Hypercalcemia
A. Ross Morton and Allan Lipton
• Hypercalcemia is a major metabolic complication associated with malignant disease.
• Hypercalcemia occurs in approximately 10% of patients with cancer.
• Hypercalcemia has a specific predilection for squamous carcinoma of the bronchus, carcinoma of the breast, and multiple myeloma.
• Hypercalcemia is frequently recognized late and managed poorly.
• Parathyroid hormone–related protein (PTHrP) produces hormonal and paracrine effects.
• Factors released by, or in response to, metastases in bone (receptor activator of nuclear factor–κB ligand [RANKL], PTHrP, transforming growth factor-α, tumor necrosis factor, interleukin-1 [IL-1], IL-6, and others) cause paracrine effects.
• The final common pathway is osteoclastic bone resorption.
• It is aggravated by renal functional abnormalities or renal effects of PTHrP, or both.
• Determination of the stage of disease and subsequent antineoplastic options provides a logical approach to management.
• Patient symptomatology is more relevant than the absolute calcium level.
• The total calcium concentration must be corrected for serum albumin concentration.
• Close attention to volume status and renal function are mandatory.
• Causes of hypercalcemia other than malignancy should be considered.
• Patients with symptoms due to their hypercalcemia should be treated as severely affected irrespective of the absolute calcium level.
• A corrected serum calcium level of less than 3.0 mmol/L is considered mild, 3.0 to 3.5 mmol/L is moderate, and greater than 3.5 mmol/L is severe.
• Antitumor therapy should be implemented for best long-term results.
• Consideration should be given to active palliation in the face of advanced disease when antitumor options are exhausted.
• Extracellular fluid volume should be expanded to induce a calciuresis.
• Antiresorptive therapy (bisphosphonates with or without calcitonin) should be considered as first-line therapy.
Introduction
Hypercalcemia in association with malignant disease was first reported by Zondek and colleagues in 1924,1 and the first review of a large series was by Gutman and coworkers in 1936.2 Since then the syndrome has become increasingly well recognized and characterized. The frequency with which hypercalcemia occurs varies considerably with tumor type, but it is most commonly seen in association with squamous carcinoma of the bronchus, carcinoma of the breast, and multiple myeloma. It is of considerable interest that some tumors that frequently metastasize to bone—for example, small-cell carcinoma of the lung, carcinoma of the prostate, and some other common tumors, such as adenocarcinoma of the colon and stomach—are infrequently associated with hypercalcemia.
Etiology
Before we discuss the possible etiologies of hypercalcemia in malignant disease, a short review of normal calcium homeostasis is appropriate. (For a more extensive review, see the article by Peacock.3) The adult human body contains approximately 1 kg of calcium, of which all but 10 g is lodged in bone. Most of the extraosseous calcium is found in the extracellular fluid, but the minute concentrations present in cells (10−8 to 10−7 M) are vital to normal cellular function and control. The total amount of calcium in the body is dependent on the balance between calcium intake and calcium loss. Figure 37-1 demonstrates normal calcium metabolism.4 Normal dietary calcium intake is approximately 1 g per day (25 mmol). Absorption of dietary calcium is incomplete (25% to 50%), and in healthy persons it is approximately 300 mg (7.5 mmol per day). Although an enormous reservoir of calcium is present in bone, very little transfer of calcium (on the order of 500 mg or 12.5 mmol per day) occurs between bone and plasma in healthy persons. When net calcium balance is zero, the body is required to excrete approximately 150 mg (3.75 mmol) of calcium daily. The kidney filters large amounts (10 g or 250 mmol) of calcium daily. Of this amount, 65% is reabsorbed in the proximal convoluted tubule, 25% is reabsorbed in the ascending limb of the loop of Henle, and a variable amount is reabsorbed in the distal convoluted tubule. Calcium reabsorption in the proximal tubule is independent of hormonal control but is closely linked to the reabsorption of sodium, a phenomenon that has important consequences in, and implications for, the treatment of hypercalcemia. Calcium reabsorption from the distal tubule is enhanced in the presence of parathyroid hormone (PTH), and it is at this site that the fine-tuning of calcium homeostasis occurs. Bone resorption can increase by approximately 150% over bone formation before the renal clearance mechanisms are overwhelmed.
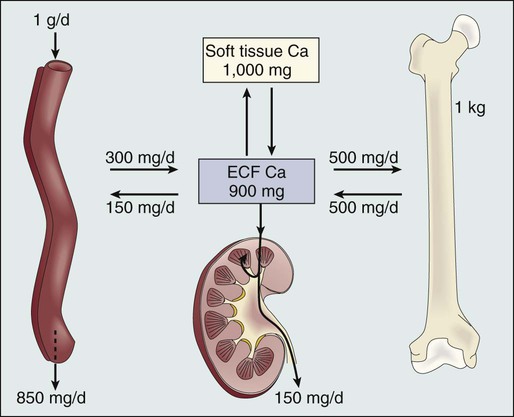
The total plasma calcium consists of free plasma calcium (which amounts to approximately 50% of the total) and calcium bound to albumin (and to a lesser extent to other proteins, including paraproteins), which varies with the level of plasma proteins but accounts for approximately 40% of the total. The remaining 10% is in complex with ions such as bicarbonate and citrate (Fig. 37-2). In physiological terms, the plasma free (or “ionized”) calcium carries the greatest significance. Direct measurement of the plasma free calcium is possible using ion-selective electrodes, but for the most part, total plasma calcium is measured. A reasonable correlation exists between serum albumin and serum calcium levels, and therefore algorithms have been suggested to “correct” the total plasma calcium for albumin concentration. Although no algorithm is 100% specific or sensitive for the detection of all true cases of hypercalcemia, the following equation has the merits of accuracy and simplicity.
< ?xml:namespace prefix = "mml" />


The serum level of ionized calcium is tightly controlled. The major hormonal determinant of ionized calcium is PTH. This hormone brings about its effects by altering calcium resorption from bone, calcium reabsorption in the kidney, and, via stimulation of formation of active vitamin D (1,25-(OH)2D3), calcium absorption from the gastrointestinal tract. The active form of circulating PTH is a polypeptide containing 84 amino acid residues; however, only the first 34 amino acid residues are required for activity related to calcium homeostasis. Several larger C-terminal fragments of PTH are found in circulation (most notably in the presence of renal insufficiency). The role of these peptides, and particularly the large PTH(7–84) fragment (which has been postulated to have antagonistic properties to PTH(1–84) and is secreted from the parathyroid glands during hypercalcemia), remains controversial. An excellent review on the topic of PTH has been written by Potts.5
PTH release and, to a lesser extent, formation is under the control of the calcium-sensing receptor (CaSR), which is located on the external cell membrane of parathyroid chief cells. This receptor is a G-protein coupled receptor with a large extracellular domain that is responsible for calcium binding, seven transmembrane domains, and a smaller intracellular domain responsible for signal transduction. Stimulation of the CaSR by even mild changes in ionized calcium level results in a rapid and profound change in the secretion of PTH, as well as more delayed effects on PTH formation.6
The parathyroid glands also manufacture the hormone calcitonin. Although pharmacologic doses of calcitonin have effects on plasma calcium levels when given acutely, considerable controversy remains about its true physiological role7 because in both the absence of calcitonin (after a total thyroidectomy) and in the presence of large circulating amounts (seen in medullary carcinoma of the thyroid), gross disturbance of calcium balance is extremely rare.
Types of Hypercalcemia of Malignancy
Humoral Hypercalcemia of Malignancy
In 1980, Stewart and colleagues8 described a series of 50 patients with hypercalcemia and malignant disease. In their extensive metabolic evaluation, they were able to characterize the patients into two groups dependent on their excretion of nephrogenous cyclic adenosine monophosphate (cAMP). Patients with high nephrogenous cAMP shared other features with primary hyperparathyroidism, including a lowered renal phosphate threshold. However, significant differences between these groups were found in terms of fasting urinary calcium excretion, 1,25-(OH)2D3 concentrations, and immunoreactive PTH levels. The investigators concluded that urinary nephrogenous cAMP was a useful marker for identifying hypercalcemia of malignancy associated with a humoral factor—so-called humoral hypercalcemia of malignancy (HHM)—but that this factor was not native PTH.
Parathyroid Hormone–Related Protein
Advances in molecular biology, in association with the failure to detect circulating immunoreactive PTH in patients with hypercalcemia, cast increasing doubt on the role of native PTH in HHM. In 1983, Simpson and colleagues,9 using complementary DNA probes to PTH messenger RNA (mRNA), failed to demonstrate the production of PTH mRNA in many of the tumors considered to be prime candidates for ectopic PTH secretion.
In 1987, Burtis and associates,10 Moseley and coworkers,11 and Strewler and colleagues12 published descriptions of a polypeptide hormone isolated from tumors that are associated with the hypercalcemia of malignancy. The primary structure of these peptides shows considerable N-terminal homology with native PTH (Fig. 37-3) and has led to the terminology “parathyroid hormone–related protein” (PTHrP). Although PTHrP is the main humoral factor in patients with hypercalcemia of malignancy, information is increasing about its normal physiological roles.13 Furthermore, the importance of PTHrP is increasingly being recognized, not only in the genesis of HHM, but also in the facilitation of cancer growth and metastasis and interaction with the RANK/RANK-ligand/osteoprotegerin system.14
Vitamin D–Linked Hypercalcemia
In addition to the well-known association of hypercalcemia in sarcoidosis, abnormal vitamin D metabolism, characterized by a substrate-dependent conversion of 25-hydroxyvitamin D3 to 1,25-(OH)2D3, has been described in association with lymphomas. Indeed, about half of patients with lymphoma who present with hypercalcemia have abnormal amounts of 1,25-(OH)2D3, whereas the remainder have elevated concentrations of PTHrP. It seems that the cells responsible for the activation of vitamin D are macrophages in close proximity to the lymphoma cells.15
It has become apparent that as many as 50% or more of patients with adult T-cell lymphoma have hypercalcemia. Evidence suggests that the mechanism of hypercalcemia in this lymphoma type is due to overexpression of the receptor activator of nuclear factor-κB ligand (RANKL; see later discussion) in the malignant T cells. Work by Okada and associates16 points to the role of macrophage inflammatory protein–1α as a RANKL-inducing agent; it also has a recruiting effect on osteoclast precursors.
The role of pharmacologic doses of active vitamin D and its analogs in the treatment of malignant disease is increasing. The major early drawback to this therapy has been the development of drug-induced hypercalcemia. The introduction of intermittent dosing, the combination with dexamethasone or bisphosphonates, and the promise of less calcemic analogs goes some way to circumventing this problem (see the reviews in references 17 and 18).
Local Osteolytic Hypercalcemia
Multiple Myeloma
Bone resorption, osteopenia, and hypercalcemia are characteristic features of patients with multiple myeloma. The neoplastic cells are in close proximity to bone by the very nature of the condition. Many factors responsible for local osteolysis have been identified and are produced by or in response to myeloma cells in the marrow. Collectively, these factors have been called osteoclast-activating factors and include interleukin-1 (IL-1), IL-6, IL-11, PTHrP, hepatocyte growth factor, tumor necrosis factor, and macrophage inflammatory protein–1α, among others (reviewed by Yeh and Berenson19).
The identification of the cytokine system involving RANKL, the target of this polypeptide (receptor activator of nuclear factor-κB [RANK]), and the controlling inhibitor osteoprotegerin (OPG) that acts as a soluble decoy receptor has represented a major breakthrough in the understanding of local control of bone cell biology (reviewed by Hofbauer and Heufelder20). Essentially, RANKL, which normally is produced by osteoblasts, is responsible for osteoclast differentiation and function. In patients with multiple myeloma, the RANK/RANKL/OPG system is deranged. Multiple myeloma cells in the bone marrow increase the presence of RANKL either by direct secretion or by stimulating stromal cell production. Furthermore, depression of OPG availability occurs, both at the level of protein synthesis and after OPG has been secreted as a result of binding to CD-138 (syndecan-1) elaborated by multiple myeloma cells.21
Carcinoma of the Breast
Despite this finding, it is also clear that not all cases of hypercalcemia in persons with carcinoma of the breast are wholly dependent on metastatic disease. Indeed, one of the original tumor types from which PTHrP was isolated was breast cancer.10 In an early study of 98 women with varying degrees of breast cancer, Bundred and coworkers22 noted elevated PTHrP levels in 12 of 13 hypercalcemic patients. Furthermore, tumor staining for PTHrP was positive in 22 of 25 patients who had bone metastases and in whom hypercalcemia later developed.
It has become increasingly clear that cell-cell interaction between breast cancer cells and local bone cells has much to do with the maintenance of the metastatic phenotype in bone. Local production of PTHrP by breast cancer cells can increase osteoclast development and recruitment via RANKL. Enhanced bone resorption can release stored factors, including insulin-like growth factor–1 and transforming growth factor–β. These substances support the growth of the malignant cells. Osteoclastic mobilization of calcium increases tumor production of PTHrP (mediated in part by the CaSR), resulting in a vicious cycle. Cellular cross talk as it relates to bone metastases has been elegantly reviewed by Yoneda and Hiraga.23
Special Cases
Pseudohypercalcemia
The phenomenon of pseudohypercalcemia is a rare condition in which excess calcium bound to nonalbumin plasma proteins results in an elevated total serum calcium concentration. These proteins are usually monoclonal proteins associated with multiple myeloma and benign monoclonal gammopathy.24 The ionized calcium concentration is normal under these circumstances, but correction formulas using albumin give falsely abnormal results.
Tamoxifen-Linked Hypercalcemia
Hypercalcemia in association with the use of estrogen or antiestrogen therapy for carcinoma of the breast has been recognized for more than 50 years. The severity of the hypercalcemia is variable, but it can be fatal. The mechanism by which tamoxifen and similar agents cause hypercalcemia is unclear. Cell culture studies suggest that prostaglandins could be the main mediators of the response.25 A role for prostaglandins would be compatible with the clinical picture of a tamoxifen “flare,” which, in addition to the hypercalcemia, is frequently associated with bone pain.