Pulmonary Complications of Anticancer Treatment
Neelesh Sharma, Ellen Kim and Mitchell Machtay
Radiation-Induced Lung Injury (Radiation Pneumonitis and/or Fibrosis)
• Risk factors include radiation dose and volume of lung irradiated, which may be expressed as mean lung dose or as the Vx, that is, the percentage of normal lung tissue irradiated to a dose above a certain threshold dose.
• Older age, co-morbidities (including chronic obstructive pulmonary disease), and/or low performance status are risk factors.
• The location of the tumor is a risk factor; irradiation of lower lobe primary lung tumors may carry a higher risk than irradiation of other tumors, although this may reflect the higher volume irradiated with lower lobe tumors.
• Biological factors can carry risk, including levels of circulating cytokines such as transforming growth factor–β and interleukin-6.
• The predominant symptoms are dyspnea and hypoxia, especially upon exertion.
• Fever (usually low grade if present at all), cough, pleuritic chest pain, and other pulmonary symptoms also frequently occur.
• Diffusing capacity of the lung for carbon dioxide is the most sensitive pulmonary function.
• Interstitial or ground-glass infiltrate usually corresponds to the irradiated volume.
• Findings at bronchoscopy are unremarkable (bronchial lavage may reveal lymphocytosis).
• Pulmonary embolism, infection, and progressive tumor must be ruled out. These conditions can co-exist with radiation pneumonitis.
• Response to corticosteroids is usually relatively rapid.
• Prevention is far more important than treatment. Patients must be selected carefully for thoracic radiation, and irradiated volumes must be limited.
• Corticosteroids are very useful in the management of acute and subacute pneumonitis (although they have no prophylactic or therapeutic value in the management of long-term radiation fibrosis).
• A pulmonologist should be consulted for all grade 3 cases and most grade 2 cases.
• Oxygen should be administered as indicated to prevent hypoxia.
• High doses of corticosteroids (60 mg/day of prednisone) should be administered with slow tapering (over several weeks to months) for severe grade 2 or any grade 3 radiation pneumonitis.
• If prolonged corticosteroid treatment is anticipated, prophylaxis against corticosteroid complications is needed, including gastrointestinal, infectious, and osteoporosis prophylaxis and dietary and pharmacologic management of hyperglycemia.
• Antibiotics, bronchodilators, diuretics, and anticoagulation should be used as indicated for co-existing cardiopulmonary illnesses.
• Usually, bleomycin, nitrosoureas, and mitomycin or combinations of several potentially pneumotoxic agents that on their own may only have modest pneumotoxicity (e.g., gemcitabine and weekly docetaxel) are risk factors.
• Bone marrow transplantation/high-dose chemotherapy with or without total body irradiation is a risk factor.
• Concurrent or recent thoracic radiation therapy is a risk factor.
• Poor baseline pulmonary function is a risk factor.
• Dyspnea and hypoxia are predominant, but a wide range of possible symptoms exists.
• Interstitial or ground-glass infiltrate usually is diffuse throughout both lungs and may be worse in the lower lobes.
• Findings at bronchoscopy are unremarkable (bronchial lavage may reveal lymphocytosis).
• Pulmonary embolism, infection, and progressive tumor must be ruled out and may co-exist with drug-induced lung injury.
• Injury is usually (but not universally) responsive to corticosteroids; it is less likely to respond well to steroids than radiation pneumonitis but more likely to respond well than late radiation fibrosis.
• When the diagnosis is suspected, the suspected causative agent should be discontinued.
• Consultation with a pulmonologist is necessary.
• Oxygen should be administered as indicated to prevent hypoxia (high fraction of inspired oxygen levels may be dangerous in bleomycin-related pneumonopathy).
• High doses of corticosteroids (≥60 mg/day of prednisone) with slow taper may be needed for severe grade 2 or any grade 3 pneumonitis.
• If prolonged corticosteroid treatment is anticipated, prophylaxis against corticosteroid complications entails gastrointestinal, infectious and osteoporosis prophylaxis and dietary or pharmacologic management of hyperglycemia.
• Antibiotics, bronchodilators, diuretics, and anticoagulation should be administered to manage co-existing cardiopulmonary illnesses.
Pulmonary Toxicity of Thoracic Radiation Therapy
Thoracic radiation is probably the most important cause of pulmonary toxicity in oncology. Lung toxicity from radiation is a clinically relevant issue for lymphoma, breast cancer, bone marrow transplantation (BMT), esophageal cancer, and lung cancer. Figure 58-1 illustrates two different cases of radiation pneumonitis and its sequelae.
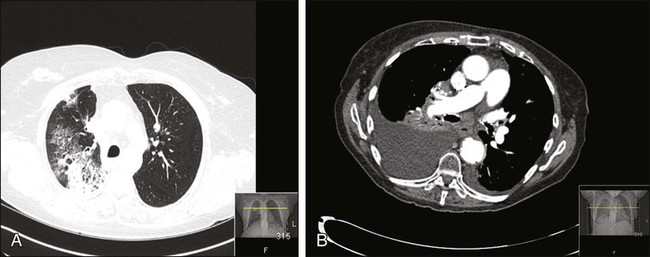
The mechanisms behind radiation-induced lung injury remain poorly understood despite decades of study. A detailed review of the histopathological and molecular events occurring in radiation pneumonopathy is beyond the scope of this chapter; several excellent reviews have been published in the past decade.3–3 Irradiation damages endothelial cells, epithelial cells (particularly surfactant-producing type II pneumocytes), and reticuloendothelial cells within the lung through several mechanisms, including apoptosis and induction of stress-response genes. It is now generally agreed that cytokines such as transforming growth factor beta (TGF-β) play a major role in promoting radiation pneumonopathy, including development of long-term fibrosis.4,5 It can be difficult histopathologically (or molecularly) to differentiate established radiation lung injury from other forms of end-stage lung disease, such as idiopathic pulmonary fibrosis, drug-induced injury, or even very advanced chronic obstructive pulmonary disease (COPD).
In most cases, radiation lung injury is confined to the regions of the lung within the radiation field or portal. This conventional wisdom has been challenged by several researchers who have found evidence of “out-of-field” radiation injury, which may be manifested in a syndrome similar to bronchiolitis obliterans with organizing pneumonia.6 In the most severe cases, diffuse acute respiratory distress syndrome can result from partial lung irradiation even with steroid treatment.7 Autoimmunity has been hypothesized as a mechanism of out-of-field radiation lung injury, with the possibility that localized lung damage triggers diffuse lymphocyte-mediated hypersensitivity against pulmonary self-antigens.8
Radiation lung injury may have any of a variety of clinical and radiographic presentations, but the hallmark symptom is dyspnea out of proportion to other findings. The most common imaging finding is an interstitial infiltrate corresponding to the radiation portals, but it is not unusual to find consolidation, nodularity, or even pleural effusions. The extent of radiographic findings does not necessarily correlate with the extent of symptoms or the patient’s clinical course.9 This problem can make the differential diagnosis among recurrent/progressive cancer, infection, and radiation lung injury extremely difficult, particularly in patients with lung cancer. Positron emission tomography with fluorodeoxyglucose may be helpful for differentiating recurrence from radiation toxicity, although intense radiation pneumonitis and even actively developing fibrosis causes elevated fluorodeoxyglucose uptake.10
Incidence of Radiation Lung Injury and Predictive Factors
The most important factor influencing development of clinically relevant radiation lung injury is the volume of lung irradiated; this issue is extensively discussed in the radiation oncology literature.11–15 In radiation oncology, the lung is considered a parallel-architecture organ, meaning that destruction of very small portions of it should not cause overall organ dysfunction. This belief is supported by the fact that wedge resection of a small portion of lung tissue or stereotactic radiosurgery to a very small lung tumor is well tolerated by most patients. In contrast, other organs, such as the spinal cord, are considered series-architecture organs, in which destruction of one region will lead to irreversible dysfunction downstream from that injury.16 The series-architecture model of radiation-induced toxicity does apply to other tissues within the lung, such as major bronchi and/or pulmonary vessels, where the result of injury to a small volume can be catastrophic (e.g., fistula and massive hemorrhage).17
The percentage of lung irradiated is often expressed by radiation oncologists as the “Vx,” where x = a certain dose in Gy. V20 indicates the percentage of a patient’s total lung volume that was irradiated to a dose of at least 20 Gy. Mean lung dose (expressed in Gy) is another parameter commonly used to express the extent to which a patient’s lung volume has been irradiated. Most clinical trials for locally advanced lung cancer require that the V20 be under a certain threshold, such as 35%. However, no single parameter can express the total radiation exposure, which is best described by a two-dimensional graphical curve called a dose-volume histogram (Fig. 58-2 provides an example).
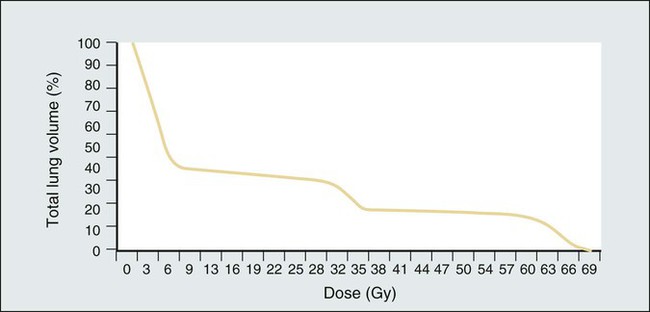
Irradiation of the entire lung volume (bilateral lungs) is uncommon today, except as part of total body irradiation for selected BMT conditioning regimens. As reviewed by Sampath and colleagues,18 the therapeutic index for whole-lung irradiation is extraordinarily narrow and highly dependent on total dose, fractionation, partial lung transmission shielding, and dose rate. A typical dose of 12 Gy total body irradiation has an approximately 11% rate of severe pneumonitis, compared with approximately 2% when lung transmission shielding is used to reduce the lung dose to 6 Gy.
At the other extreme, irradiation of small lung volumes, as with stereotactic body radiation therapy (SBRT, also known as radiosurgery) for early-stage non–small cell lung cancer (NSCLC) rarely results in high-grade RP, despite its use in a compromised patient population.21–21 The field of SBRT for lung cancer is evolving very quickly, and it is likely that with longer follow-up of patients, a greater understanding of the similarities and differences from conventional external beam radiation therapy (XRT) with regard to toxicity will be achieved.
In tangential irradiation of the breast (after lumpectomy) or chest wall (after mastectomy), the risk of clinically significant RP is approximately 1%, increasing to approximately 5% with the addition of irradiation of the regional (axillary/supraclavicular) nodes.22 An association between dose-volume irradiated and pneumonitis risk has also been shown in patients treated for esophageal carcinoma.23
Level I evidence of a strong relationship between pneumonopathy and the volume irradiated comes from a randomized trial from China.24 This study showed that the incidence of RP was significantly reduced from 29% to 17% with the use of “involved-field” radiotherapy, despite the use of higher tumor radiotherapy doses in the involved field arm.
Understanding the true incidence of radiation pneumonopathy is complicated by multiple factors, including ambiguities in scoring,26 uncertainties related to concurrent medical conditions, and limitations of the historical and current standards for defining and grading this illness.25 Kocak and colleagues25 found that 28% of their suspected RP had confounding medical conditions that made the clinical diagnosis uncertain. Yirmibesoglu and colleagues26 concluded that 48% of RP cases were “hard to score” (vs. “unambiguous”) because of confounding factors (e.g., tumor progression, acute exacerbation of COPD, and infection).
The traditional scoring system developed by the Radiation Therapy Oncology Group (RTOG), which dates back to the early 1970s, is shown in Table 58-1.27 This system is no longer generally used because of limitations such as an arbitrary cutoff of 90 days between acute and late radiation lung injury28; however, understanding of this system is important because numerous publications rely on it. Modern toxicity scoring is performed according to the Common Terminology Criteria (CTC), currently at version 4 (CTCv4).29 The CTCv4 definitions and grading for select pulmonary events are shown in Table 58-2. A comparison of CTCv3 or the old RTOG criteria to CTCv4 shows that numerous changes have been made to the scoring criteria in the pulmonology section. Separate scales are no longer used for early and late radiation therapy-related events.
Table 58-1
Traditional Scoring System for Radiation Lung Injury Based on the Radiation Therapy Oncology Group
Grade | (Acute) Radiation Pneumonopathy (Within 90 Days of Start of External Beam Radiation Therapy) | (Late) Radiation Pneumonopathy (>90 Days after Start of External Beam Radiation Therapy) |
1 | Mild, dry cough; dyspnea on significant exertion | Asymptomatic (e.g., slight radiographic findings only) or mild symptoms (e.g., dry cough) |
2 | Dyspnea on minimal exertion and/or persistent cough (requiring narcotics) | Moderate symptomatic fibrosis and/or pneumonitis (severe cough), fever, patchy radiographic appearances |
3 | Obvious severe radiation pneumonitis with dyspnea at rest and/or severe cough unresponsive to narcotics; oxygen and/or steroids are indicated | Severe symptomatic fibrosis/pneumonitis with dense radiographic changes |
3 | Life-threatening respiratory insufficiency requiring continuous oxygen/mechanical ventilation | Life-threatening respiratory insufficiency requiring continuous oxygen/mechanical ventilation |
5 | Death | Death |
Data from http://www.rtog.org/ResearchAssociates/AdverseEventReporting/AcuteRadiationMorbidityScoringCriteria.aspx and http://www.rtog.org/ResearchAssociates/AdverseEventReporting/RTOGEORTCLateRadiationMorbidityScoringSchema.aspx.
Table 58-2
Common Terminology Criteria Version 4* Select Common Terminology Criteria for Adverse Events Related to the Lung
Event | Grade 1† | Grade 2 | Grade 3 | Grade 4 |
Acute respiratory distress syndrome | N/A | N/A | Present with radiologic findings; intubation not required | Life-threatening respiratory or hemodynamic compromise; intubation required |
Atelectasis | Asymptomatic; clinical or diagnostic observations only; intervention not indicated | Symptomatic (e.g., dyspnea, cough), medical intervention indicated (e.g., chest physiotherapy, suctioning); bronchoscopic suctioning | Oxygen indicated; hospitalization or elective operative intervention indicated (e.g., stent, laser) | Life-threatening respiratory or hemodynamic compromise; intubation or urgent intervention indicated |
Carbon monoxide diffusion capacity (DLCO) | 3 to 5 units below LLN; for follow-up, a decrease of 3 to 5 units (mL/min/mm Hg) below the baseline value | 6 to 8 units below LLN; for follow-up, an asymptomatic decrease of >5 to 8 units (mL/min/mm Hg) below the baseline value | Asymptomatic decrease of >8 units drop; >5 units drop along with the presence of pulmonary symptoms (e.g., >grade 2 hypoxia or >grade 2 or higher dyspnea) | N/A |
Cough | Mild symptoms; nonprescription intervention indicated | Moderate symptoms, medical intervention indicated; limiting instrumental ADL | Severe symptoms; limiting self-care ADL | N/A |
Dyspnea | Shortness of breath with moderate exertion | Shortness of breath with minimal exertion; limiting instrumental ADL | Shortness of breath at rest; limiting self-care ADL | Life-threatening consequences; urgent intervention indicated |
FEV1 | 99% to 70% of predicted value | 60% to 69% of predicted value | 50% to 59% of predicted value | ≤49% of predicted value |
Hypoxia | N/A | Decreased O2 saturation with exercise (e.g., <88% pulse oximetry); intermittent supplemental oxygen | Decreased O2 saturation at rest (e.g., <88% pulse oxymetry or PaO2 ≤55 mm Hg) | Life-threatening airway compromise; urgent intervention indicated (e.g., tracheotomy or intubation) |
Pleural effusion (nonmalignant) | Asymptomatic; clinical or diagnostic observations only; intervention not indicated | Symptomatic, intervention indicated (e.g., diuretics or limited therapeutic thoracentesis) | Symptomatic with respiratory distress and hypoxia; surgical intervention including chest tube or pleurodesis indicated | Life-threatening respiratory or hemodynamic compromise; intubation or urgent intervention indicated |
Pneumonitis/pulmonary infiltrates | Asymptomatic; clinical or diagnostic observations only; intervention not indicated | Symptomatic; medical intervention indicated; limiting instrumental ADL | Severe symptoms; limiting self-care ADL; oxygen indicated | Life-threatening respiratory compromise; urgent intervention indicated (e.g., tracheotomy or intubation) |
Pneumothorax | Asymptomatic; clinical or diagnostic observations only; intervention not indicated | Symptomatic; intervention indicated (e.g., tube placement without sclerosis) or temporary chest tube | Sclerosis and/or operation indicated; hospitalization indicated | Life-threatening consequences; urgent intervention indicated |
Pulmonary fibrosis | Mild hypoxemia; radiologic pulmonary fibrosis <25% of lung volume | Moderate hypoxemia; evidence of pulmonary hypertension; radiographic pulmonary fibrosis 25%-50% | Severe hypoxemia; evidence of right-sided heart failure; radiographic pulmonary fibrosis >50%-75% | Life-threatening consequences (e.g., hemodynamic/pulmonary complications); intubation with ventilator support indicated; radiographic pulmonary fibrosis >75% with severe honeycombing |
*This is an abbreviated/abridged version of the Common Terminology Criteria, Version 4; the complete version can be found at http://evs.nci.nih.gov/ftp1/CTCAE/About.html.
†As with the older scoring systems, grade 5 (not shown) is death, and grade 0 is the absence of the particular toxicity. Note that for some adverse events, grades 1 to 2 (mild to moderate) might not be applicable (e.g., acute respiratory distress syndrome). Conversely, for some adverse events, grade 4 (life threatening) might not be applicable (e.g., cough).
Tucker et al.30 found that the scoring system used (CTCv2 vs. CTCv3 vs. RTOG) greatly affected the results of analyses of the risk of RP and the radiation dose-volume factors associated with RP.
Although radiation dose-volume parameters are the major predictor of radiation pneumonopathy, the addition of systemic therapy must also be considered. It appears that neoadjuvant chemotherapy before radiotherapy does not greatly affect the risk of radiation pneumonopathy. However, concurrent chemoradiotherapy probably increases the risk significantly; the maximum tolerated dose of localized radiotherapy with concurrent chemotherapy for NSCLC is generally considered to be 74 Gy,31 whereas the maximum tolerated dose for radiotherapy alone is usually considered to be higher than that.14
A combination of concurrent radiotherapy and gemcitabine appears to have a particularly high rate of radiation pneumonopathy, and extreme caution is advised for this combination (Table 58-3).32 Several other drugs, most notably the anthracyclines (e.g., doxorubicin), methotrexate, and bleomycin, should be considered contraindicated during thoracic radiotherapy.33 Concurrent thoracic radiotherapy plus a taxane (paclitaxel or docetaxel) is a commonly used and generally safe combination, although it has been suggested that the risk of radiation pneumonopathy might be slightly higher than expected from historical series based on older chemoradiotherapy regimens, particularly with weekly taxane schedules.36–36 It may be especially prudent to use three-dimensional radiotherapy techniques and to carefully analyze lung dose-volume histogram parameters in patients who are receiving a concurrent taxane.
Table 58-3
Select Large Clinical Studies of the Incidence and Severity of Radiation Pneumonopathy
Study | Patient Population | Rate of Grade 2 Pneumonopathy (%) | Rate of Grade 3+ Pneumonopathy (%) | Comments |
LUNG CANCER STUDIES | ||||
Byhardt et al.156 | 388 patients treated with conventional (large field) chemo-RT in the RTOG experience | NS | 17 | 2-dimensional, large-field XRT; grade 3 RP 20% with concurrent chemo-RT vs. 10% with sequential chemo-RT |
Keller et al.157 | 488 patients with moderate dose postoperative RT | NS | 3.5 | High performance status population of patients, moderate dose radiotherapy |
Turrisi et al.158 | 417 patients (limited stage small cell cancer) with concurrent chemo-RT | 12 | 5 | Small cell cancer (moderate dose RT); no difference between once daily and twice a day XRT |
Wang et al.13 | 223 patients treated with 3D XRT + concurrent chemo | NS | 32 | Higher rate than other studies may reflect the use of actuarial statistics and CTCv3 definition |
Hope et al.159 | 219 patients treated with high-dose 3D XRT | 12 | 11 | Strong association of RP with tumor location, lung DVH characteristics |
Palma et al.36 | 836 patients in a multicenter individual patient meta-analysis | 30 “symptomatic” pneumonitis | All patients received concurrent chemo—higher risk with taxane-based therapy | |
Movsas et al.62 | 205 patients in an RTOG randomized trial of chemo-RT ± Amifostine | 17 | 10 | No significant differences with/without amifostine |
STUDIES IN CANCERS OTHER THAN LUNG CANCER | ||||
Lind et al.160 | 613 patients with breast cancer | 2.4% of patients diagnosed with RP | RP was significantly more common with regional nodal irradiation | |
Yu et al.161 | 189 patients with breast cancer randomized between different chemo regimens prior to RT | 5 | <1 | High rate (24% to 39%) of radiographic changes but low rate of clinical RP |
Hughes-Davies et al.162 | 172 patients with bulky intrathoracic Hodgkin disease | 14 | NS | 1% fatal toxicity |
Koh et al.163 | 64 patients with Hodgkin disease | 3 | <1 | RP associated with larger lung volume irradiated |
Cooper et al.164 | 117 patients with esophageal cancer treated with chemo-RT | NS | 4 | |
Ishikura et al.165 | 139 patients with esophageal cancer treated with chemo-RT | 2 | 2 | In both of these studies, Radiation-induced cardiac complications and/or pleural effusions were more common than RP |
Kato et al.166 | 76 patients with esophageal cancer treated with chemoradiotherapy | NS | 4 |
Nontreatment factors that appear to be predictive of radiation lung injury have been studied. Not surprisingly, pretreatment performance status has been shown to correlate with development of clinical radiation pneumonopathy.37 Underlying pretreatment pulmonary function is probably an important factor as well,38 although study results have been inconsistent,39,40 perhaps because in many cases, poor pretreatment pulmonary function is due to effects of the cancer itself; an effective treatment (such as radiotherapy) may then substantially improve rather than harm pulmonary function. Other possible risk factors for toxicity include prior thoracic irradiation, volume loss due to lung collapse, forced expiratory volume in one second <2 L for severe (grade ≥3) RP,37 older age,33 female gender,21,33 never or current nonsmokers33,41,42 and poor pretreatment performance status, and pretreatment COPD in patients with lung cancer.43 Risk factors significantly associated with RP in patients with Hodgkin lymphoma were minimum lethal dose ≥13.5 Gy and V20 ≥33.5% when initial combined modality therapy consisted of Adriamycin (doxorubicin), bleomycin, vinblastine, and dacarbazine and mediastinal radiotherapy.44 Use of angiotensin-converting enzyme (ACE) inhibitors may be associated with a lower risk of RP,45 although this is controversial and not confirmed by any prospective data.
Risk of radiation-induced lung injury may be higher in patients with certain genetic variations. Studies have explored ataxia-telangiectasia mutated (ATM) gene46 or TGFβ1 gene polymorphisms47 as they relate to radiation lung injury, although this parameter is not yet used clinically.
Diagnosis and Management of (Acute and Subacute) Radiation Pneumonitis
With modern, three-dimensional conformal, multifield radiation therapy, SBRT, or intensity-modulated radiation therapy, it is not possible to simply look for pathognomonic rectangular infiltrates on a chest radiograph to confirm RP. A patient who has undergone radiation treatment (particularly if a large volume of lung was irradiated to a dose >20 Gy) and has symptoms should undergo computed tomography (CT) scanning, in some cases with high-resolution CT imaging. CT angiography may also be indicated to rule out a pulmonary embolism. Imaging will typically reveal an interstitial infiltrate and/or ground-glass appearance, which can be very difficult to distinguish from an infection or tumor-related changes. Suspected moderate to severe radiation pneumonopathy should be evaluated by a pulmonologist for consideration of bronchoscopy to rule out infection, particularly if fever is present.48
Pulse oximetry or arterial blood gas testing should be performed to assess the need for supplemental oxygen. Pulmonary function testing, especially the diffusion capacity of the lung for carbon monoxide (DLCO) test, is useful as part of the diagnostic workup, in particular to assess the severity of gas-exchange dysfunction, although pulmonary function tests are not strongly correlated to clinical symptoms of radiation-induced lung disease.33 Spirometric parameters of pulmonary function are frequently reversible after radiation pneumonopathy, whereas DLCO abnormalities are less likely to improve.49 The tests and procedures to be considered in the evaluation of patients with cancer who have suspected pneumonopathy are summarized in Table 58-4. This workup should be considered appropriate for either suspected radiation-related or chemotherapy-related pneumonopathy.
Table 58-4
Evaluation of Patients with Cancer Who Have Pulmonary Symptoms (Especially Dyspnea) and Suspected Radiation or Chemotherapy Pneumonopathy
Study* | Rationale |
BASIC, MINIMAL WORKUP | |
CT of chest, preferably both with and without contrast | Assess extent/appearance/location of infiltrates/effusions; correlate with radiation therapy and/or surgical data; rule out recurrent/progressive cancer and/or other etiologies for dyspnea |
Pulse oximetry | Assess degree of hypoxia and possible need for supplemental oxygen |
Pulmonary function testing (spirometry and DLCO) | Assess extent and type of pulmonary function (radiation/drug pneumonitis is a restrictive pattern, with DLCO often markedly abnormal compared with baseline levels) |
CBC/differential; chemistry panel | Rule out leukocytosis and/or leukopenia (possible signs of infection), anemia, hepatic and/or renal insufficiency (all factors that can lead to pulmonary distress) |
EXTENDED WORKUP† | |
High-resolution pulmonary-embolus protocol CT scan, V/Q scan, and/or pulmonary angiogram | Rule out pulmonary embolism. |
Electrocardiogram | Rule out cardiac ischemia and/or dysrhythmia |
Blood test for B-type natriuretic peptide | Rule out CHF—can occur concurrently with pneumonopathy |
Arterial blood gas testing | More accurate measure of oxygenation than pulse oximetry; measurement of pH and CO2 levels |
Blood cultures | Rule out sepsis and/or endocarditis |
Bronchoscopy | Rule out infection (particularly if atypical infection is suspected) and assess for possible recurrent cancer |
PET/CT scan | Assessment of status of the cancer; possible role as adjuvant form of imaging the extent of lung injury |
Open lung biopsy (e.g., thoracoscopic biopsy) | Definitive diagnosis but high-risk procedure; avoid if diagnosis is highly likely and response to steroids is good |
*Notice that chest radiograph is not a sufficiently sensitive or specific test to warrant inclusion in this table.
†An extended workup should be performed if the diagnosis is in question and/or if there is a possibility of co-existing cardiorespiratory problems.
If the clinical manifestations and test results are consistent with grade 2 or greater RP, administration of corticosteroids should be instituted in most cases. Controlled randomized trials of corticosteroids for RP have not been conducted with human subjects. However, the efficacy of corticosteroids has been well established in nonrandomized clinical studies50 and in preclinical models.51 No single “standard” dose schedule exists for steroid therapy for persons with RP; the exact schedule must be tailored to the individual patient. In general, for severe (grade 3) RP, prednisone, approximately 1 mg/kg/day, is indicated for 2 weeks. Most clinicians suggest starting with 60 mg/day prednisone, then tapering as symptoms improve. Brief hospitalization for intravenous administration of steroids may be indicated. Early onset of RP after the completion of radiotherapy may predict a more virulent course and therefore might require a more aggressive management approach.7 Moderate RP (grade 2) may be effectively managed with somewhat lower initial doses of steroids (e.g., 0.5 to 0.75 mg/kg/day of prednisone); however, the patient must be evaluated frequently to ascertain that his or her condition is not progressing to grade 3 or worse RP. After several weeks, the dose should be tapered gently, by approximately 10 mg every 2 weeks. It is not unusual for patients to have a symptomatic relapse in the setting of steroid taper.52 If relapse occurs, it is important to rule out concomitant infection. If the diagnosis of recurrent RP is confirmed, the steroid dose should be increased and titrated accordingly.
It is uncertain whether a low-dose “prophylactic” antibiotic should be prescribed. In any given patient with interstitial pneumonitis after thoracic radiotherapy, it can be very difficult to entirely rule out a concomitant infection, particularly if bronchoscopy or another invasive diagnostic procedure was not performed.48 In light of the profound lymphopenia that many patients have after chemoradiation therapy and steroid treatment (as with typical concurrent paclitaxel-radiation therapy regimens), it might be appropriate to prescribe an every-other-day dose of trimethoprim-sulfamethoxazole (Bactrim) when starting high-dose steroids.53,54 If findings of a chest CT image suggest the presence of co-existent active infection, broader and more intense antibiotics are indicated. The patient should undergo bronchoscopy if no improvement occurs after several days of steroid and antibiotic therapy.
The prognosis of grade 1 to 2 RP is relatively good with meticulous supportive care and the use of steroids as needed. Grade 3 RP, however, at least in patients with lung cancer, has a much worse prognosis.7,13 It is uncertain whether this worse prognosis is the direct result of RP or coexisting problems, including tumor recurrence and infection.
Management of radiation pneumonopathy that is refractory to steroids and/or that occurs in a patient who has severe contraindications to steroids is uncertain. The literature includes case reports of the use of antirheumatic medications such as cyclosporin,55,56 but little scientific evidence supports their use.
Trials of Prevention or Management of Radiation Pulmonary Fibrosis
Until the 1990s, radiation-induced lung injury was considered inevitable and untreatable, the result of delayed mitotic death of parenchymal or vascular cells.57 As understanding of the mechanisms of lung injury has improved, prophylactic treatments have been studied, but few pharmacologic agents are approved for prevention of radiation-induced lung injury. Three main classes of radiation countermeasure agents are (1) radioprotective agents, given before irradiation; (2) radiation mitigators, given after irradiation but before expression of tissue damage; and (3) therapeutic agents, given after irradiation as palliation or support.58
Only one radioprotective agent, a synthetic thiol-reducing agent called amifostine, has been approved by the U.S. Food and Drug Administration to protect normal tissues from radiation during treatment, and this was to prevent renal and salivary gland damage, not specifically for pulmonary toxicity.58 The efficacy of amifostine to prevent RP is uncertain; results of randomized trials have been mixed.61–61 A relatively large confirmatory study found no significant differences.62 Amifostine is logistically and financially difficult to deliver and is associated with its own toxicities (e.g., nausea, fatigue, skin rash, hypotension, and syncope). Its effectiveness is likely highly correlated with dose and schedule, and amifostine has no known role or rationale as a treatment once radiation has been completed.
Other nonspecific antioxidants have been studied as means of decreasing radiation fibrosis, although they are not necessarily specific for the lung.63–66 Multiple dietary69–69 plant70 antioxidants have been studied in vitro and in animal models to prevent radiation lung damage. Gene therapy to deliver antioxidants to the lung is under study. Researchers from the University of Pittsburgh have developed a system in which the gene for the potent antioxidant enzyme manganese superoxide dismutase can be transfected into a plasmid and administered by inhalation.64 This approach, which is still highly experimental, might offer a preventive and/or therapeutic measure.
The use of angiotensin-converting enzyme (ACE) inhibitors, particularly captopril, showed promise in preclinical animal studies.71 In one retrospective analysis of patients with stage I-III lung cancer who were treated with definitive radiation, the incidental concurrent use of ACE inhibitors was associated with less radiation-induced pulmonary toxicity.72 This finding has not been validated in a prospective or controlled trial.73 The mechanism of efficacy is unclear; it might be related to the beneficial vasodilatory effects of these drugs on small blood vessels or attributed to antioxidant activity.
Two modest-sized randomized trials from Turkey suggested that pentoxifylline (PTX) may help prevent acute and late lung tissue damage.74,75 In one study, PTX or placebo was given at a dose of 400 mg three times a day throughout radiotherapy; results showed significantly better DLCO and perfusion scan results at 3- and 6-month follow-up among patients randomly assigned to PTX. In the other study, patients were randomized to control versus PTX (400 mg three times a day during radiotherapy and 400 mg daily after radiotherapy for 3 months) plus vitamin E (300 mg twice daily during radiotherapy and 300 mg daily after radiotherapy). Results showed less RP in the arm randomized to PTX/vitamin E. The potential mechanism by which PTX ameliorates RP is unknown, and these studies have not been validated in other randomized trials. No meaningful data are available regarding the efficacy of PTX as a treatment (as opposed to preventative agent) for radiation pneumonopathy.
Increased understanding of the cytokine-based mechanisms of radiation lung injury may offer opportunities for intervention.65,76 Because TGF-β is thought to be the dominant profibrotic cytokine and perhaps even a cause of RP (particularly late radiation pulmonary fibrosis), attempts are underway to develop molecules with anti-TGF-β activity. At this time, no molecularly targeted treatments for established radiation lung injury (RP or radiation pulmonary fibrosis) are clinically available. Steroids (for RP at least) remain the only known treatment.
Pulmonary Toxicity of Systemic Anticancer Therapies
Many chemotherapy drugs can cause pulmonary toxicity, with the incidence ranging from less than 1% to more than 30%. The drugs that are most associated with pulmonary toxicity are bleomycin, methotrexate, cytosine arabinoside, mitomycin, and the nitrosoureas (especially carmustine [BCNU]). Table 58-5 provides a broad categorization of anticancer therapies into high, moderate, and low risks of pneumotoxicity, although any individual patient may experience severe lung problems from any agent.
Table 58-5
Anticancer Therapies Categorized by Risk of Pneumotoxicity
Therapy | Examples |
Highly pneumotoxic agents (risk of pulmonary SAE probably >5%) | Bleomycin; BCNU; mitomycin; interleukins; bone marrow transplantation (with or without TBI); large-volume thoracic radiation therapy (e.g., T4N3 lung cancer); surgical resection for lung cancer |
Moderately pneumotoxic agents (risk of pulmonary SAE probably 1%-5%) | Methotrexate; busulfan; melphalan; CCNU/MeCCNU; cyclophosphamide; ifosfamide; fludarabine; gemcitabine; paclitaxel/docetaxel; small-volume thoracic radiation therapy (e.g., breast cancer); non–lung cancer oncologic surgery |
Uncommon pneumotoxic agents (risk of pulmonary SAE probably <1%) | 5-FU; capecitabine; cisplatin/carboplatin; doxorubicin; actinomycin-D; etoposide; topotecan/irinotecan; vincristine/vinblastine; vinorelbine; temozolomide; tamoxifen; aromatase inhibitors for breast cancer; hormonal therapies for prostate cancer; steroids |
Unlike thoracic radiation therapy, which usually affects only the portion of lung within the radiation field, systemic agents often cause diffuse pneumonopathy. Although chemotherapy-induced lung injury is relatively rare in comparison with radiation pneumonopathy, it can be extremely intense and can even have a higher fatality rate than RP. As with RP, corticosteroids are commonly used and may be effective, particularly in early stages of injury. It is particularly important to rule out alternative and concurrent diagnoses (see Table 58-4). Unlike radiation injury, drug-induced lung toxicity can occur in a time frame during which it is possible to discontinue the offending agent. A review of some of the systemic agents associated with pulmonary toxicity follows.
Bleomycin
Bleomycin is the chemotherapy drug most commonly associated with lung damage. The reported incidence ranges from 3% to 40%.77,78 This drug is used predominantly in the management of Hodgkin disease and germ cell tumors, cancers that occur mainly in younger patients with less underlying pulmonary comorbidity than patients with lung cancer. Bleomycin pneumonopathy and RP have a number of similarities, including two patterns of disease (pneumonitis and fibrosis). As with radiation, the clinical manifestations of bleomycin lung toxicity usually occur weeks to months after the initiation of treatment. The infiltrates can be diffuse or limited to basilar and subpleural aspects of the lungs.79 A nodular pattern occasionally occurs, mimicking cancer progression. Imaging other than CT scanning is investigational, although there are reports of abnormal fluorodeoxyglucose-positron emission tomography scan results in persons with bleomycin pneumonopathy.80
As in radiation pneumonopathy, dyspnea is the primary symptom of bleomycin lung toxicity, although, as is the case with radiotherapy, other symptoms such as cough and fever often occur as well. Pulmonary function testing shows a restrictive pattern; DLCO is frequently abnormal. DLCO should be measured before bleomycin is started and periodically between cycles. A significant decrease in DLCO should prompt consideration of discontinuation of this drug. Although no consensus exists on what represents a “significant decrease” in DLCO, some investigators have conservatively used a criterion of a 20% decrease to warrant discontinuation of bleomycin.81
An association appears to exist between cumulative bleomycin dose and risk of pneumonopathy. Again, no consensus exists regarding the absolute maximum cumulative bleomycin dose for an individual patient; values of 300 to 400 mg maximum have been suggested.77 (A typical cumulative dose of bleomycin from six cycles of Adriamycin [doxorubicin], bleomycin, vinblastine, and dacarbazine [used to treat persons with Hodgkin disease] is approximately 120 mg/m2). Again, however, it must be stressed that occasionally life-threatening or even fatal pneumonopathy can occur with cumulative bleomycin doses less than 100 mg. Another important predictive factor (in addition to age and dose) for bleomycin pneumonopathy appears to be renal insufficiency,77,82 which is particularly concerning in patients who are receiving nephrotoxic agents such as cisplatin. Other possible risk factors may include concomitant use of a colony-stimulating factor,83 combined treatment with radiotherapy, and concomitant delivery with gemcitabine.84,85 Exposure to high oxygen concentrations as part of operative anesthesia has been associated with potentiation of bleomycin pneumonopathy, particularly in animal models,86 and in some cases led to acute respiratory distress syndrome.87,88
Extreme caution is advised when supplemental oxygen is being electively prescribed for patients treated with bleomycin, especially those who are currently undergoing or recently finished bleomycin (within less than 12 months) and/or have documented bleomycin pneumonopathy.89
Bleomycin pneumonitis is rarely fatal; one study estimated a lethality risk of about 3%, with a suggestion that older age was associated with a higher risk for death.90 Most patients achieve complete or near-complete recovery.90–94 Corticosteroids may have some benefit,77,91,95 although the data are not as clear as for radiation pneumonitis. Some cases of steroid-responsive bleomycin pneumonitis/fibrosis may represent early hypersensitivity, bronchiolitis obliterans with organizing pneumonia–like events. After bleomycin chemotherapy, cancer survivors may have significant declines in pulmonary function for approximately 6 months, but by 2 years after chemotherapy, few have significant respiratory dysfunction.
Antimetabolites
Methotrexate, which is commonly used in therapy for rheumatoid arthritis and for cancer, is the antimetabolite drug that is most commonly linked to pulmonary injury. It can cause interstitial pneumonitis similar to that caused by bleomycin and other drugs.96–99 Steroid therapy may be indicated for severe cases.
Gemcitabine has become one of the most commonly used chemotherapy drugs for solid tumors, including lung cancer. When gemcitabine is used as a single agent in previously untreated patients with cancer, the risk of significant pulmonary toxicity is small, although it is associated with an occasional severe respiratory insufficiency that may be related to capillary leak syndrome.100 However, increasing data suggest that combinations of gemcitabine with other agents may severely potentiate those agents’ pneumonopathy (see the discussion in previous sections regarding thoracic radiotherapy and bleomycin)—in particular, the combination of gemcitabine with taxanes.101,102 Steroid therapy can be a useful adjunct to supportive treatment.92 Gemcitabine may also be associated with radiation recall pneumonitis, even a year or more after thoracic radiation therapy. Gemcitabine radiation recall reaction appears to be more likely to affect parenchymal organs such as the lung than the more common dermatologic radiation recall reactions associated with other chemotherapeutic agents.93
Fludarabine is now commonly used to manage hematologic malignant disease, including chronic lymphocytic leukemia, and, like other antimetabolite drugs, it is relatively well tolerated. In approximately 8% of cases, fludarabine causes pulmonary toxicity characterized by fever and interstitial pneumonitis.94,101 Although this syndrome is probably steroid responsive,103 it is critical to rule out opportunistic infection given the patient population usually treated with this drug.
Alkylators and Nitrosoureas
Alkylators and nitrosoureas are frequent components of conditioning regimens for BMT, a procedure that is associated with a high rate of pulmonary toxicity. It is difficult to isolate the effect of these drugs in this setting, in which many other insults to the lung often occur, but it is likely that these drugs contribute to pulmonary toxicity, particularly at BMT dose intensity. Results suggest that pulmonary toxicity from BMT with regimens including whole-body irradiation is similar to that from BMT with regimens that do not include whole-body irradiation,104,105 which underscores the relationship between high-dose alkylator therapy and pneumonopathy.
In the non-BMT setting, classic alkylators, such as cyclophosphamide, ifosfamide, and melphalan, have occasionally been associated with interstitial pneumonitis and acute or subacute dyspnea that may be steroid responsive.106,107 However, these drugs have caused long-term pulmonary fibrosis, even several decades after treatment.106
For nitrosoureas such as BCNU, pulmonary injury is considered a dose-limiting toxicity and has been well studied.108 The predominant problem with these agents is pulmonary fibrosis, which appears to be chronic and dependent on dose and patient age.111–111 In one study, BCNU pulmonary fibrosis in patients treated for glioma was rare at cumulative doses less than 960 mg/m2.111 The association between other nitrosoureas and pulmonary toxicity is not commonly reported but does occasionally occur.112,113 The pulmonary fibrosis that is caused by nitrosoureas does not appear to be steroid responsive. The unpredictable and untreatable nature of nitrosourea-induced pneumonopathy has recently limited the use of these drugs in several settings.113
Anthracyclines and Other Antitumor Agents
Essentially every cytotoxic chemotherapy has been associated with pulmonary toxicity. Case reports and/or literature reviews can be identified even for drugs that are rarely associated with pneumonopathy, such as vinorelbine,114 paclitaxel,115 and oxaliplatin.116 Response to steroids has been variable. Several other agents, however, deserve special mention.
The antibiotic chemotherapy drug mitomycin is well known as a potentially pneumotoxic agent that can cause noncardiogenic pulmonary edema, pneumonitis, and pleural effusion. Toxicity is difficult to predict and not clearly dose-related but is estimated to occur in 5% of patients or more.117,118 In a prospective study of patients treated with mitomycin, 28% of patients tested had significant (>20%) declines in DLCO, and 5% had grade 3+ lung toxicity.118 Mitomycin-induced pulmonary toxicity appears to be steroid responsive.119
Anthracyclines are vital drugs for the treatment of breast cancer among other malignancies; although they are associated with significant cardiac risk (which can mimic pulmonary toxicity), they rarely independently cause pneumonopathy. However, the combination of anthracyclines such as doxorubicin with thoracic radiotherapy can be highly toxic. For example, a randomized trial of concurrent versus sequential chemoradiotherapy (including doxorubicin) for small cell lung carcinoma was closed early because six fatal cases of pneumonopathy developed among 82 patients who were treated concurrently.120 There may be a risk of radiation recall-like reactions in patients receiving doxorubicin after high-dose thoracic radiation therapy, at least in the vulnerable lung cancer population.121
Like the anthracyclines, the taxanes (paclitaxel and docetaxel) are widely used solid tumor antineoplastic drugs. Docetaxel seems to be more associated with pneumonopathy than does paclitaxel. The risk of pneumonitis appears to be schedule-related, with a significantly higher risk with the use of weekly (versus every 3 weeks) docetaxel.107 This finding contrasts with the nonpulmonary toxicities of this drug, which appear to be lessened with the weekly schedule.122 Docetaxel has also been associated with enhancement of radiation pneumonopathy in patients with lung cancer.123 The combination of paclitaxel plus radiotherapy after anthracycline-based chemotherapy might not be feasible in patients with breast cancer because of pulmonary toxicity124 despite the relatively modest amounts of lung in the irradiated field. As was noted previously, the combination of taxanes with gemcitabine appears to result in a significant risk of pneumonopathy93 and should be used with extreme caution in patients with underlying lung disease.
Biological Agents
Immunomodulatory anticancer agents are one of the oldest classes of biological therapies. Pneumonopathy associated with interleukins or interferons occasionally occurs,125 as well as a syndrome of noncardiogenic pulmonary edema, which differs from typical drug-induced interstitial pneumonitis.126 Noncardiogenic pulmonary edema is generally reversible with steroids and supportive care and rarely progresses to fibrosis.
Several randomized trials suggested that the addition of interferon to conventional lung cancer therapy increased the risk of pneumonopathy.127,128
Targeted therapies against signal transduction pathways have become a heralded advance in several hematologic and solid tumor types. Most of these drugs have not been studied extensively enough to fully characterize their pulmonary risks. An overview of incidence and specific patterns of pulmonary toxicity seen with molecularly targeted agents used for cancer therapy follows (see Table 58-6).
Table 58-6
Management of Temsirolimus-Related Interstitial Lung Disease
Type of ILD | Treatment |
ILD in asymptomatic patients | No specific therapy or drug interruption required in asymptomatic patients with only radiologic changes |
ILD in patients with mild symptoms | Consider interrupting therapy until symptoms improve; will need monitoring of PFTs and chest CT |
ILD in patients with underlying lung disease or moderate symptoms/PFT/CT abnormalities | Consider interrupting therapy until symptoms improve; the use of corticosteroids may be indicated; will need frequent monitoring of PFTs and chest CT |
ILD in patients with severe or progressive symptoms in conjunction with a decrease in DLCO on PFT | Discontinue temsirolimus; consider high-dose glucocorticoids |
Antiepidermal Growth Factor Receptor Tyrosine Kinase Inhibitors
Gefitinib and erlotinib are the oral tyrosine kinase inhibitors (TKIs) (against the intracellular portion of the epidermal growth factor receptor) that have been well studied and are primarily used in the treatment of NSCLC (Fig. 58-3). These agents are associated with an uncommon but sometimes very severe syndrome of interstitial pneumonitis. This pneumonitis appears to be more common in the Japanese population, with a reported incidence of about 3%.129 Common radiographic appearance in patients with confirmed gefitinib-induced pneumonitis are nonspecific ground-glass opacities, multifocal areas of airspace consolidation, and patchy ground-glass opacities with septal thickening.129 In the TRIBUTE trial (paclitaxel and carboplatin with or without concurrent erlotinib) in persons with advanced NSCLC, erlotinib was not associated with an increased risk of serious pulmonary adverse events.130 However, a blinded independent review of interstitial lung disease associated with death in this trial identified fatal interstitial lung disease in 0.6% of cases treated with the combination of erlotinib plus chemotherapy.131
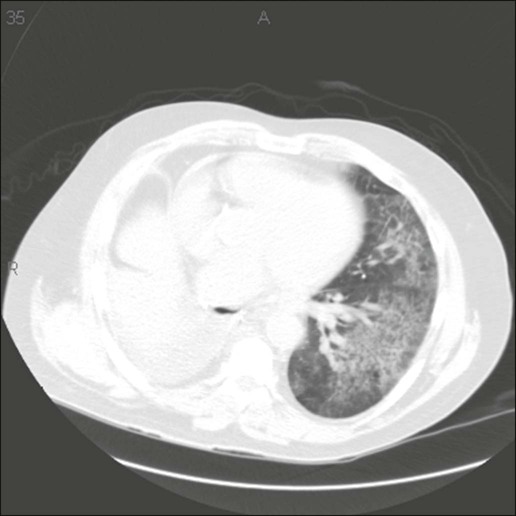
Other Tyrosine Kinase Inhibitors
The risk of pneumonopathy from targeted therapies does not appear to be limited to the lung cancer population. Imatinib, an inhibitor of KIT and BCR-ABL tyrosine kinases that is used for several types of hematologic malignancies and gastrointestinal stromal tumors, has been associated with interstitial pneumonitis as well.132 Dasatinib, a second-generation TKI that inhibits BCR-ABL and SRC kinase, is used for newly diagnosed and imatinib-resistant chronic myelogenous leukemia. Exudative pleural effusions developed in up to 35% of patients treated with dasatinib in phase 1/2 studies.133 Dasatinib can cause pulmonary hypertension (PH) by a direct and specific effect on pulmonary vessels; in a review of French PH registry, nine cases of dasatinib-induced PH were identified.134 Crizotinib is another TKI that is approved for the treatment of advanced or metastatic NSCLC and contains a characteristic EML4-ALK fusion oncogene. Severe, life-threatening pneumonitis occurred in 4 of 255 patients (1.6%) treated with crizotinib in a phase 2 clinical trial.135 All the cases developed within 2 months of the initiation of treatment. Sorafenib and sunitinib are small molecule multikinase inhibitors of vascular endothelial growth factor and platelet derived growth factor. Both are currently approved for treatment of advanced renal cell cancer (RCC). Interstitial pneumonitis has been reported with sorafenib, which completely resolved upon discontinuation of the drug.136 Radiation recall pneumonitis was reported in one case of sunitinib 3 weeks after completion of chest radiotherapy. At this time, there are no specific contraindications to the use of signal transduction inhibitory agents based on pulmonary risk; however, current protocols call for the drugs to be discontinued if thoracic imaging reveals evidence of interstitial pneumonitis.
Monoclonal Antibodies
Many newer molecularly targeted cancer drugs are antibodies against growth factor ligands or cell surface receptors. Bevacizumab is the prototype antibody against vascular endothelial growth factor that is currently approved for use in persons with metastatic colorectal cancer, NSCLC, RCC, and glioblastoma multiforme. Bevacizumab has been associated with life-threatening pulmonary hemorrhage,137 which seems to be more associated with centrally located squamous cell lung carcinomas and might be related to rapid tumor response, resulting in bronchovascular fistula. Further studies and means of predicting who is at risk for this devastating complication and how to avoid it are necessary to advance the use of this class of agents.
Cetuximab and panitumumab are monoclonal antibodies that target epidermal growth factor receptor and have activity against metastatic colorectal cancer and squamous cell carcinoma of the head and neck. Cetuximab infusion can cause severe hypersensitivity reactions, including bronchospasms in up to 3% of patients.138 Both cetuximab and panitumumab have rarely been associated with pulmonary fibrosis.139,140 Rituximab and ofatumumab are anti-CD20 monoclonal antibodies approved for the treatment of CD20-expressing hematologic malignancies. Case reports of fatal interstitial pneumonitis have been reported with both agents.141,142 Rituximab infusion can cause bronchospasm similar to that seen with other chimeric antibodies (i.e., cetuximab), which typically resolves upon stopping or slowing the infusion rate. Fatal pulmonary interstitial fibrosis has also been reported after initiation of weekly rituximab for follicular lymphoma.143 Alemtuzumab is a humanized anti-CD52 antibody that is approved for refractory chronic lymphocytic leukemia. Infusion-related pulmonary symptoms of dyspnea and hypoxia have been reported in up to 28% of patients.144 Diffuse alveolar hemorrhage has been reported with alemtuzumab in the postrenal transplant setting.145
Ipilimumab is a recombinant, human monoclonal antibody that binds to the cytotoxic T-lymphocyte–associated antigen 4 and blocks interaction with its ligands, CD80/CD86. Inhibition of cytotoxic T-lymphocyte–associated antigen 4 augments T-cell activation and mediates antitumor immune responses. Ipilimumab was recently approved for treatment of advanced melanoma. Severe immune-mediated adverse reactions including pneumonitis have been reported.146 Although pneumonitis responds to a prolonged course of corticosteroids, permanent discontinuation of ipilimumab treatment is recommended.
Inhibitors of Mammalian Target of Rapamycin
Mammalian target of rapamycin (mTOR) is a serine threonine kinase that regulates cell growth, proliferation, and apoptosis. Noninfectious pneumonitis, a class effect of rapamycin derivatives, develops in patients treated with mTOR inhibitors such as everolimus and temsirolimus. Everolimus is approved for the treatment of RCC, neuroendocrine tumors of pancreatic origin, and subependymal giant cell astrocytoma. Temsirolimus is approved for the treatment of RCC. In clinical studies with these agents, some pneumonitis cases have been severe and occasionally fatal. Radiographic findings consistent with drug-induced pneumonitis were detected in 36% of patients receiving temsirolimus for advanced neuroendocrine tumors and endometrial carcinoma.147 Only one half of the patients with radiographic changes had clinical symptoms of pneumonitis, and drug treatment was continued in some cases without worsening of the pneumonitis.147 Guidelines have been suggested for management of temsirolimus-induced pneumonitis (see Table 58-7).
Table 58-7
Pneumotoxicity Associated with Use of Targeted Agents
Agent | Pneumotoxicity |
Gefitinib, erlotinib | Acute interstitial lung disease |
Imatinib, dasatinib | Pneumonitis, pleural effusions, pulmonary hypertension |
Crizotinib | Acute interstitial pneumonitis |
Sorafenib, sunitinib | Pneumonitis, radiation recall pneumonitis |
Bevacizumab | Pulmonary embolus, pulmonary hemorrhage |
Cetuximab, panitumumab | Bronchospasm, bronchiolitis, pulmonary fibrosis |
Rituximab, ofatumumab | Pulmonary interstitial fibrosis, infusion-related bronchospasm, interstitial pneumonitis |
Alemtuzumab | Dyspnea, hypoxia, pulmonary hemorrhage (after solid organ transplant) |
Ipilimumab | Pneumonitis |
Everolimus, temsirolimus | Interstitial pneumonitis |
Thalidomide/lenalidomide | Pulmonary embolism, pneumonitis, organizing pneumonia, eosinophilic pneumonia |
Bortezomib | Bronchiolitis obliterans organizing pneumonia |
In a placebo-controlled randomized trial of everolimus for advanced RCC, clinical pneumonitis was suspected in 37 (13.5%) of the 274 patients who received this drug.148 Nine patients (3.3%) had grade 1 pneumonitis (asymptomatic), 18 (6.6%) had grade 2 pneumonitis (not interfering with daily living), and 10 (3.6%) had grade 3 pneumonitis (interfering with daily living or oxygen dependent). Half of the patients with grade 3 pneumonitis had baseline radiologic evidence of pneumonitis before starting treatment with everolimus. The common radiographic features of pneumonitis were focal areas of consolidation at the lung bases or diffuse ground-glass opacities. Resolution of pneumonitis occurred after dose reduction for 20 patients and treatment discontinuation for 10 patients.148
The etiology of the pneumonitis related to mTOR inhibitors remains unclear. Clinicopathological patterns include interstitial pneumonitis with or without fibrosis, bronchiolitis obliterans organizing pneumonia, or alveolar hemorrhage.148 Immune-mediated mechanisms of pneumonitis are supported by lung biopsies, bronchoalveolar lavage findings, and the observed clinical response to corticosteroids. The bronchoalveolar lavage fluid and lung biopsy specimens obtained from four patients in whom grade 3 interstitial lung disease developed while they were being treated with everolimus for advanced RCC showed lymphocytosis, interstitial lymphocytic infiltration, and septal thickening of alveolar walls.149
Other Agents: Thalidomide and Lenalidomide
Thalidomide and lenalidomide are used in the treatment of multiple myeloma and share a complex mechanism of action that includes immunomodulatory and antiangiogenic effects. The most common pulmonary adverse effect of thalidomide and lenalidomide is pulmonary embolism. Venous thromboembolic disease occurs in as many as 12% of patients taking these agents and appears to be higher when the drug is combined with dexamethasone or other chemotherapeutic agents.150 Dyspnea in association with thalidomide treatment has been reported in 4% to 54% of patients.151 Rare adverse effects with these agents include interstitial pneumonitis, organizing pneumonia, and pleural effusion that typically resolve with steroids and discontinuation of the drug.152,153
Bortezomib
Bortezomib, a proteasome inhibitor, is currently approved as front-line therapy for multiple myeloma. Bortezomib can cause serious lung injury, and its incidence might vary among different ethnicities. In a series of 13 Japanese patients treated with bortezomib for multiple myeloma, severe pulmonary toxicity developed in four patients and two died of respiratory failure without progression of underlying disease.154 Autopsy results from a patient in one of the cases showed acute and organizing diffuse alveolar damage. Another case report described a rapidly progressive bronchiolitis obliterans organizing pneumonia resulting in respiratory failure after treatment with bortezomib. This patient responded rapidly to high-dose glucocorticoids.155