Hematopoietic Stem Cell Transplantation
Qaiser Bashir and Richard Champlin
• Hematopoietic cell transplantation (HCT) is a potentially curative treatment for a variety of hematopoietic, immune, metabolic, and malignant diseases.
• Hematopoietic stem cells for transplantation can be collected from bone marrow, peripheral blood, or umbilical cord blood.
• With the advent of unrelated donor, haploidentical, and cord blood transplantation, virtually every patient can now have a suitable donor.
• Reduced-intensity conditioning regimens are associated with lower treatment-related mortality and allow for transplantation in elderly and patients with comorbidities who are not candidates for myeloablative HCT.
• Allogeneic HCT is most frequently performed for patients with acute and chronic leukemias, myelodysplastic syndromes, immune deficiencies, metabolic disorders, and bone marrow failure states.
• Autologous HCT is usually performed as part of the initial treatment for multiple myeloma, relapsed Hodgkin and non-Hodgkin lymphoma, and select solid tumors.
• Common complications of HCT include regimen-related organ toxicity, graft rejection, graft-versus-host disease, infections, and secondary malignancies.
Introduction
Allogeneic HCT
Allogeneic HCT can be used if the patient’s bone marrow is involved by the malignancy or damaged by the disease or prior therapy. Allogeneic HCT carries the risk of immune complications including graft rejection (a situation in which the recipient rejects the donor cells) or graft-versus-host disease (GVHD) (a situation in which the immune cells from the donor react against recipient tissues). Allogeneic HCT may also confer a beneficial immune-mediated graft-versus-malignancy effect in which donor immune cells react against residual malignant cells.2 Compared with autologous transplants, allogeneic HCT is generally more effective in eradicating malignancies, but it has a greater risk of treatment-related mortality as a result of GVHD and infections.
Histocompatibility
An international network of registries to provide an HLA-matched unrelated donor has been established. More than 18 million potential donors can be accessed worldwide. Initial studies of unrelated donor transplants reported a relatively high rate of GVHD and treatment-related mortality. Improved results have been achieved with donors and recipients matched for HLA-A, HLA-B, HLA-C, and DRB1 using high-resolution (allele level) typing,3 and recent results with matched unrelated donor transplants are similar to those achieved with matched sibling donors.
Cell Sources for HCT
After HCT, hematopoiesis and immunity are predominantly derived from donor-derived hematopoietic stem cells, although mature T cells in the graft contribute to initial immune recovery. Human hematopoietic stem cells and progenitor cells are contained in the CD34+ fraction of bone marrow cells, which constitutes approximately 1% of the bone marrow. Bone marrow was initially the source of hematopoietic cells for transplantation, collected by multiple aspirations with the patient under general anesthesia. Hematopoietic cells also circulate in the peripheral blood, and their frequency increases after treatment with growth factors and after chemotherapy and peripheral blood progenitor cells can be used for transplantation. Larger numbers of CD34+ cells can generally be collected as peripheral blood progenitor cells, and hematopoietic recovery is accelerated with peripheral blood progenitor cell transplants compared with bone marrow; as a result, almost all autologous HCTs utilize peripheral blood progenitor cells. Several studies have compared the outcome of allogeneic HCT using bone marrow or peripheral blood progenitor cells. These studies uniformly show more rapid time to engraftment with peripheral blood progenitor cells but an increased risk of chronic GVHD (cGVHD). Some investigators have suggested superior disease-free survival (DFS) in matched siblings using peripheral blood progenitor cells,4 but in most studies, overall survival (OS) was similar. Bone marrow has produced improved outcomes compared with peripheral blood progenitor cells in children and in patients with aplastic anemia.
Umbilical cord blood is an alternative source of hematopoietic stem cells for transplantation. Cord blood units are obtained by collecting blood remaining in the umbilical cord and placenta after the delivery of an infant. Umbilical cord blood transplants are less likely to produce GVHD than are hematopoietic cells from adult donors, which allows successful transplants using unrelated units matched for four or five of the six HLA-A, HLA-B, and HLA-DR antigens.5 An international network of cord blood banks has been established, with collection of umbilical cord blood from volunteer unrelated donors after delivery of the newborn. Approximately 500,000 umbilical cord blood units are available worldwide. An umbilical cord blood unit provides a relatively low stem cell dose, which results in a slower pace of hematopoietic and immune recovery. Results are improved with higher cell doses and better HLA matching. Adequately matched umbilical cord blood units can be identified for most patients. Initial studies reported better results in children than in adults. A sufficient cell dose cannot be achieved with a single umbilical cord blood unit for most adult recipients; two umbilical cord blood units have been reported to improve outcomes and allow treatment of most adults. Centers focusing on this approach have reported results in children and adults similar to those obtained with matched bone marrow and peripheral blood progenitor cell transplants from unrelated adult donors. A recent registry analysis showed similar results with double umbilical cord blood transplants as with matched or one antigen mismatched unrelated donor peripheral blood progenitor cell transplants.6 Because the units are already collected and banked, umbilical cord blood transplants can generally be performed more rapidly than transplants from an unrelated adult donor.
Haploidentical relatives are another potential donor source. Parents, children, and half of siblings are haploidentical, and thus these donors are readily available for most patients. Several centers have reported success with transplantation of T-cell–depleted peripheral blood progenitor cells with a low rate of GVHD.7 These transplants are associated with a relatively high rate of rejection, slow immune recovery, and a substantial risk of treatment-related mortality. An alternative approach uses unmodified haploidentical bone marrow transplantation with posttransplant treatment with cyclophosphamide, tacrolimus, and mycophenolate; this regimen produces a low rate of severe acute and chronic GVHD and treatment-related morbidity and mortality.8
Recent multicenter studies confirm that successful transplants can be performed in children and adult recipients from either unrelated cord blood or a haploidentical related donor9; overall, results have been similar with each approach and comparable with those reported with matched unrelated donors. Further studies are required to directly compare these strategies and to optimize results of HCT from every donor source. Use of these alternative donors provides an opportunity for treatment of nearly all patients with a clinical indication for HCT. A comparison of different sources of hematopoietic stem cells is presented in Table 30-1.
Table 30-1
Comparison of Different Types of Allogeneic Transplantation
Related | Unrelated | Cord Blood | Haploidentical | |
Chances of finding a match | 25% | 50% | 90% | >95% |
HLA-matching requirement | Strict (8/8 is optimal; 1 mismatch may be feasible) | Strict (8/8 is optimal; 1 mismatch may be feasible) | Less strict (6/6 is optimal; 1 or 2 mismatches allowed) | Less strict (matched at only one haplotype) |
Banking needs | Minimal* | Minimal* | Dedicated CB banks | Minimal* |
Time required | 15-30 days | 3-4 mo | 15-30 days | 15-30 days |
Donor attrition | Unlikely | Likely | Unlikely | Unlikely |
Donor safety | Toxicity due to BM harvest or G-CSF | Toxicity due to BM harvest or G-CSF | None | Toxicity due to BM harvest or G-CSF |
Type of graft | BM or PB | BM or PB | Cryopreserved CB | BM or PB |
Stem cell dose | Standard† | Standard† | Low | Standard† |
Graft manipulation | TCD | TCD | None | TCD |
Conditioning | Myeloablative conditioning or reduced- intensity conditioning | Myeloablative conditioning or reduced- intensity conditioning | Myeloablative conditioning or reduced- intensity conditioning | Myeloablative conditioning or reduced-intensity conditioning |
Engraftment | Standard† | Standard† | Delayed | Delayed |
Treatment-related mortality | Standard† | Standard† | High | High |
Risk of GVHD | Standard† | Slightly high | Low | High |
Availability of additional cells (DLI) | Yes | Yes | None | Yes |
Immune reconstitution | Standard† | Standard† | Delayed | Delayed |
*Grafts are occasionally cryopreserved.
†For the purpose of this table, results with a related donor transplant are considered standard.
Disease Indications for HCT
This review focuses on HCT for treatment of cancer. HCT is performed for a wide array of hematologic malignancy and chemotherapy-sensitive solid tumors. Allogeneic HCT is associated with higher treatment-related mortality compared with autologous HCT, but it provides a graft that is free of disease, has a competent immune system, and confers the immune-mediated graft-versus-malignancy effect. Donor lymphocyte infusions (DLIs) can be given to augment this antitumor effect and may induce complete remission (CR) in selected patients with disease relapse after HCT.10
Because HCT is associated with significant morbidity and mortality, it is generally reserved for life-threatening diseases for which no other satisfactory treatment option exists. As new therapeutic options continue to evolve, so does the role of HCT. The indications for HCT vary by the disease type. Several organizations such as the European Blood and Marrow Transplantation11 and the National Comprehensive Cancer Network (www.nccn.org) have provided detailed treatment guidelines for specific diseases and clinical settings.
The indications for HCT vary by disease type. Results are better in patients who have chemosensitive disease or are in remission. Outcomes of HCT are not favorable in patients with poor performance status and who have refractory or progressive disease. Evidence-based reviews have been published by the American Society for Blood and Marrow Transplantation regarding the role of blood and marrow transplantation in the treatment of selected disease.12 The numbers of patients receiving autologous and allogeneic transplantation for each major indication are shown in Figure 30-1.
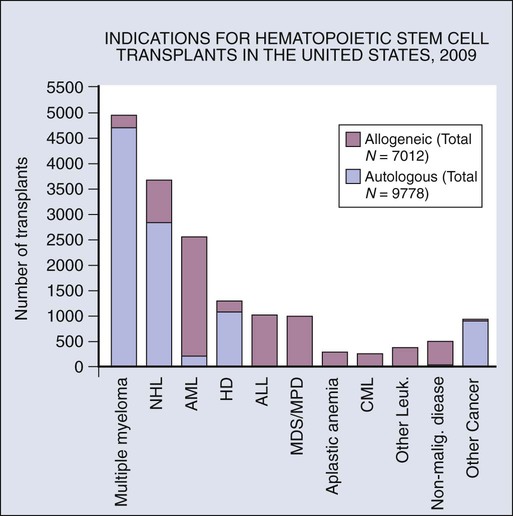
Acute Myeloid Leukemia
Allogeneic HCT is an optimal treatment for appropriately selected patients with acute myeloid leukemia (AML).13 Some centers have also successfully used autologous HCT for patients with AML who are experiencing their first or second remission. Recent advances have allowed risk stratification of patients with AML based on cytogenetic and molecular abnormalities, and this risk stratification helps guide therapeutic decisions.14 HCT is not recommended for patients in the “favorable” category. Large metaanalyses have shown that availability of an HLA-matched sibling did not result in superior DFS or OS with allogeneic HCT performed in the first period of remission for patients with favorable cytogenetics, t(8;21), inv16, or t(15;17). HCT prolongs DFS in patients with “intermediate-risk” and “high-risk” disease. Patients with diploid cytogenetics are considered intermediate risk, but recent studies have identified prognostically important subgroups based on molecular abnormalities. A large donor versus no-donor analysis of patients with cytogenetically normal AML showed that patients with the prognostically adverse FLT3-internal tandem duplication had improved DFS with allogeneic transplantation.15 Patients with nucleophosmin (NPM1) or CEBPA mutations without FLT3-internal tandem duplication had a better prognosis with chemotherapy, and there was a similar outcome with HCT compared with standard chemotherapy. HCT can also result in long-term DFS in approximately one third of patients with primary refractory AML.
AML is most common in elderly persons. Reduced intensity conditioning HCT allows for successful transplantation in patients with AML who would otherwise be considered ineligible because of age or comorbidities. Disease relapse is an important concern after HCT. The prognosis of patients who relapse with AML after undergoing a transplant is poor. However, approximately 20% of patients with chemosensitive disease can achieve a durable remission with a second allogeneic transplant16 (see Chapter 98).
Myelodysplastic Syndromes
Allogeneic HCT is an established indication for the treatment of myelodysplastic syndromes (MDSs).17 An international prognosis scoring system (IPSS) is used to classify patients with MDS into low, intermediate-1, intermediate-2, and high-risk categories based on bone marrow blast percentage, karyotype, and cytopenias.18 A modification of the IPSS system that incorporates a larger number of cytogenetic abnormalities, IPSS-R, was recently presented.19 Allogeneic HCT is generally not recommended for patients with low-risk MDS because long-term disease control is usually achieved with standard therapies. Allogeneic HCT is indicated for patients with low or intermediate-1 risk disease who fail to respond to standard therapy and for patients with intermediate-2 or high-risk MDS.20 HCT is also effective for patients with treatment-related MDS who generally have a poorer prognosis compared with de novo MDS.21,22 Seventy-five percent of patients with MDS are older than 60 years. Several studies have shown that reduced intensity conditioning allogeneic HCT is feasible in elderly patients and can induce long-term disease remission (see Chapter 99).
Myeloproliferative Syndromes
Allogeneic HCT is also a potentially curative therapy for myelofibrosis. About a third of patients with primary myelofibrosis can achieve long-term DFS and potential cure with myeloablative conditioning regimens. HCT is feasible for either primary myelofibrosis or after diagnosis of polycythemia vera or essential thrombocythemia. Reduced intensity conditioning allogeneic HCT is also effectively used for myelofibrosis. The treatment-related mortality is generally lower and the long-term survival is generally similar to that seen with myeloablative conditioning allogeneic HCT25–25 (see Chapter 100).
Chronic Myeloid Leukemia
Allogeneic HCT can induce long-term CRs in patients with chronic myeloid leukemia (CML).26 However, the introduction of tyrosine kinase inhibitors has resulted in extraordinary improvements in survival of persons with CML, and these agents are recommended for initial treatment. Allogeneic HCT is generally reserved for patients with suboptimal response or failure of treatment with tyrosine kinase inhibitors. Reduced intensity conditioning allogeneic HCT allows for wider application of transplantation. CML is very sensitive to the graft-versus-leukemia effect of donor T cells. Patients who experience a relapse after allogeneic HCT can be treated successfully with DLI27 (see Chapter 101).
Acute Lymphoblastic Leukemia
Allogeneic HCT is an effective treatment for persons with ALL.28 In children, allogeneic HCT is generally used for patients with induction failure, high-risk disease (Ph+ or MLL mutation), and severe hypodiploid and for infants with ALL. Allogeneic transplants are indicated for pediatric patients experiencing a second period of remission, particularly those who have disease recurrence while receiving maintenance chemotherapy.29 In adults, allogeneic HCT is indicated for patients with primary refractory or relapsed disease.30 Data conflict regarding the role of allogeneic HCT for patients experiencing their first CR. The large Medical Research Council and Eastern Cooperative Oncology Group showed that Ph-negative patients with a donor had improved OS compared with patients undergoing standard chemotherapy.28 HCT is most clearly indicated for patients with Ph+ ALL or MLL mutations because of the poor prognosis observed with standard chemotherapy in these patients. Autologous HCT has been advocated for consolidation after first or second remission; however, the results are generally superior with allogeneic HCT (see Chapter 98).
Chronic Lymphocytic Leukemia
Chronic lymphocytic leukemia (CLL) cells are susceptible to graft-versus-leukemia effects, and studies have shown that allogeneic HCT is feasible and can produce durable remissions in selected patients with advanced CLL. Reduced intensity conditioning regimens have been successful, particularly in patients with chemotherapy-sensitive relapse. Allogeneic HCT may overcome the adverse prognostic effect of zeta-chain–associated protein kinase–70 expression.31 Certain high-risk patients such as those with 17p13 and 11q22 abnormalities may benefit from allogeneic HCT during their first period of remission. Autologous HCT has also been studied for the treatment of CLL. This approach has a lower risk of treatment-related mortality and can induce prolonged remission; however, it is not curative, and OS is not improved (see Chapter 102).
Lymphoma
Lymphomas are the most common indication for autologous HCT. Lymphomas are a heterogeneous group of diseases that encompass malignant disorders of B cells, T cells, or natural killer (NK) cells. Both allogeneic and autologous transplants have been studied. Autologous HCT is generally recommended for patients with Hodgkin lymphoma and aggressive non-Hodgkin lymphoma who experience chemosensitive relapse.32 Allogeneic HCT can produce a graft-versus-lymphoma effect and is associated with a lower relapse rate. Several studies of myeloablative conditioning and reduced intensity conditioning allogeneic HCT have shown the feasibility of HCT for select groups of high-risk patients33 (see Chapters 105 to 108).
Follicular Lymphoma
HCT is indicated for persons with relapsed or refractory follicular lymphoma (FL) or for patients with histologic transformation to a more aggressive lymphoma subtype. HDT and autologous HCT during the first remission improve progression-free survival (PFS), but OS is generally similar to that seen with standard chemotherapy.34 An increased risk of secondary malignancies occurs after autologous HCT, and in the absence of OS benefit, autologous HCT is not indicated as first-line treatment for most patients. A plateau in the survival curve can be seen after prolonged follow-up in a fraction of patients who undergo autologous HCT during a second or subsequent remission.35 The incorporation of high-dose radioimmunotherapy into pretransplant conditioning may improve the outcome of autologous HCT in persons with relapsed FL. Ex vivo purging has been studied to deplete lymphoma cells from the graft. Recently, in vivo purging with rituximab maintenance after autologous HCT was reported to prolong PFS.36
Both myeloablative conditioning and reduced intensity conditioning allogeneic HCT are feasible treatment options for advanced FL. Myeloablative allogeneic HCT may be curative, but it has a relatively high treatment-related mortality. FL is susceptible to graft-versus-lymphoma effect, and long-term results of reduced intensity conditioning allogeneic HCT show that a high cure rate can be expected in patients with relapsed chemosensitive disease.37 Umbilical cord blood transplants have also been used successfully for the treatment of advanced lymphoid malignancies in patients who lack a suitable sibling donor (see Chapter 106).
Large Cell and Aggressive B-Cell Lymphomas
Autologous HCT is recommended as salvage therapy for patients with relapsed diffuse large B-cell lymphoma (DLBCL) who have chemosensitive disease.29 Autologous HCT is also recommended for patients who have chemosensitive disease but have never achieved CR; 30% to 60% long-term DFS can be seen in these patients. Autologous HCT has also been studied as part of front-line therapy for patients with poor prognostic features. Use in this setting is controversial. Randomized trials have conflicting results.38 The use of rituximab around the time of transplant may improve the outcomes of autologous HCT.39 However, emerging data suggest that patients who experience a relapse after first-line therapy that includes rituximab may have a poorer prognosis with autologous HCT than in the past before rituximab was available.40 Patients with negative results of a positron emission tomography/computed tomography scan at HCT have longer PFS compared with patients who have positron emission tomography–positive disease.41 Allogeneic HCT is associated with a lower relapse rate compared with autologous HCT,42 but this benefit is offset by higher rates of treatment-related mortality, and the indications for allogeneic transplantation in patients with DLBCL are not clearly established. It should be considered for select high-risk patients with relapsed chemosensitive DLBCL, particularly those who experience a relapse after undergoing an autologous HCT.
Both autologous and allogeneic HCT are effective therapies for patients with lymphoblastic lymphoma.43 Autologous HCT has also been used successfully for the treatment of persons with human immunodeficiency virus–associated lymphomas44 and persons with relapsed Burkitt lymphoma that is sensitive to salvage chemotherapy45 (see Chapter 107).
Mantle Cell Lymphoma
Mantle cell lymphoma is an aggressive lymphoma that generally has a poor prognosis. Autologous HCT has been shown to prolong PFS as part of initial therapy in randomized controlled trials.46 Autologous transplants are generally ineffective for patients with relapsed mantle cell lymphoma. Promising results have been reported with allogeneic HCT in these patients.47
Hodgkin Lymphoma
Sustained remissions can be achieved with high-dose chemotherapy and autologous HCT for relapsed or refractory Hodgkin lymphoma.49 Autologous HCT has also been used for first remission consolidation in high-risk patients; however, no clear OS benefit is seen with autologous HCT in this setting. No consensus has been reached about early versus late intensification for patients with high-risk Hodgkin lymphoma. A graft-versus-Hodgkin lymphoma effect has been documented, and a small proportion of patients can potentially be cured with allogeneic HCT. Allogeneic HCT is generally indicated for patients who have high-risk recurrent disease or relapse after an autologous HCT.50 Promising results have also been seen with alternate donor transplantation using cord blood or haploidentical donors (see Chapter 105).
Multiple Myeloma and Plasma Cell Disorders
Plasma cell disorders are the most common indication for autologous HCT. Autologous HCT is recommended as part of first-line treatment for eligible patients with multiple myeloma.51 Randomized trials have shown that high-dose melphalan chemotherapy and autologous HCT is associated with improved CR rate and prolongation of PFS.52 In some but not all studies, OS is improved. Results have improved with the recent use of lenalidomide and proteasome inhibitors, and ongoing studies are comparing chemotherapy alone with chemotherapy consolidated by autologous HCT. A more intense approach is performing tandem autologous HCT. Data from a randomized trial showed that the patients who failed to achieve near-CR after initial autologous HCT had a trend toward improved OS with a tandem transplant.53 This benefit has not been confirmed in other studies. Recently the results of maintenance therapy with lenalidomide after initial autologous HCT have shown to increase event-free survival and OS.54 Until further data become available, incorporation of high-dose chemotherapy and autologous HCT remains the standard in front-line treatment for patients with newly diagnosed myeloma who are considered medically fit to tolerate the procedure.
Allogeneic HCT can induce durable remissions in selected patients with multiple myeloma. A graft-versus-myeloma effect is well documented but less potent in this disease than with chronic leukemias. Reduced-intensity conditioning regimens have led to reduced treatment-related mortality but may increase the relapse rate.55 A number of randomized trials have compared tandem autologous transplants with an autotransplant followed by a reduced intensity conditioning allogeneic transplant to try to exploit the graft-versus-myeloma effect. Two randomized trials showed improved event-free survival and OS with this approach, but these results were not reproduced in other studies, including a large study by the Blood and Marrow Transplant Clinical Trials Network.56 Overall, available data do not support the routine use of allogeneic HCT as part of initial therapy for newly diagnosed patients outside of a clinical trial. Allogeneic HCT can be effective in patients with chemotherapy-sensitive relapsed disease for whom there are no other treatment options; approximately 20% of patients may achieve long-term survival (see Chapter 104).
Amyloidosis
High-dose melphalan therapy followed by autologous HCT is an effective treatment for patients with light chain amyloidosis. This approach is considered the standard of care, which may provide prolonged disease control and may prolong survival.57 One randomized clinical trial comparing autologous HCT with standard chemotherapy failed to show benefit for autologous HCT; however, this trial was criticized for the high rate of treatment-related mortality at the participating transplant centers.58 Increasing numbers of organs involved, particularly decompensated cardiomyopathy, increases the risk of treatment-related mortality. However, a substantial proportion of patients, including those with early cardiac involvement, can have reversal of amyloid-related disease after autologous HCT (see Chapter 104).
Solid Tumors
Solid tumors that show a steep dose response to myelosuppressive chemotherapy or radiation may benefit from HDT and autologous stem cell transplantation. Autologous stem cell transplants are widely used for treatment of advanced germ cell tumors59 and can produce durable remission in patients who fail to respond to front-line treatment. HDT with autologous stem cell transplantation has been extensively evaluated for breast cancer60; some promising phase 2 studies have shown a high response rate and improved PFS, but controlled trials have not demonstrated improved survival in patients with metastatic disease or in high-risk patients with local-regional disease. Promising results have been reported with high-dose chemotherapy and autologous transplantation for treatment of advanced ovarian cancer.61 Phase 3 randomized trials have not been performed.
Some patients with renal cell cancer have a marked antitumor response after nonmyeloablative allogeneic stem cell transplantation, indicating that a graft-versus-malignancy effect can occur with this disease,62 but most patients fail to have a durable response. Small studies of allogeneic HCT have been performed for other solid tumors.
Dose intensity is important for treatment of a broad range of pediatric solid tumors. Autologous HCT is commonly used to support HDT.63 Because these malignancies are relatively uncommon, large series and controlled studies are generally not feasible. Autologous HCT can improve survival in patients with high-risk or recurrent neuroblastoma.64 Allogeneic transplants have also been used with promising results in some studies. Autologous HCT has been widely used as a treatment option for Ewing sarcoma, osteosarcoma, pediatric germ cell tumors, central nervous system tumors including medulloblastoma, embryonal tumors and glial tumors, rhabdomyosarcoma, and retinoblastoma.
Complications of HCT
Pulmonary Complications
Pulmonary complications are frequent after autologous and allogeneic HCT. Patients may have preexisting lung damage due to prior chemotherapy/radiotherapy or can have a lung injury as a result of pretransplant conditioning regimens. Prompt treatment with corticosteroids is generally effective after ruling out other etiologies, particularly infection.65 Diffuse alveolar hemorrhage is a post-HCT complication that carries high mortality. Patients usually present with progressive dyspnea, hypoxia, and diffuse alveolar infiltrates.
Engraftment Syndrome
Engraftment syndrome is a syndrome of noninfectious fever plus any of the following symptoms: maculopapular skin rash, pulmonary infiltrates, or diarrhea.66 It usually develops approximately 10 to 15 days after HCT and is believed to be mediated by inflammatory cytokines. It is more commonly seen after autologous HCT than allogeneic HCT. Most patients respond to a short course of corticosteroids.
Hepatic Complications
Liver toxicity can be the result of damage due to conditioning regimens. The most dreaded complication is hepatic sinusoidal obstruction syndrome (previously termed hepatic venoocclusive disease), which usually occurs within first 3 weeks after HCT. Preexisting liver damage and a number of chemotherapeutic agents increase the susceptibility. The diagnosis is made clinically and usually involves a combination of increase in serum total bilirubin, hepatomegaly, and sudden weight gain.67 An ultrasound may show an abnormal portal vein waveform and an elevated resistive index. The pathogenesis involves endothelial cell injury that eventually results in progressive venular occlusion. Patients may recover with supportive measures, but patients with severe manifestations have a high mortality. No effective treatment has been established. Defibrotide is a promising agent in clinical trials for treatment of sinusoidal obstruction syndrome.68
Renal Complications
Acute kidney injury is a relatively common complication in patients undergoing allogeneic HCT. The incidence of acute kidney injury is higher after myeloablative conditioning allogeneic HCT compared with reduced intensity conditioning allogeneic HCT.69 Patients generally require treatment with nephrotoxic drugs such as calcineurin immunosuppressive drugs and frequently require foscarnet or amphotericin for treatment of infections. Other factors that increase the risk of acute kidney injury include development of sinusoidal obstruction syndrome, agents used for GVHD prophylaxis such as cyclosporine, and the development of acute GVHD. Occurrence of acute kidney injury increases short- and long-term mortality after HCT and also increases the risk of development of subsequent chronic kidney injury.
Cardiovascular Complications
Cardiac complications may be the result of high-dose chemotherapy or irradiation use in the conditioning regimens.70 Several other factors such as fluid overload and electrolyte imbalance during the early phase of transplant also increase the risk of cardiotoxicity. The prior cumulative dose of anthracyclines and their use within 60 days before HCT have been shown to increase the risk of cardiac complications. Cardiotoxicity due to high-dose cyclophosphamide use in preparative regimens is well known. Late mortality due to cardiac complications is also higher after allogeneic HCT compared with the general population. The cumulative incidence of arterial events, including cerebrovascular disease and coronary artery disease, can also be higher after allogeneic HCT. Preexisting risk factors such as diabetes mellitus, hypertension, and dyslipidemia increase the risk of late arterial events. Patients are generally screened for cardiac risk factors before undergoing HCT. Long-term transplant survivors should also be closely monitored for the late development of cardiovascular disease.
Graft-Versus-Host-Disease
GVHD is the major complication associated with allogeneic HCT. It is a clinicopathological syndrome that occurs when donor T cells react against disparate major or minor histocompatibility antigens expressed by recipient tissues. This reaction results in cytokine- and cell-mediated injury of the target tissues. Acute GVHD generally occurs within the first 100 days after transplant and typically involves the skin, liver, or gastrointestinal tract. The diagnosis is usually made clinically, supported by a biopsy of the involved tissue. Investigators have a considerable interest in identifying biomarkers to predict for the development of GVHD and to differentiate between the patients with GVHD and those with other diagnoses where the clinic presentation resembles GVHD.71 Once diagnosed, the severity is determined by the degree of involvement of each organ system.
Advanced GVHD is associated with considerable morbidity and mortality, and hence emphasis is placed on developing effective preventative strategies. Generally, combinations of immunosuppressive agents are used for GVHD prevention. The agents used most commonly include a combination of calcineurin inhibitor (cyclosporine or tacrolimus) and methotrexate.72 Other commonly used agents include sirolimus and mycophenolate mofetil. T-cell depletion is the most effective strategy for GVHD prevention but may be associated with increased risk of infections and disease relapse, and controlled studies have not shown improvement in overall outcome. Corticosteroids remain the mainstay for the treatment of acute GVHD.73 Second-line agents are used in patients who are refractory to corticosteroids, but they are generally less successful.
cGVHD has distinct clinical manifestations and typically occurs after 100 days following HCT. Limited cGVHD may be treated with localized therapy, such as topical corticosteroids. Patients with extensive GVHD should be treated with systemic corticosteroids.74 Other treatment options include extracorporeal photopheresis, calcineurin inhibitors, mycophenolate mofetil, pentostatin, or other immunosuppressive agents.
Infections
Infections related to pancytopenia may occur after high-dose chemotherapy, in persons with posttransplant immunodeficiency, and in persons who receive allogeneic transplants as a result of the effects of immunosuppressive therapy and GVHD. Patients undergoing HCT are susceptible to a host of bacterial, fungal, and viral infections. Antibiotic prophylaxis is recommended to prevent herpes virus, fungal, and pneumocystis infections. The Infectious Disease Society has issued detailed guidelines for preventing opportunistic infections after HCT (www.idsociety.org/Index.aspx). This area is complex in its own right and is out of the scope of this review.
Secondary Malignancies
The risk of developing secondary malignancies is higher in survivors of HCT compared with the general population.75 A wide range of secondary malignancies are seen; however, malignant neoplasms of the skin are most common.75 The presence of cGVHD particularly increases the risk of developing squamous cell carcinoma of the skin and oral cavity.76 Several studies have shown that patients who receive autologous HCT are at increased risk of the development of secondary MDS and AML.77 Posttransplant lymphoproliferative disorder may occur; this disorder is an Epstein-Barr virus (EBV)–driven process involving B cells, and it occurs in about 1% of HCT recipients.78 Risk factors include having an HLA-mismatched donor, use of antithymocyte globulin, cGVHD, and T-cell depletion. EBV lymphoproliferative disease can be effectively treated with rituximab and adoptive immunotherapy with DLI or EBV-specific cytotoxic T cells.