a 2009 systematic review was undertaken to update evidence since 2002 and it focused especially on women of average risk aged 40 to 49 years and 70 years or older.138 For women aged 39 to 49 years, eight trials provided data and the pooled RR for breast cancer mortality for women randomly assigned to mammographic screening was 0.85 which corresponded to a number needed to invite to screening to prevent 1 breast cancer death (NNI) of 1904. For women aged 50 to 59 years six trials provided data that showed a pooled RR of 0.86 with an NNI of 1559, and for women aged 60 to 69 years two trials gave a RR for breast cancer mortality of 0.68 with an NNI of 337. A single trial of women aged 70 to 74 years gave a RR of 1.12 with broad confidence intervals and inability to provide an NNI. Other discussion centers around the optimal frequency of mammography, as well as the value of clinical and breast self- examination.
Table 91-5 lists the current screening guidelines for mammography for the American Cancer Society, NCCN, and the USPSTF.145
Table 91-5
Suggested Screening Guidelines for Mammography from Some U.S. Organizations
AMERICAN CANCER SOCIETY
For women for normal risk, yearly mammograms are recommended starting at age 40 years. Clinical breast examination suggested about every 3 years for women in their 20s and 30s and every year for women age 40 years and older. Breast awareness is encouraged.
NATIONAL COMPREHENSIVE CANCER NETWORK
Between ages 20 and 40 years women at average risk are recommended to have a clinical breast examination every 1 to 3 years; breast awareness is encouraged. Beginning at age 40 years, an annual clinical breast examination and mammograms are recommended, and breast awareness is encouraged.
UNITED STATES PREVENTIVE SERVICES TASK FORCE
Women who are 50 to 74 years of age should receive screening mammography every 2 years. For women younger than the age of 50 years, regular biennial screening mammography should be an individual decision that takes into account patient context, including values regarding benefits and harms.
Patient Compliance
Over the last decade, aggressive public education programs conducted by the American Cancer Society, the NCI, and public interest groups have led to a dramatic increase in mammographic screening in the United States. A particular concern was its marked underuse by minority women, although the use of mammography has increased dramatically and the racial gap has virtually disappeared. A recent study concluded that ethnicity was “not associated” with seeking and obtaining a screening mammogram, once socioeconomic factors are considered.142 Interventions associated with an increased use of screening included providing women with information, especially by a health care provider urging compliance, and vouchers for free mammography. Media intervention has little effect on compliance and limited long-range benefit, but educational interventions targeting primary care physicians should become a priority.
Sensitivity of Mammography
The sensitivity, or accuracy, of mammography also is affected by the experience of the radiologist. Features with the highest positive predictive value for carcinoma were spiculated margins, irregular shape, linear calcification, and segmented or linear calcification distribution. In general, the positive predictive value of a Breast Imaging Reporting and Data System (BI-RADS) category 5 lesion is greater than 80%, whereas that of a category 4 lesion approaches 30% to 40%. The last decade has seen the development of full-field digital mammography. A recent multicentric trial comparing conventional with digital mammography showed an improvement over conventional film mammography in the detection of breast cancer in young, premenopausal, and perimenopausal women, and in women who have dense breasts. However, there was no significant difference in diagnostic accuracy between digital and film mammography in the population as a whole or in the other predefined subgroups.139
Other Methods of Screening
To overcome some of the variables that affect the predictive value of mammography, a number of new technologies are being explored. Emerging techniques for breast screening, such as MRI, tomosynthesis, scintimammography, and ultrasound are under study in selected women who present difficulties in screening with mammography.146 Data increasingly suggest that women with very dense breasts and those with BRCA1 and BRCA2 mutations are more likely to have clinically occult carcinomas detected by other screening methods than by mammograms. Women who meet the criteria for hereditary breast cancer should be considered for additional screening as well. A number of options exist and could include the following: (a) initiation of screening 10 years before the onset of a first-degree relative’s breast cancer, or at 25 years of age; (b) the addition of MRI screening; (c) shorter screening intervals (e.g., every 6 months); and (d) the addition of ultrasound screening. A woman with certain BRCA1 or BRCA2 mutations may have a risk as high as 20% by 40 years of age, and MRI appears to be the most sensitive technique for screening these very young women. The role of clinical examination and breast self-examination in high-risk women seems more limited. Computer simulation modeling suggests that annual MRI at age 25 years with delayed alternating digital mammography at age 30 years is probably the most effective screening strategy in BRCA mutation carriers.147 Although MRI shows improved sensitivity, it has lower specificity than mammography and may lead to additional fine-needle aspiration (FNA) or core biopsy in these young women at high risk.
Screening in the Elderly Patient
The life expectancy for women (all races) in the United States increased from 77.4 years in 1980 to 80.4 years in 2002 (see http://www.cdc.gov/nchs/fastats/lifexpec.htm). Biological parameters suggest a higher prevalence of higher-risk tumors in younger women, with lower-risk tumors (better differentiated and hormone receptor-rich) occurring in older women. Tabar and colleagues148 showed that for a given tumor size, the likelihood of nodal involvement is lower in older women than in younger women, and these data in aggregate have led some, including the USPSTF, to suggest that it may be appropriate to increase the screening interval to 2 years in older women and stop screening mammography at age 75 years, although there are insufficient data to suggest an age when routine screening can be abandoned. At 75 years of age, the average additional life expectancy for a white or a black woman in the United States is 12 years or more. Even so, fewer elderly women are being screened annually, even those with a history of breast cancer. Although the benefits from screening appear to be seen in all age groups, and even in patients with comorbid disease, the magnitude of the benefit appears to diminish with age and with the severity of comorbid illnesses. Indeed it seems reasonable to forego screening mammography for women whose life expectancy is less than 5 years. Data from the U.S. National Health Interview Survey show an overall drop in the overall rate of mammography screening, from 70% in 2000 to 66% in 2005.149
Mammographic Abnormalities
The value of mammography relies heavily on the technical expertise available in obtaining the study, as well as on the experience of the radiologist interpreting the study. Vigorous compression is essential to even out the breast tissue over the film to provide more uniform exposure. Compression decreases the possibility that glandular areas will obscure masses or calcification. It also avoids motion, which causes loss of fine resolution. Additional views may be required to image all of the breast tissue, especially tissue adjacent to the chest wall. Magnification films are valuable for evaluating areas of architectural distortion and fine calcification. Evidence supports the importance of significant experience in performing mammograms and interpreting the results. Distinct advantages for the patient occur when mammography is provided by a dedicated mammography unit with a full-time technical team and an experienced radiologist. When more than one radiologist specializing in breast radiology reviews films, the accuracy of interpretation is further increased, and evidence also suggests that having mammography technologists review screening mammograms in addition to radiologist review may increase the rate of breast cancer detection.150
Masses
Benign masses typically are well defined, with sharp margins, and have little effect on the surrounding breast architecture. Fibroadenomas, papillomas, intramammary lymph nodes, and cysts are the most common causes of benign mammographic density (Fig. 91-5). Malignant breast densities classically have irregular borders that blend into the surrounding tissue and often appear to infiltrate the breast background tissue with a stellate appearance. Usually, there is some distortion of adjacent breast stroma. The mammographic abnormality that has the highest rate of malignancy is a mass density with associated calcification (Figs. 91-6 through 91-8). Examples of questions that should be asked include the following:
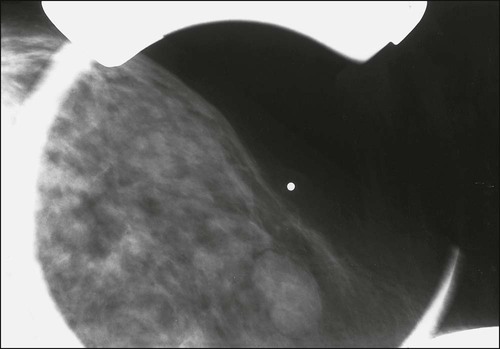
Calcifications
Malignant calcifications typically are linear, or small (<1 mm) in diameter, nonuniform in size, and clustered. Between 20% and 25% of clustered microcalcifications are positive for cancer on biopsy. Benign calcifications usually are larger and coarser, and are often round with smooth margins. Table 91-6 lists various types and distributions of calcifications. Benign causes of microcalcification include involuting fibroadenoma, arteriosclerosis, sclerosing adenosis, fat necrosis, and previous mastitis with ductal calcium deposits.
Table 91-6
Types and Distributions of Calcifications
Type of Calcification | Description |
TYPICAL BENIGN | |
Skin (dermal) | Typical lucent-centered deposits that are pathognomonic. Atypical forms may be confirmed by tangential views to be in the skin. |
Vascular | Parallel track or linear tubular calcifications that are clearly associated with blood vessels. |
Coarse or popcornlike | The classic calcifications produced by an involuting fibroadenoma |
Large rodlike | Benign calcifications forming continuous rods that occasionally may be branching. They usually are >1 mm in diameter, and may have lucent centers, if calcium surrounds rather than fills an ectatic duct. These are the kinds of calcifications found in secretory disease, “plasma cell mastitis,” and duct ectasia. |
Round | When multiple, they may vary in size. They usually are considered benign, and when small (<1 mm), they often are formed in the acini of lobules. When <0.5 mm, the term “punctate” can be used. |
Lucent-centered | Benign calcifications ranging from <1 mm to ≥1 cm. These deposits have a smooth surface, are round or oval, and have a lucent center. The “wall” that is created is thicker than the “rim” or “eggshell” type of calcifications. Included are areas of fat necrosis, calcified debris in ducts, and occasional fibroadenomas. |
Eggshell or rim | Very thin, benign calcifications that appear as calcium deposited on the surface of a sphere. These deposits usually are <1 mm thick when viewed on edge. Although fat necrosis can produce these thin deposits, calcifications in the walls of cysts are the most common “rim” calcifications. |
Milk of calcium | Consistent with sedimented calcifications in cysts. On the craniocaudal image, they often are less evident and appear as fuzzy, round, amorphous deposits; on the 90-degree lateral view, they are sharply defined, semilunar, crescent-shaped, curvilinear, or linear, defining the dependent portions of cysts. |
Suture | Calcium deposited on suture material. They are relatively common in the postirradiation breast. They typically are linear or tubular in appearance, and knots often are visible. |
Dystrophic | Calcifications that usually form in the irradiated breast or in the breast after trauma. Although irregular in shape, they usually are >0.5 mm in size. They often have lucent centers. |
Punctate | Round or oval, >0.5 mm, with well-defined margins. |
INTERMEDIATE CONCERN | |
Amorphous or indistinct | Often round or flake-shaped calcifications that are so small or hazy that a more specific morphologic classification cannot be determined. |
HIGHER PROBABILITY OF MALIGNANCY | |
Pleomorphic or heterogeneous (granular) | Usually more conspicuous than the amorphic forms. They are neither typically benign nor typically malignant irregular calcifications. They vary in size and shape and are usually <0.5 mm in diameter. |
Fine linear, or fine linear branching (casting) | Thin, irregular calcifications that appear linear, but are discontinuous and <0.5 mm wide. Their appearance suggests filling of the lumen of a duct involved irregularly by breast cancer. |
DISTRIBUTION MODIFIERS | Used as modifiers of the basic morphologic description. These terms describe the arrangement of the calcification. Multiple similar groups may be indicated when there is more than one group of calcifications that are similar in morphology and distribution. |
Grouped or clustered | Although historically the term “clustered” has connoted suspicion, the term is now used as a neutral distribution modifier and may reflect benign or malignant processes. It is used when multiple calcifications occupy a small volume (<2 mL) of tissue. |
Linear | Calcifications are arrayed in a line that may have branch points. |
Segmental | Worrisome in that their distribution suggests deposits in a duct and its branches, raising the possibility of multifocal breast cancer in a lobe or segment of the breast. Although benign causes of segmental calcifications exist (e.g., secretory disease), this distribution is of greater concern when the morphology of the calcifications is not specifically benign. |
Regional | Calcifications scattered in a large volume of breast tissue and not necessarily conforming to a duct distribution. They are likely benign, but are not everywhere in the breast, and do not fit the other, more suspicious categories. |
Diffuse/scattered | Calcifications that are distributed randomly throughout the breast. |
Adapted from Breast Imaging Reporting and Data System. Reston, VA: American College of Radiology; 1998. p. 27.
Ultrasound
Ultrasound is of greatest use when the mammographic findings are equivocal and when examination shows multiple areas of abnormality (Fig. 91-9). Ultrasound is very accurate (>95%) in diagnosing breast cysts. Cysts have well-demarcated, smooth margins and an echo-free center (Fig. 91-10), and usually are rounded and thin-walled and produce distal shadowing. Clear cysts require no further evaluation. Complex cysts that contain evidence of tissue or debris may be aspirated to clarify whether they are simply cysts or represent cystic degeneration of a tumor.
Approach to the Patient
Management of the Palpable Mass
Finding a breast mass by accident or regular self-examination is not uncommon. One study found that 57% of all women presenting to an urban breast clinic in the year 1999 complained of a mass.151 Determination of whether a palpable abnormality is malignant can be difficult, especially in premenopausal women. In general, if a palpable mass is determined to be solid, histologic diagnosis is required. An area of abnormal thickening in the breast that is not dominant and discrete (asymmetrical thickening compared with the same area in the opposite breast) usually suggests a benign change. Such areas of thickening may be safely observed for 1 or 2 menstrual cycles.
Patients with a palpable mass or a new breast symptom should have mammography and often an ultrasound as well (Fig. 91-11). The goals of diagnostic mammography for patients with breast symptoms or positive findings on examination are different from the goals of screening. For the patient with a breast lump, the goals are to characterize the area of palpable abnormality, to evaluate the remaining breast for signs of additional occult lesions, and to evaluate the contralateral breast.
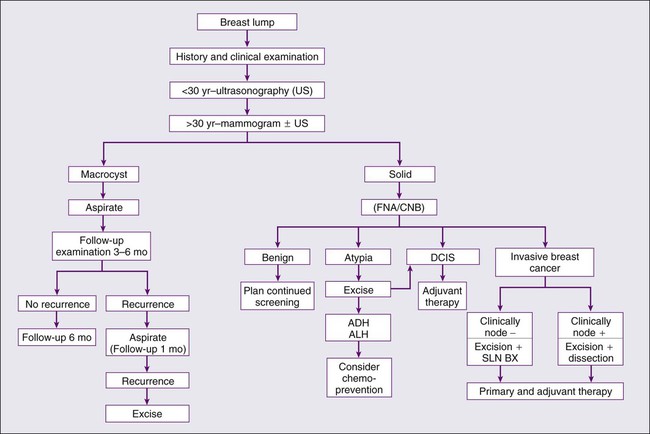
A solid mass, as confirmed by mammography, ultrasound, or needle aspiration, requires histologic confirmation of its character. If the cytologic findings indicate cancer, then definitive therapy is planned. If they do not, then cytologic or core biopsy diagnosis must be consistent with the findings that led to the biopsy. If the lesion is highly suspicious, negative findings on FNA or core biopsy will not completely remove suspicion unless they show a histologic explanation of the findings. The finding of proliferative epithelium, or atypia, affects the risk of subsequent breast cancer. Atypical hyperplasia is found in 4% to 10% of benign biopsy specimens. Associated risk relates strongly to family history. Table 91-7 shows the relative associated risks for patients with benign histologic findings.
Table 91-7
American Board of Pathology Histologic Classification of Benign Disease
Histopathology | Approximate Relative Risk |
Nonproliferative | No added risk |
Cysts | |
Duct ectasia | |
Calcification | |
Fibroadenoma | |
Milk ductal epithelial hyperplasia | |
Sclerosing adenosis | No added risk |
Papillomatosis | Slight added risk |
Radial scars | |
Complex sclerosing lesions | ? |
Moderate florid hyperplasia | 1.5 : 1 to 2 : 1 |
Atypical hyperplasia (ductal and lobular) | 4 : 1 |
Extensive ductal involvement of atypical hyperplasia | 7 : 1 |
Lobular carcinoma in situ | 10 : 1 |
Ductal carcinoma in situ | 10 : 1 |
Management of the Nonpalpable Mammogram Abnormality
• A localized soft-tissue density that was not previously seen on mammography
• A localized soft-tissue density that has changed on successive studies
• A localized soft-tissue density with ill-defined borders or stellate distortion of the stroma (or both)
• A focus of suspicious microcalcification, with or without soft-tissue density (see Table 91-6)
If these findings are seen on screening mammograms, diagnostic mammograms—including magnification views—are required to confirm the abnormality. Often, mammographic changes are not as clear as those listed in Table 91-6. The mammography report may state “cannot exclude an early breast cancer,” or “clinical correlation is recommended.” In these situations, all previous mammograms must be obtained for comparison. If the mammographic abnormality is believed to warrant histologic evaluation, several options exist: needle core biopsy (NCB) under ultrasound guidance if the abnormality is easily visualized by ultrasound; NCB with a dedicated stereotactic mammography unit; and wire localization and guided excisional biopsy with specimen radiography. Clearly, the expense of tissue sampling is considerably less if stereotactic NCB is used and proves reliable. Candidates for NCB include patients with highly suspicious mammographic findings, those with multiple suspicious lesions in the same breast, and those with lesions of low suspicion.
When lobular carcinoma in situ (LCIS) is found in a NCB, in situ or invasive cancer can be found in about 25% of cases at surgery.152 On biopsy, approximately two benign tumors are found for each cancer that is discovered, but some authors argue that biopsy should be performed when the chance of finding cancer reaches 10%. Driving the rate of positive biopsy findings even lower, with the goal of decreasing the incidence of interval cancers, would significantly increase the overall cost of screening. The refinement in technology for the use of NCB suggests that this may be a way to reduce cost and provide an alternative to short-interval follow-up mammography. As with wire-localized biopsy, however, careful evaluation of the criteria for the use of NCB is required. Performing NCB biopsy on all patients would clearly defeat the goal of reducing cost. It is estimated that approximately 10% of women who undergo screening require either diagnostic biopsy or short-interval (4- to 6-month) follow-up. False-negative rates for NCB are reported to be as low as 2%, a rate similar to that for wire-localized–guided excisional biopsies. No cost savings will occur if every negative stereotactically guided NCB is followed by excisional biopsy.
The techniques used for wire-localized excisional biopsy are shown in Figures 91–12 through 91–15. Wire-localized excisional breast biopsy is highly accurate and has in good to excellent cosmetic results. The key to success is the accuracy of the needle or wire placement, which should go directly into the area to be removed. Other techniques of preoperative localization using radioisotopes, radioactive seeds, and cryo assistance, have been described with possible surgical advantages in obtaining more of the lesion in the surgical specimen and higher rate of negative margins.153 The surgical specimen is sent to the radiology department, where it is filmed and compared with the mammogram to ensure that the suspicious lesion or microcalcifications are contained within the specimen. For patients with a benign biopsy finding, repeat mammogram is obtained 4 to 6 months later to reestablish a baseline and confirm clearing of the suspicious abnormality.
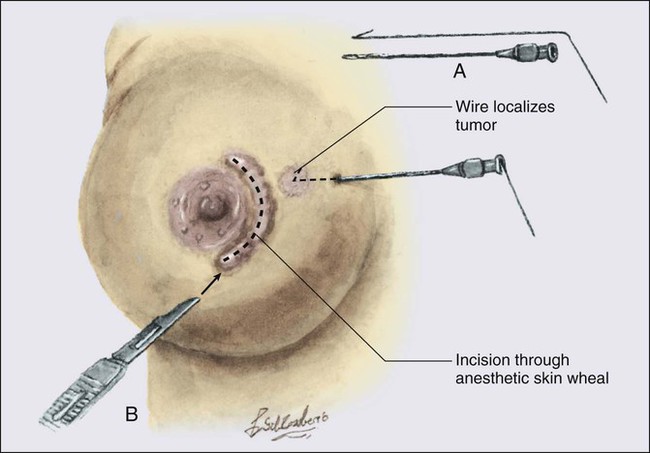
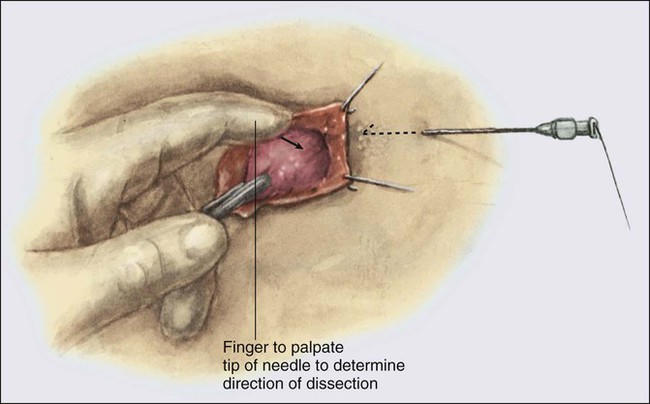
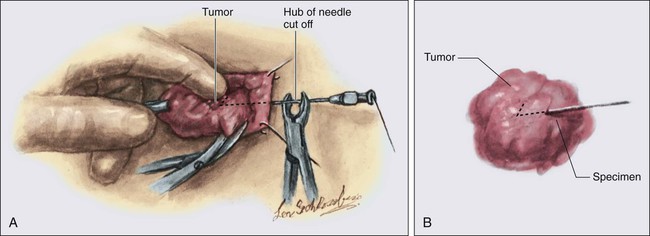
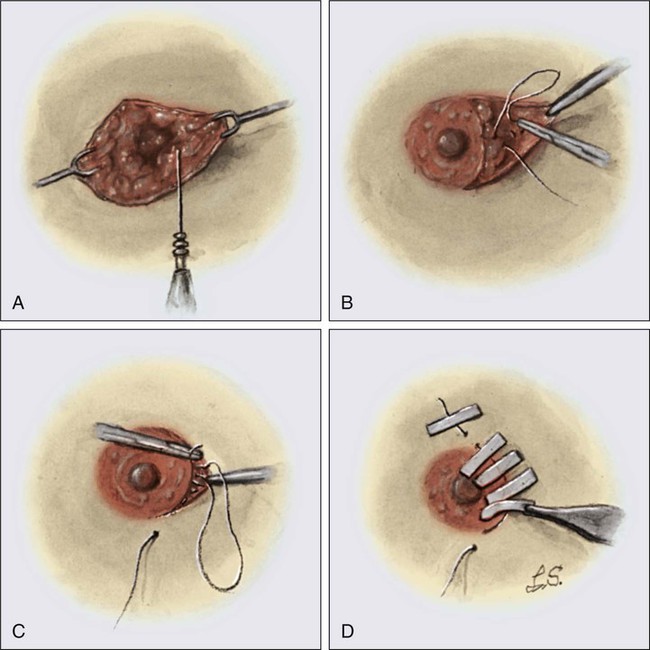
Management of the Magnetic Resonance Imaging Abnormality
With the increasing use of MRI in women at high risk for breast cancer, radiologists and breast surgeons often are faced with an MRI lesion not visible with mammography and ultrasound. A focal ultrasound in an MRI suspicious lesion fails to reveal a sonographic correlate in up to 77% of cases,154 and MRI-guided percutaneous, vacuum-assisted biopsy should be performed.155 Open surgical biopsy with MRI needle localization is the other option to diagnose a suspicious MRI lesion. Because the nonmagnetic wire used for the MRI localization is thinner than the wire used for ultrasound or stereotactic localizations, possible transection of the wire during surgery may make removal of the area more challenging. Because the presence of the lesion in the specimen cannot be confirmed by x-ray, postexcisional MRI may be performed 1 month after the procedure to confirm removal of the lesion.
Staging and Prognostic Evaluation
2010 Update of the TNM Staging System
The seventh edition of the American Joint Committee on Cancer staging system for breast cancer includes important changes in each of the TNM elements (Table 91-8).156 Changes in the tumor (T) classification include: (a) an attempt to estimate size of DCIS and LCIS; (b) use of microscopic measurement if a small invasive cancer was entirely submitted in one paraffin block; use of the clinical measurement to determine T at presentation in patients treated with neoadjuvant treatment, and use of gross and microscopic histology to describe the T size after neoadjuvant treatment; (c) the description of simultaneous ipsilateral carcinomas whether in the same quadrant or in separate quadrants; and (d) the classification of Paget disease based on the underlying cancer (e.g., Tis, T1, …) or as Tis (Paget) if no underlying cancer. Changes in nodes (N) classification include: (a) the use of the new stage IB to describe small T1 tumors with node micrometastases (N1mi); (b) the description of isolated tumor cell (ITC) clusters or single cells; (3) the deletion of the modifier (sn) modifier if six or more sentinel nodes are identified on gross pathological examination; and (4) the expansion of ITCs clusters to include small clusters of cells up to 0.2 mm, or if nonconfluent or nearly confluent, clusters of up to 200 cells in a single histologic lymph node cross section. As for metastases (M), the new M0(i+) category is defined by DTCs in bone marrow, as CTCs, or if incidentally observed in other tissues as clusters of up to 0.2 mm. Of note, however, M0(i+) patients with no clinically or radiographically detectable metastases continue to have their staging defined by T and N.
Table 91-8
American Joint Committee on Cancer TNM Staging System for Breast Cancer
TNM Staging | |||
PRIMARY TUMOR (T) | |||
Definitions for classifying the primary tumor (T) are the same for clinical and for pathological classification. If the measurement is made by the physical examination, the examiner uses the major headings (T1, T2, or T3). If other measurements, such as mammographic or pathological measurements, are used, the subsets of T1 can be used. Tumors should be measured to the nearest 0.1-cm increment. | |||
TX | Primary tumor cannot be assessed | ||
T0 | No evidence of primary tumor | ||
Tis | Carcinoma in situ | ||
Tis (DCIS) | Ductal carcinoma in situ | ||
Tis (LCIS) | Lobular carcinoma in situ | ||
Tis (Paget) | Paget disease of the nipple with no tumor | ||
Note: Paget disease associated with a tumor is classified according to the size of the tumor. | |||
T1 | Tumor 2 cm or less in greatest dimension | ||
T1mic | Microinvasion 0.1 cm or less in greatest dimension | ||
T1a | Tumor more than 0.1 cm but not more than 0.5 cm in greatest dimension | ||
T1b | Tumor more than 0.5 cm but not more than 1 cm in greatest dimension | ||
T1c | Tumor more than 1 cm but not more 2 cm in greatest dimension | ||
T2 | Tumor more than 2 cm but not more than 5 cm in greatest dimension | ||
T3 | Tumor more than 5 cm in greatest dimension | ||
T4 | Tumor of any size with direct extension to (a) chest wall or (b) skin, only as described below | ||
T4a | Extension to chest wall, not including pectoralis muscle | ||
T4b | Edema (including peau d’orange) or ulceration of the skin of the breast or satellite skin nodules confined to the same breast | ||
T4c | Both T4a and T4b | ||
T4d | Inflammatory carcinoma | ||
REGIONAL LYMPH NODES (N) | |||
CLINIC* | |||
NX | Regional lymph nodes cannot be assessed (e.g., previously removed) | ||
N0 | No regional lymph node metastasis | ||
N1 | Metastasis to movable ipsilateral axillary lymph node(s) | ||
N2 | Metastases in ipsilateral axillary lymph nodes fixed to one another (matted) or in clinically detected ipsilateral internal mammary nodes in the absence of clinically evident axillary lymph node metastasis | ||
N2a | Metastases in ipsilateral axillary lymph nodes fixed to one another (matted) or to other structures | ||
N2b | Metastases only in clinically apparent ipsilateral internal mammary nodes and in the absence of clinically evident axillary lymph node metastasis | ||
N3 | Metastasis in ipsilateral infraclavicular lymph node(s), with or without axillary lymph node involvement, or in clinically apparent ipsilateral internal mammary lymph node(s) and in the presence of clinically evident axillary lymph node metastasis; or metastasis in ipsilateral supraclavicular lymph node(s), with or without axillary or internal mammary lymph node involvement | ||
N3a | Metastasis in ipsilateral infraclavicular lymph node(s) | ||
N3b | Metastasis in ipsilateral internal mammary lymph node(s) and axillary lymph node(s) | ||
N3c | Metastasis in ipsilateral supraclavicular lymph node(s) | ||
PATHOLOGICAL (PN)† | |||
pNX | Regional lymph nodes cannot be assessed (e.g., previously removed or not removed for pathological study) | ||
pN0 | No regional lymph node metastasis histologically | ||
pN0(i−) | No regional lymph node metastasis histologically, negative IHC | ||
pN0(i+) | Malignant cells in regional lymph node(s) ≤0.2 mm (detected by IHC including isolated tumor cell(s) | ||
pN0(mol−) | No regional lymph node metastasis histologically, negative molecular findings (RT-PCR) | ||
pN0(mol+) | No regional lymph node metastasis by IHC or histology, positive molecular findings (RT-PCR) | ||
pN1 | Metastasis in 1 to 3 axillary lymph nodes, or in internal mammary nodes with microscopic disease detected by sentinel lymph node dissection, but not clinically detected‡ | ||
pN1mi | Micrometastasis (greater than 0.2 mm, and/or more than 200 cells, none greater than 2.0 mm) | ||
pN1a | Metastasis in 1 to 3 axillary lymph nodes with a least one metastases >2 mm | ||
pN1b | Metastasis in internal mammary nodes with micrometastases or macrometastases detected by sentinel lymph node dissection but not clinically detected | ||
pN1c | Metastasis in 1 to 3 axillary lymph nodes and in internal mammary nodes with micrometastases or macrometastases detected by sentinel lymph node dissection, but not clinically detected) | ||
pN2 | Metastasis in 4 to 9 axillary lymph nodes or in clinically detected internal mammary lymph nodes in the absence of axillary lymph node metastasis | ||
pN2a | Metastasis in 4 to 9 axillary lymph nodes (at least one tumor deposit greater than 2.0 mm) | ||
pN2b | Metastasis in clinically detected internal mammary lymph nodes in the absence of axillary lymph node metastasis | ||
pN3 | Metastasis in 10 or more axillary lymph nodes, or in infraclavicular lymph nodes, or in clinically detected ipsilateral internal mammary lymph nodes in the presence of 1 or more positive axillary lymph nodes; or in more than 3 axillary lymph nodes and in internal mammary lymph nodes with micrometastases or macrometastases detected by sentinel lymph node biopsy but not clinically detected; or in ipsilateral supraclavicular lymph nodes | ||
pN3a | Metastasis in 10 or more axillary lymph nodes (at least one tumor deposit greater than 2.0 mm), or metastasis to the infraclavicular lymph nodes | ||
pN3b | Metastasis in clinically detected ipsilateral internal mammary lymph nodes in the presence of 1 or more positive axillary lymph nodes; or in more than 3 axillary lymph nodes and in internal mammary lymph nodes with micrometastases or macrometastases detected by sentinel lymph node dissection, but not clinically detected | ||
pN3c | Metastasis in ipsilateral supraclavicular lymph nodes | ||
DISTANT METASTASIS (M) | |||
MX | Distant metastasis cannot be assessed | ||
M0 | No clinical or radiographic evidence of distant metastasis | ||
cM0(+) | No clinical or radiographic evidence of distant metastases but deposits of molecularly or microscopically detected tumor cells in blood, bone marrow, or nonregional nodes ≤ without symptoms or signs of metastases | ||
M1 | Distant detectable metastases as determined by classic clinical and radiologic means and/or histologically proven >0.2 mm | ||
Stage Grouping | |||
Stage 0 | Tis | N0 | M0 |
Stage IA | T0 | N0 | M0 |
Stage IB | T0 or T1 | N1mi | M0 |
Stage IIA | T0 | N1 | M0 |
T1 | N1 | M0 | |
T2 | N0 | M0 | |
Stage IIB | T2 | N1 | M0 |
T3 | N0 | M0 | |
Stage IIIA | T0 | N2 | M0 |
T1 | N2 | M0 | |
T2 | N2 | M0 | |
T3 | N1 | M0 | |
T3 | N2 | M0 | |
Stage IIIB | T4 | N0 | M0 |
T4 | N1 | M0 | |
T4 | N2 | M0 | |
Stage IIIC | Any T | N3 | M0 |
Stage IV | Any T | Any N | M1 |
Note: Stage designation may be changed if postsurgical imaging studies show distant metastases, provided that the studies are carried out within 4 months of diagnosis in the absence of disease progression and provided that the patient has not received neoadjuvant therapy. | |||
Histopathological Type | |||
The histopathological types are the following | |||
IN SITU CARCINOMAS | |||
NOS, not otherwise specified; RT-PCR, reverse transcriptase polymerase chain reaction.
*Clinically apparent is defined as detected by imaging studies (excluding lymphoscintigraphy) or by clinical examination or grossly visible pathologically.
†Classification is based on axillary lymph node dissection with or without sentinel lymph node dissection. Classification based solely on sentinel lymph node dissection without subsequent axillary node dissection is designated (sn) for “sentinel node” (e.g., pN0[i+] [sn]).
‡Not clinically apparent is defined as not detected by imaging studies (excluding lymphoscintigraphy) or by clinical examination.
Used with the permission of the American Joint Committee on Cancer (AJCC), Chicago, Illinois. The original and primary source for this information is the AJCC Cancer Staging Manual, 7th ed. (2010) published by Springer-Verlag New York. (For more information, visit www.cancerstaging.net.) Any citation or quotation of this material must be credited to the AJCC as its primary source. The inclusion of this information herein does not authorize any reuse or further distribution without the expressed, written permission of Springer-Verlag New York, Inc., on behalf of the AJCC.
Pathological Factors for Invasive Carcinoma
The number of axillary lymph nodes involved with the disease remains an important prognostic marker (Table 91-9) and is inversely related to disease-free survival and overall survival along with tumor size (see Table 91-3). However, optimal characterization of tumor phenotype based primarily on the expression of ER, PR, and HER2, and secondarily on measures of proliferation like tumor grade, has become critical to assess risk of recurrence (prognosis) and likelihood of benefit from specific systemic therapies (prediction), and the clinical use of predictive and prognostic markers is discussed later in this chapter.
Table 91-9
Axillary Node Status and Outcome in Operable Breast Cancer
Authors | Survival (%) Related to Node Status | |||||
Negative Nodes | 1–3 Positive Nodes | 4 Positive Nodes | ||||
5 Years | 10 Years | 5 Years | 10 Years | 5 Years | 10 Years | |
Milan | 89 [91%] | — | 68 [53%] | — | 48 [31%] | — |
Royal Marsden | 66 [69%] | — | 70 [51%] | — | 42 [32%] | — |
MD Anderson | — | — | 91 [69%] | — | 53 [43%] | — |
Carter et al. | 92 | — | 81 | — | 57 | — |
Valagussa et al. | 88 [79%] | 83 [74] | 69 [46%] | 54 [33%] | 42 [26%] | 26 [15%] |
Ariel | 81 | 63 | 66 | 53 | 48 | 23 |
Fisher et al. | 78 | 65 | 62 | 38 | 32 | 13 |
Adapted from Yeh I, Fowble B, Viglione MJ, et al: Pathologic assessment and pathologic prognostic factors in operable breast cancer. In: Fowble B, Goodman RI, Glick JH, Rosato EF, editors. Breast cancer treatment: a comprehensive guide to treatment. St. Louis: Mosby-Year Book; 1991. p. 171.
Axillary staging is an essential procedure because of the strength of axillary lymph node status as a prognostic factor, and SLNB in experienced hands is now accepted as the preferred staging procedure for early stage tumors. Many pathological factors of the primary tumor, including histologic subtype, nuclear and histologic grade, and lymphatic or vascular invasion, have been evaluated as additional prognosticators of clinical course. Of interest, there is now evidence to show that immunohistochemical evidence of sentinel lymph node metastasis does not affect overall survival of women treated with SLNB and breast-conserving therapy that includes radiation therapy to the conserved breast. At the same time, occult bone marrow metastasis, although rare, is associated with decreased survival, although this information alone is not enough to guide decisions about adjuvant therapy.157
Most invasive breast cancers are epithelial neoplasms. The rarer sarcomas, lymphomas, and nonepithelial tumors are discussed elsewhere. Infiltrating breast cancers are histologically heterogeneous, but most of them are adenocarcinomas arising from the terminal ducts. Invasive ductal carcinoma, which accounts for approximately 85% of breast cancers, has no specific histologic features (Fig. 91-16). Invasive lobular carcinoma is characterized by single-filing (“Indian file”) of small, regular epithelial cells that tend to grow around ducts and lobules (Fig. 91-17) and accounts for 5% to 15% of breast cancers. The overall prognosis of classic invasive lobular cancer is thought to be similar to that of the invasive ductal subtype, although they respond poorly to preoperative chemotherapy.158
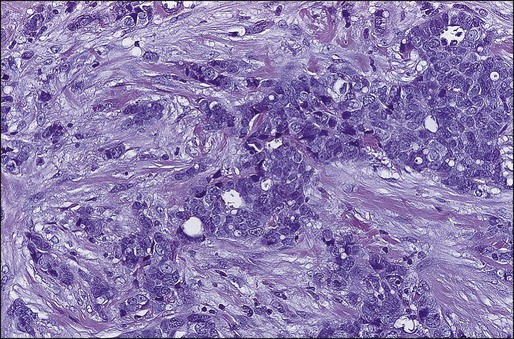
Many special types of breast carcinoma are less common than the invasive ductal and lobular variants and usually have a more favorable prognosis. These include mucinous, papillary, tubular, and adenoid cystic carcinoma. The better prognoses associated with these special histologic subtypes have been most clearly defined in node-negative patients. Patients with lymph node-negative disease with special tumor types up to 3 cm in diameter have, on average, a prognosis equivalent to that of patients with infiltrating ductal or lobular carcinoma up to 1 cm. Tubular cancers often resemble normal mammary ducts (Fig. 91-18) and appear to have a better prognosis, and many small tubular cancers are treated with local therapy alone. Mucinous (colloid) carcinoma is characterized microscopically by abundant accumulation of extracellular mucin around tumor cells (Fig. 91-19). A frond-forming growth pattern characterizes papillary carcinoma (Fig. 91-20).
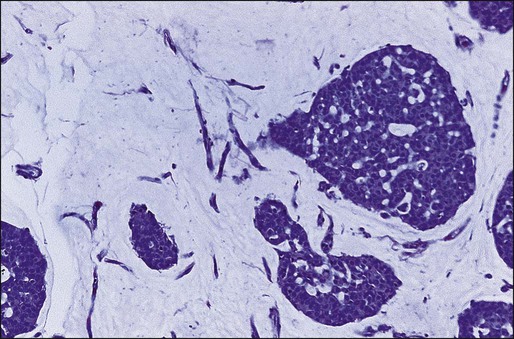
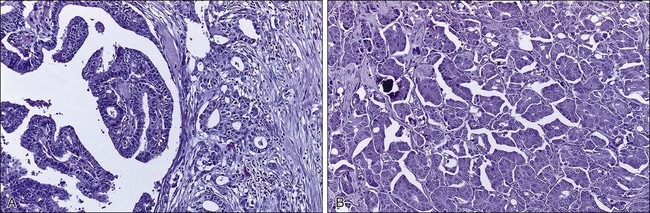
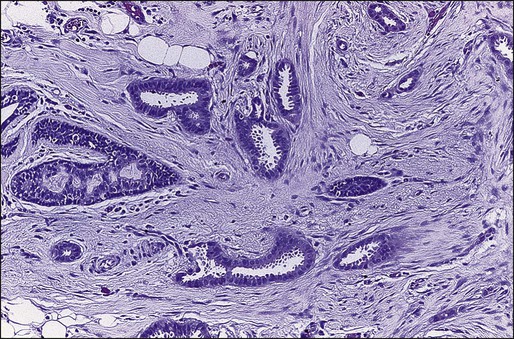
The histopathological features that define medullary carcinoma include a well-circumscribed border, intense reaction with lymphocytes and plasma cells, poorly differentiated nuclei, a syncytial growth pattern, and little or no intraductal carcinoma (Fig. 91-21). The more favorable prognosis requires the presence of all of these characteristics. Thus atypical medullary tumors do not have the same excellent outcome. Furthermore, the risk of incorrectly labeling an aggressive, poorly differentiated carcinoma as medullary carcinoma led some to exclude the latter from the special types of breast cancer with favorable prognoses.
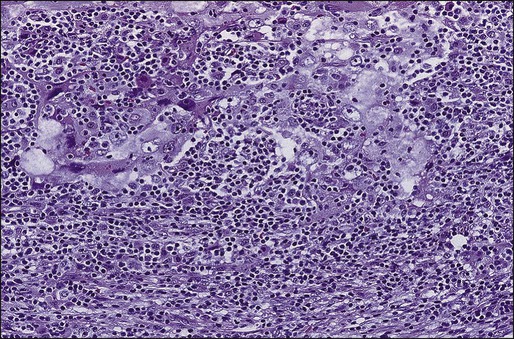
Studies of the prognostic value of other pathological factors, such as histologic and nuclear grade, vascular-lymphatic invasion, inflammatory response, and tumor necrosis, have shown considerable variation. The major criticism of nuclear grade is the lack of observer consistency in making this subjective classification. Various prognostic indices incorporating several parameters have been intensively studied, such as the Nottingham Prognostic Index.159