CHAPTER 126 Medulloblastoma
Medulloblastoma is the most common primary central nervous system tumor in children. Although major advances in diagnosis and treatment have led to improved outcomes in children with medulloblastoma since Cushing first reported his experience in 1930,1 significant challenges remain. This chapter summarizes these challenges in the context of what is currently known about the clinical and pathologic features of medulloblastoma.
Terminology and Classification
The term medulloblastoma cerebelli was first introduced by Bailey and Cushing in 1925 to refer to a distinct, highly malignant, small cell tumor of the midline cerebellum.2 The terminology and classification of medulloblastoma remained unchallenged until 1983 when Rorke3 and Becker and Hinton4 suggested that all malignant small cell tumors, including medulloblastoma, be classified as primitive neuroectodermal tumors (PNETs) and subdivided by location. Hart and Earle5 had previously used PNET to refer to supratentorial malignant small cell tumors that were believed to arise from primitive embryonal cells. Whether medulloblastoma should be considered under the rubric PNET or as a unique tumor type remains an area of debate. However, the fourth edition of the World Health Organization Classification of Tumours of the Central Nervous System, published in 2007,6 designates medulloblastoma as a distinct embryonal tumor and reserves the term central nervous system (CNS) PNET for neoplasms histologically indistinguishable from medulloblastoma but occurring outside the cerebellum (Table 126-1). This classification endorses the idea that medulloblastoma is a distinct entity from the other PNETs, as recent molecular studies have suggested.7 In addition, the incidence of medulloblastoma is higher than for all the other PNETs, further distinguishing it among PNETs.
TABLE 126-1 World Health Organization Classification of Embryonal Central Nervous System Tumors
From Louis DN, Ohgaki H, Wiestler OD, et al. WHO Classification of Tumours of the Central Nervous System. Lyon, France, IARC, 2007.
Epidemiology
Information gathered by the Central Brain Tumor Registry of the United States from 1998 to 2002 documents the incidence of medulloblastoma to be 0.6 per 100,000 in patients no more than 14 years old, and medulloblastoma accounts for 17% of all brain tumors in this age group.8 Although common in children, medulloblastoma is rare in adults, constituting less than 1% of all adult CNS tumors, with an estimated annual incidence of only 0.5 to 0.6 per million individuals.9–11 There appears to be a bimodal age distribution in children, with peaks at ages 3 to 4 years and at ages 8 to 9 years. Beyond these ages there is a steady decrease in incidence, with most medulloblastomas in adults occurring during the third and fourth decades.9,11 Males are more frequently affected with medulloblastoma, with a reported male-to-female ratio of 2 : 1,12 and whites are more frequently affected than African Americans.13
The cause of medulloblastoma is unknown. There is no particular geographic pattern to its occurrence, and no environmental factors have been specifically correlated with its development, although prenatal exposure to dietary N-nitroso compounds (as found in cured meats and beer) may pose a minor increased risk.14,15 In contrast, multivitamin, fruit, and vegetable intake by pregnant women may provide a small protective benefit.15 There is also some evidence that viral infection plays a role in the development of medulloblastoma, including the finding of the JC polyomavirus T-antigen in medulloblastoma cells.16 Although medulloblastoma can be induced experimentally by the transfection of simian virus 40 large T-antigen into fetal neural tissue, there is no evidence of a connection between simian virus 40 infection and the development of medulloblastoma in humans.17 Medulloblastoma has been associated with several familial cancer syndromes, suggesting a genetic basis for tumor development (Table 126-2).
TABLE 126-2 Familial Cancer Syndromes Associated with Medulloblastoma
Pathology
Gross Pathology
Medulloblastoma is a poorly demarcated, pink-purple, soft, friable mass that arises in the cerebellar vermis, usually from the inferior medullary velum. There may be foci of hemorrhage or necrosis, but cysts are unusual. Desmoplastic variants may be more firm, as a result of their greater connective tissue component.6 The tumor may extend through the fourth ventricle into the aqueduct of Sylvius or into the cisterna magna through the foramen of Magendie. Involvement of the cerebellar hemispheres is uncommon in children but is more frequent in adults. Brainstem infiltration is seen in 15% to 40% of surgical cases.18,19
Medulloblastoma has a strong propensity to metastasize. The most common site of metastasis is the subarachnoid space, where microscopic deposits, gross nodules, or en plaque masses may form along the brain or spinal cord. Autopsy series revealed that cerebrospinal dissemination occurs in up to 50% of patients.20 Extraneural metastasis occurs in about 5% of patients and most commonly involves the bone marrow.
Microscopic Pathology
The classic medulloblastoma is a highly cellular tumor composed of homogeneous fields of small, round basophilic cells with hyperchromatic nuclei, prominent nucleoli, and scant cytoplasm. Mitotic figures are numerous and may be bizarre. There may be foci of hemorrhage. Areas of necrosis may occur but are not extensive. Endothelial proliferation is not prominent. A granulofibrillary background is usually seen. The most common pattern is a continuous sheet of cells. Homer Wright rosettes (indicative of neuroblastic differentiation) may be found (Fig. 126-1). Grossly, a pseudocapsule may be present, but when viewed microscopically, cells are seen to invade the surrounding brain and often extend into the leptomeninges and subarachnoid space. Neuronal differentiation in medulloblastoma is common and is shown by staining for synaptophysin, neuron-specific enolase, neurofilament protein, microtubule-associated protein, and tubulin.21 Astrocytic differentiation, evidenced by positive glial fibrillary acidic protein immunoreactivity, can be seen in up to 50% of medulloblastomas.22 In addition, the expression of a variety of other antigens, including vimentin, nestin, neuronal cell adhesion molecules, nerve growth factors, and rhodopsin have all been documented.6,21
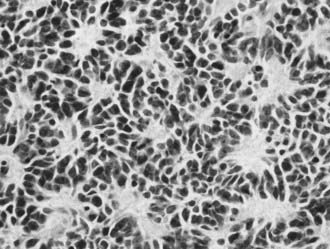
FIGURE 126-1 Photomicrograph of medulloblastoma showing small cells and multiple Homer-Wright rosettes.
The 2007 World Health Organization (WHO) classification of tumors of the central nervous system6 recognizes four histologic variants of medulloblastoma, including desmoplastic medulloblastoma, large cell medulloblastoma, and two newly recognized variants: medulloblastoma with extensive nodularity and anaplastic medulloblastoma (see Table 126-1).6 The most frequently seen variant is desmoplastic medulloblastoma,23,24 which is particularly common in older children and adults. It is distinguished from classic medulloblastoma by the presence of an intercellular stromal network consisting of reticulin and collagen that separates groups of typical medulloblastoma cells into islands. This desmoplastic variant constitutes roughly 20% of adult and 10% of childhood medulloblastomas,22 and it is seen with high frequency in patients with Gorlin’s syndrome, an autosomal dominant disorder resulting from mutation in the sonic hedgehog homologue (SHH) pathway (discussed later). Medulloblastoma with extensive nodularity, a new addition to the 2007 WHO classification, is closely related to the desmoplastic variant.24 It tends to occur in infants and differs from the desmoplastic variant by exhibiting a more extensive lobular pattern.24 The reticulin component present in abundance in the desmoplastic variant is reduced in this variant. Importantly, medulloblastomas with extensive nodularity and desmoplastic medulloblastomas are both associated with a more favorable prognosis compared with classic medulloblastomas.24–26
The other two variants, large cell medulloblastoma and anaplastic medulloblastoma (which is also new to the 2007 WHO classification) have features with considerable overlap, despite being recognized as two separate histologic variants. In most studies, patients with large cell and anaplastic medulloblastomas are categorized together. In general, large cell and anaplastic medulloblastomas display marked nuclear pleomorphism and high mitotic activity. Atypia is particularly pronounced and widespread in the anaplastic form. The large cell variant displays spherical cells with round nuclei, open chromatin, and prominent central nucleoli.24 Most importantly, both entities are associated with poor clinical outcome compared with classic medullobastoma. Indeed, in a study of 495 medulloblastomas from the Pediatric Oncology Group, the median survival time of patients with large cell or anaplastic medulloblastomas was 0.9 years, compared with 4.8 years in the control group.27 In a follow-up study, this result was further validated by showing that as the degree (slight versus severe) and the extent (focal versus diffuse) of anaplasia increased, the clinical outcome worsened.28 Similar results were obtained from a cohort of 273 medulloblastomas in the International Society of Pediatric Oncology/United Kingdom Children’s Cancer Study Group.29
Histogenesis
Medulloblastoma cells have an undifferentiated appearance that led to initial speculation that the tumor arises from multipotent stem cells. Recent studies have shown that CD133+ cells can be isolated from medulloblastomas, supporting the hypothesis that medulloblastomas arise from stem cells.30 Indeed, Cushing and Bailey proposed that medulloblastomas arise from primitive medulloblasts, one of the five types of stem cells that populate the primitive neural tube as described by Schaper.31 However, the medulloblast is now of historical significance only, because its existence has never been substantiated. More recently, two general hypotheses regarding the origin of medulloblastomas have emerged. In the first, medulloblastomas have been proposed to arise from primitive multipotent cells in the external granular cell layer (EGL) of the cerebellar medullary velum, which are unique to the cerebellum. An alternative view has been that medulloblastomas arise from multipotent cells in the subependymal-subventricular region and within the fetal pineal region, and give rise to all PNETs, regardless of location. These alternative views are reflected in the classification of medulloblastomas as a unique tumor type rather than as a subset of PNETs.
Current evidence has increasingly supported the latter view, namely that stem cells from the EGL, specifically granule neuron precursors (GNPs), are the cells of origin for medulloblastomas. This conclusion is based largely on experiments in animals in which disruption of the migration and differentiation of GNPs within the EGL resulted in the development of medulloblastomas (for a complete review, see reference 32). This evidence has led to the continued classification of medulloblastoma as a distinct entity from other PNETs in the 2007 WHO classification and is supported by large scale protein expression studies demonstrating distinct gene activation patterns between supratentorial PNETs and medulloblastomas.7
Molecular Biology
As with other cancers, medulloblastomas are frequently aneuploid,33 and a more aggressive course may be associated with increased ploidy. Likewise, losses or gains of chromosomes or chromosomal regions have been identified in medulloblastoma, including 1q, 5, 7, 8p, 10q, 11, 16q, and 17p.34,35 The most common abnormality involves a deletion of the short (p) arm of chromosome 17, usually in association with an isochromosome of 17q (I[17q]).36,37 Loss of 17p13.3 has been demonstrated in 26% to 50% of medulloblastomas.38,39 Because the tumor suppressor gene p53 is located at 17p13.1 and is infrequently mutated in medulloblastomas, it has been postulated that there may be a distinct gene at 17p13.3 that is involved in the tumorigenesis of medulloblastoma,37 but such a gene has not been identified.
Although it is common in medulloblastomas, loss of 17p is not seen in supratentorial PNETs.40 Although several studies suggest a correlation of poor prognosis with 17p deletion,36,38 these reports did not control for clinical characteristics that affect outcome.
Sonic Hedgehog Homologue Pathway
Various observations implicate the activation of the SHH pathway in the pathogenesis of medulloblastoma. SHH is a diffusible mitogenic protein that binds to the transmembrane receptor protein PATCHED1 (PTCH1). In the absence of SHH, PTCH1 is bound to another transmembrane protein, smoothened (SMO), and this PTCH1/SMO complex inhibits the glioma-associated oncogene homolog (GLI) family of transcription factors, whose overexpression promotes cellular proliferation. When SHH binds to PTCH1, the inhibitory effects of SMO on GLI are reversed, leading to activation of GLI and enhanced oncogenesis. The importance of the SHH pathway has been validated by global gene expression studies, which show that sporadic medulloblastomas are characterized by SHH signaling pathway activation.7,41 In addition, Gorlin’s syndrome, an autosomal dominant disorder that results from a loss-of-function mutation of the PTCH1 gene, is associated with an increased incidence of medulloblastoma.42,43 About 15% of sporadic medulloblastomas harbor mutations of PTCH1, particularly the nodular-desmoplastic subtypes.35,39 Gene mutations in SMO and GLI1 are found in sporadic medulloblastoma,44,45 and more than 30% of sporadic medulloblastomas demonstrate elevated GLI1 protein expression.45
WNT Pathway
Evidence implicating the WNT pathway in medulloblastoma formation comes from studies of patients with Turcot’s syndrome, who suffer from familial adenomatous polyposis and brain tumors, including medulloblastoma. The genetic defect underlying Turcot’s syndrome is an inactivating mutation in the APC gene,46 which results in overactivation of the WNT pathway. Similarly, loss-of-function mutations of AXIN and GSK-3β also activate the WNT pathway, and these mutations have been reported in sporadic medulloblastoma.47–50 β-CATENIN mutations have also been found in 5% to 10% of sporadic medulloblastomas.51,52 These mutations alter the phosphorylation sites on β-CATENIN, which inhibits targeted degradation and results in the activation of the WNT pathway. Immunochemical studies have documented elevated intranuclear localization of β-CATENIN protein in up to 25% of medulloblastomas.51,53
Other Signaling Pathways
The p53 tumor suppressor is a transcription factor that regulates cell cycle arrest, apoptosis, and cellular senescence. Although medulloblastoma has been reported to occur in patients with Li-Fraumeni syndrome (in which there is a germline mutation of p53), and despite the importance of p53 in other brain tumors, mutations of p53 are observed in only 5% to 10% of medulloblastomas.54 However, loss of p53 enhances medulloblastoma formation in mice heterozygous at the PTCH1 locus.55 Loss of Rb or LIG4 and other DNA repair genes in p53-null mice all promoted medulloblastoma formation.56–58 Although it is useful to show that manipulation of p53 in mouse model systems can increase the incidence of medulloblastoma formation, this may not faithfully recapitulate medulloblastoma formation in humans.
Insulin growth factor (IGF) pathway components, as measured by expression of activated AKT, IGF1, and IRS-1, are frequently activated in primary human medulloblastomas.59 IGF may represent a downstream, transcriptional target for SHH because its expression was observed to be increased in mouse model systems having an activated SHH pathway.60
MYC is an oncogenic transcription factor that has been shown to be important in granular neuronal precursor development. Both c-MYC and N-MYC have been implicated in medulloblastoma tumorigenesis. In one study, high levels of c-MYC expression were demonstrated both in medulloblastoma cell lines and in human specimens.61 Clinical correlative studies have shown that overexpression of c-MYC in large cell/anaplastic medulloblastomas is associated with a shorter patient survival time.27 Likewise, N-MYC amplification was associated with shorter survival times in a correlative clinical study from the International Society of Pediatric Oncology/United Kingdom Children’s Cancer Study Group.62 Although amplification of N-MYC showed a trend toward shorter survival of medulloblastoma patients in a study by the Pediatric Oncology Group, this effect was not statistically significant.63
Apoptotic pathway components, the antiapoptosis protein Bcl-2 being the most notable, have also been shown to enhance tumor formation in mouse model systems.64 These results confirm that cellular programs responsible for growth and differentiation, combined with the abrogation of apoptotic pathways, contribute to the tumorigenesis of medulloblastoma.
Mouse Model Systems and Novel Therapeutics
Based on observations in human tumors, mouse model systems of medulloblastoma have been created by direct manipulation of various components of the SHH pathway, including SHH, PTCH1, and SMO. In mice overexpression of SHH produced by retroviral vectors causes medulloblastomas.65,66 Loss of the inhibitory function of PTCH1 in downregulating the SHH pathway promotes medulloblastoma formation. In a manner similar to patients with Gorlin’s syndrome, mice that are haploinsufficient for PTCH1 will form spontaneous medulloblastomas about 15% to 20% of the time.67 Expression of constitutively activated SMO in cerebellar granule neuron precursors results in a high rate of medulloblastoma formation.68,69 Combining various SHH pathway mutations may directly enhance or suppress tumor phenotype in mice. For example, inactivation of GLI1 in mice heterozygous at PTCH1 results in an inhibition of medulloblastoma formation, which is consistent with GLI1 being a downstream effector of the SHH pathway.70,71
As mouse model systems of medulloblastoma have become more reliable in recapitulating the genotypic and phenotypic characteristics of human medulloblastoma, their utility in evaluating novel therapeutic agents has become apparent. The use of an SMO inhibitor has been shown to decrease tumor incidence significantly in a mouse model system.72 A monoclonal antibody directed against hepatocyte growth factor, shown to be highly expressed in human medulloblastoma, has also been shown to reduce tumor incidence significantly in a mouse model system.73 Preclinical testing of new therapies using these model systems may provide new agents for human testing.
Diagnostic Imaging Studies
Medulloblastoma appears as a well-defined, hyperdense midline mass on noncontrast computed tomography (CT) scans. Increased density correlates with hypercellularity and desmoplastic changes.74 The inferior cerebellar vermis is the most common location of the tumor, but medulloblastomas arising in the cerebellar hemisphere can be found in older patients. The most common pattern on postcontrast CT scans is homogeneous, intense enhancement, although minimal or no contrast enhancement or heterogeneous patterns can also occur. Scattered small areas of hemorrhage, calcification, or necrosis may be found. Hydrocephalus is seen in 85% to 90% of cases.
Magnetic resonance imaging (MRI) offers better detail than CT in terms of exact tumor location, brainstem infiltration, ventricular extension, and subarachnoid dissemination. Medulloblastoma displays a heterogeneous pattern on T1-weighted MRI studies of the cerebellum and varies from hypointense to hyperintense on T2-weighted images. Gadolinium-enhanced MRI generally reveals a heterogeneously enhancing mass, although the degree of enhancement varies (Figs. 126-2 to 126-4).75
Because medulloblastoma has a strong propensity to metastasize within the neuraxis, and because information on dissemination is important for staging (discussed later), the spinal axis should be imaged at the time of diagnosis. MRI has supplanted CT myelography for detecting metastatic seeding. MRI also better defines fine spinal nodules. Although the exact sensitivity of spinal MRI in detecting cerebrospinal metastases is unknown, recent reports suggest a high correlation between abnormal MRI findings and positive cytologic findings in cerebrospinal fluid (CSF) collected by lumbar puncture.76 Nevertheless, use of either spinal MRI or CSF cytologic analysis alone to diagnose leptomeningeal disease (LMD) has a false-negative rate of 14% to 18%. Thus, it is recommended to use both CSF cytology studies and spinal MRI together to diagnose LMD.77 Because blood generated during surgery may be detected in the spinal canal and confused with tumor metastases on postoperative spinal MRI scans, preoperative MRI is recommended when medulloblastoma is suspected, in order to minimize the likelihood of false-positive results on postoperative scans. If preoperative MRI is not performed, current recommendations are to wait 2 weeks before performing imaging on the spinal axis.
Staging
Staging is an important aspect of the management of patients with medulloblastoma. In 1969, Chang and colleagues78 were the first to propose a staging system for medulloblastoma. This operative staging system was adapted from the TNM system by eliminating the N (node) grade. The T (tumor) stage was originally based on the surgeon’s intraoperative assessment of the location and size of the tumor. More recently, the T stage has been determined by postoperative imaging. The M (metastasis) stage was determined on the basis of intraoperative observations, cerebrospinal fluid cytologic results, and postoperative myelography findings. More recently, spinal MRI has replaced myelography. The Chang system was modified subsequently (Table 126-3),79 and in the late 1980s, this modified system became the required staging system for multicenter clinical trials sponsored by the Children’s Cancer Group (CCG) and the Pediatric Oncology Group (POG). Alternative staging schemes have been suggested by Laurent,23 Schofield,80 and Sure81 and their respective coauthors. To varying degrees, these schemes consider tumor features, clinical features, extent of resection, histologic features, and extent of disease. Yet, none of these staging systems has been universally adopted.
TABLE 126-3 Modified Chang Classification for Medulloblastoma
T1 | Tumor < 3 cm in maximal diameter; limited to the midline vermis, roof of the fourth ventricle, or cerebellar hemisphere |
T2 | Tumor ≥ 3 cm in maximal diameter, invading one adjacent structure or partially filling the fourth ventricle |
T3A | Tumor ≥ 3 cm in maximal diameter with extension into the aqueduct of Sylvius, foramen of Magendie, or foramen of Luschka, thus producing hydrocephalus |
T3B | Tumor ≥ 3 cm in maximal diameter invading brainstem |
T4 | Tumor ≥ 3 cm in maximal diameter extending through the aqueduct of Sylvius to involve the midbrain or third ventricle, or down past the foramen of Magendie |
M0 | No metastatic disease |
M1 | Microscopic tumor cells in cerebrospinal fluid (cerebrospinal fluid cytology positive for tumor cells) |
M2 | Gross nodular seeding in cerebellar or cerebral subarachnoid space or in the third or lateral ventricles |
M3 | Gross nodular seeding in spinal subarachnoid space |
M4 | Extraneural metastasis |