Eye, Orbit, and Adnexal Structures
Zeynel A. Karcioglu and Barrett G. Haik
Summary of Key Points
Incidence
• Primary ocular (eye) and ophthalmic (eye and adnexa) tumors are relatively uncommon.
• The most common primary ocular tumors are choroidal melanoma and retinoblastoma. The most common adnexal tumors are lymphoma, rhabdomyosarcoma, optic nerve glioma, and epithelial and melanocytic malignancies of the eyelid and conjunctiva, respectively.
• Many systemic neoplasms can involve the eye and adnexa, especially breast and lung cancers, as well as lymphoma and leukemia.
Etiology
• The etiology of most ophthalmic tumors is unknown.
• Retinoblastoma is the prototypical model of a genetically transmissible tumor via loss of a tumor suppressor gene.
• Squamous neoplasms of the eyelids and conjunctiva are associated with sun exposure, immunosuppressed states, and viral infections such as human papillomavirus and human immunodeficiency virus.
• Ocular and orbital metastases are frequently unexpected; approximately 25% of ocular metastases are discovered at an occult stage.
Diagnosis
• Intraocular tumors can be directly visualized, greatly facilitating diagnosis, but performing a biopsy is not easy and is limited to special circumstances.
• For intraocular tumors, a combination of funduscopic examination, intravenous angiogram, ultrasonography, and computed tomography/magnetic resonance imaging (CT/MRI) can yield diagnostic accuracies greater than 90% to 95%.
• Orbital and adnexal tumors are diagnosed by CT/MRI imaging and biopsy.
Treatment
• Intraocular tumors are treated with local modalities such as external beam irradiation, brachytherapy, and photocoagulation, with or without chemotherapy. If useful vision cannot be preserved in the eye affected by a tumor, the eye is enucleated.
• Orbital malignancies are frequently treated by excision and radiation/chemotherapy, but exenteration may be necessary in far-advanced sarcomas.
• Eyelid, conjunctival, and corneal malignancies are managed by local excision, with or without topical chemotherapy, cryotherapy, or irradiation.
• Metastatic malignancies may be palliated by external beam irradiation and adjuvant chemotherapy.
• Because radiation is widely used in treatment of ophthalmic malignancies, radiation toxicity is an exceedingly important issue in management protocols. Ophthalmic tissue components range from extremely radiosensitive tissues, such as the lens, to relatively radioresistant tissues, such as the retina and the optic nerve.
Introduction
A practical approach to a discussion of ophthalmic tumors—as with tumors of other anatomic regions—is to consider neoplastic conditions of the major structures with primary involvement: the globe, the conjunctiva and eyelids, and the orbit. Because the tumors and tumorlike conditions of these structures are exceedingly diverse, only the common entities are addressed in this chapter (Boxes 67-1 to 67-4). As with other oncologic subspecialties, the practice of ophthalmic oncology would be impossible without the use of radiation for imaging and therapeutic purposes.3–3 Furthermore, some of the idiopathic inflammatory conditions (Graves disease and pseudotumor of the orbit), which may masquerade as neoplasms, may also require radiation for diagnosis and treatment.4,5
Advances in medicine, particularly the ones with clinical applications, take a long time to develop. In our era of computer-generated medicine, however, this growth happens much faster than in the past. This chapter summarizes the management of the ophthalmic neoplasms commonly encountered in clinical practice and the specifics of radiation therapy and other recommended modalities. For discussion of rare ocular and periocular tumors, the reader is referred to other textbooks and review references.6–9 Noteworthy developments that have taken place in ophthalmic oncology practice since the last edition of this textbook was published in 2008 are summarized in the box at the end of the chapter.
Radiation Toxicity in the Eye
The eye is a complex organ composed of different types of tissues. Its different components range from extremely radiosensitive tissues, such as the lens, to radioresistant tissues, such as the retina and the optic nerve.10 The variations in radiation effect on the eye are dependent not only on tissue sensitivities but also on the methods of radiation delivery. Today, a majority of ophthalmic radiotherapy protocols involve the use of external beam radiation therapy (EBRT); indications for brachytherapy are fewer.1,5 Newer techniques of radiation delivery decrease the rates of ocular toxicity. For example, a combination of proton- and photon-accelerated fractionated radiation allows the delivery of high doses to advanced tumors of the nasal cavity and paranasal sinus with tolerable eye complication rates.11 Modern EBRT techniques such as stereotactic fractionated radiation therapy (SFRT) and photon EBRT appear to allow safe delivery of high target doses to patients with locally advanced orbital malignancies.12,13
External Eye
In the subacute period, ranging from 6 to 24 months, telangiectasias may develop in the skin and the conjunctiva, and the dryness worsens. The cornea demonstrates few structural changes until fractionated doses are in the range of 50 Gy; at this point, superficial punctate keratitis may develop. The cause of corneal damage is twofold: (1) the direct toxicity of ionizing radiation on its epithelial cells and stromal collagen and (2) developing dry eye syndrome. Total dose and dose per fraction are significant in terms of developing dry eye syndrome, leading to visual compromise in the radiotherapy of head and neck tumors.14
Punctate keratitis, which develops during the acute stages as a result of focal epithelial erosion and edema, becomes worse. Along with epithelial changes, the corneal sensation diminishes for weeks to months. During the subacute phase, scarring of the cornea may advance if deep keratitis was present during the acute period. Further scarring may lead to vascularization and thinning of the stroma, which in turn may lead to total opacification or perforation.15 Medical and surgical management of radiation keratopathy may be difficult because of poor wound healing. Moreover, patients treated with chemotherapy are also known to experience severe dry eye syndrome.
It would be an error to underestimate the consequences of the dry eye syndrome and to send the patient home with artificial tears and antibiotic or steroid eye drops or ointments. The management of dry eye should be undertaken jointly by the radiation oncologist and an ophthalmologist who are familiar with current treatment of dry eye syndrome, ranging from simple supplementation with eye drops to complex surgical interventions such as stem cell transplants and amniotic membrane grafts.16,17
The sclera, which maintains the structural integrity of both the anterior eye and posterior eye, consists of thick, irregular collagen bundles and is less affected by ionizing radiation than the cornea.18 No scleral radiation damage has been reported after EBRT at doses up to 60 Gy. However, extremely high scleral doses, which may be given during brachytherapy (e.g., in excess of 600 Gy), can cause melting of the sclera.19 In practice, strontium-90 (90Sr) and ruthenium-106 (106Ru) are more likely to deliver much higher scleral doses than is either iodine-125 (125I) or palladium-103 (103Pd) during plaque radiation therapy of intraocular tumors.20
Anterior Intraocular Components
The iris and ciliary body are composed of fibroblasts, smooth muscle, vessels, and columnar pigmented and nonpigmented epithelial cells. Many of these cells are reverting postmitotic cells and therefore are quite resistant to radiation. Iritis has been reported with a single dose of 10 to 20 Gy, but severe anterior uveitis is not observed until higher doses of 30 to 40 Gy (in 10-Gy fractions) are given, and then it appears only after 6 to 8 weeks. Radiation iritis may be followed by secondary glaucoma. Dry eye–related corneal ulceration also may exacerbate iritis and secondary iris neovascularization.21 Localized iris atrophy has been noted after brachytherapy for iridociliary melanomas but not after EBRT for orbital tumors.21
Among the anteriorly located intraocular tissues, the crystalline lens is uniquely susceptible to radiation injury. The lens is an avascular protein structure covered by an elastic tissue capsule. The anterior surface of the lens has a layer of epithelial cells that do not show much mitotic activity; these cells behave as reverting postmitotic cells. Near the equator of the lens, however, the epithelial cells divide regularly and thus behave as VICs. Formation of cataracts is the most frequently encountered delayed radiation effect in the mammalian eye. It is known that DNA damage and abnormal protein cross-linking lead to development of opacities within the lens protein; however, the exact mechanism of the DNA damage by radiation is not known.22 The best clinical description of radiogenic cataracts has been reported by Cogan and associates23 (Fig. 67-1).
The development of radiation opacity is dose, time, and age dependent (Fig. 67-2). Significant variation, however, is observed among persons who receive the same dose. The lenticular opacities do not always interfere with vision; clinically significant cataract development usually requires higher radiation doses and longer postradiation times. The latent period for production of cataracts from the time of exposure is on average 2 to 3 years but may range from 6 months to 3 decades.
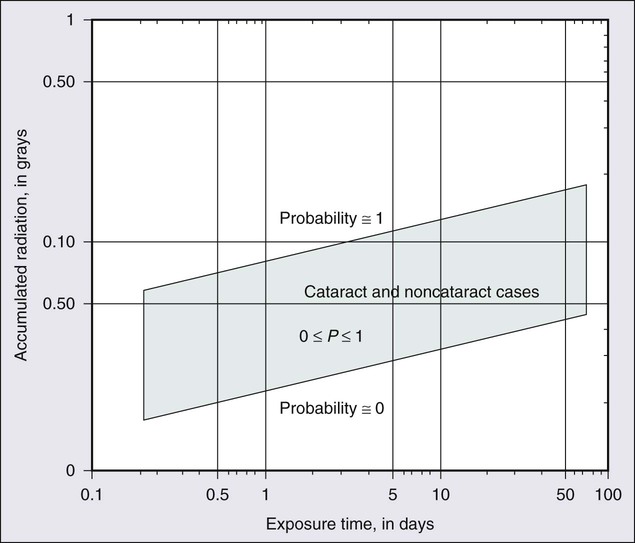
In the classic study by Merriam and Focht,24 a large series of patients undergoing EBRT for ocular and orbital malignancies was studied from the standpoint of cataract formation.24 The higher the absorbed dose, the shorter the latent period to cataract formation. Single doses of 2 Gy or fractionated doses of 4 Gy result in the formation of posterior lens opacities but rarely in significant visual impairment. The lowest cumulative ionizing radiation dose to the crystalline lens that can produce a cataract has been estimated also to be around 2 Gy. Recent literature indicates that this margin is substantially smaller than previously thought.25
A dose of 7.5 Gy invariably causes clinically significant cataract formation. Merriam and Focht concluded that a given dose of radiation becomes less likely to produce a cataract when it is fractionated over a longer period. Fractionation of the radiation dose delays the onset and decreases the incidence of cataract development and slows its progression, as does the use of partial and total shielding of the equatorial areas of the lens.26
After hematopoietic stem cell transplantation, Gurney and co-workers27 reported an incidence of cataracts of 36% at 15 years after the procedure; cataracts developed only in patients who received total-body irradiation as a conditioning regimen or head irradiation before transplantation. Of 4000 cancer survivors, 4% had cataracts.28 Cataracts develop as a result of total-body irradiation or prolonged corticosteroid use. Cataracts that develop after moderate-dose fractionated radiation often do not impair vision significantly enough to be removed.29
Another concern with radiation toxicity is the ocular exposure during modern imaging procedures. Although the great majority of computed tomography (CT) scanners deliver lower than the threshold dose for the development of cataracts, nevertheless, the potential exists for delivery of higher doses.30
It has been reported that comprehensive imaging procedures may result in radiation exposure up to local doses of 490 mGy. Although critical doses for organ damage (e.g., cataract formation or hair loss) are not reached, physicians need to be aware of possible radiation-induced complications, particularly with repetitive studies.31 For decades it has been known that in utero exposure to radiation, particularly during the first trimester, can result in cataract formation, as well as pigmentary degeneration of the retina or microphthalmia.32,33 In humans and experimental animals, even a low dose of radiation given with steroid treatment accelerates the development of lenticular opacities.34
Posterior Intraocular Components
The retina, choroid, and optic nerve are composed of relatively radioresistant tissues. At common therapeutic dose levels, acute effects of irradiation are rare; however, at doses higher than 50 Gy, acute retinal edema may occur, although this effect usually is transient (Fig. 67-3).
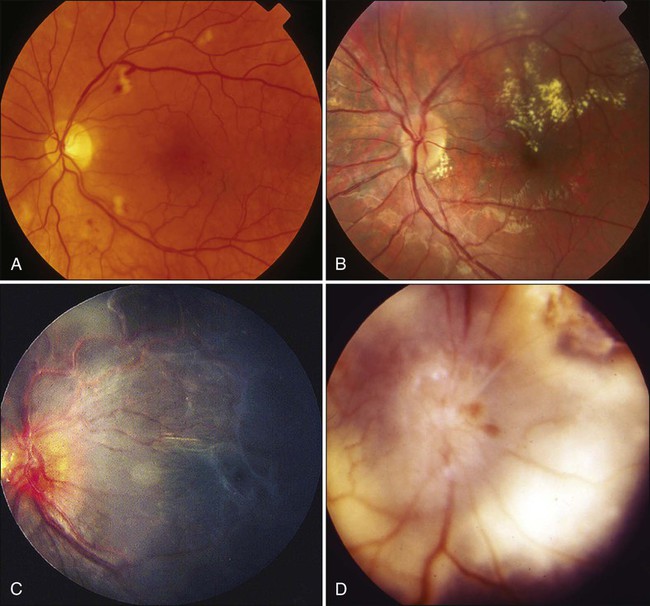
Chronic radiation damage to posterior ocular tissues is a form of microangiopathy. Because of the radiation damage to endothelial cells, vascular lumina are narrowed or obliterated and abnormal vascular permeability occurs. As a result of vascular disruption, the nerve fiber layer of the retina becomes ischemic, with the potential for the development of infarcts, exudates, and hemorrhages. The retinal vascular changes include totally occluded “ghost” vessels, vascular sheathing, microaneurysm formation, increased tortuosity, retinal telangiectasis, and eventually neovascularization (Fig. 67-3). The associated abnormal vascular permeability may impair vision as a result of macular exudates, often in a circinate pattern. Vascular occlusions of both the arterial and venous circulation may occur. Radiation retinopathy is a common vision-threatening complication following EBRT or brachytherapy. During the past two decades, most choroidal melanomas have been treated with plaque brachytherapy, which has increased the incidence of radiation retinopathy, with higher rates associated with larger tumors. When the damage involves the macula, serious visual loss occurs. Typically, onset of radiation retinochoroidal disease is between 6 months and 3 years after treatment; in some patients, however, the disease develops after much longer periods.35,36 Laser photocoagulation, photodynamic therapy, oral pentoxyphylline, and intraocular injections of anti-VEGF have been attempted as treatment modalities with unsatisfactory results.37
Both anterior ischemic optic neuropathy and posterior vascular occlusions can occur in the optic nerve, leading to visual loss (Fig. 67-3). Optic atrophy may be found as a result of ganglion cell degeneration or after a direct vascular insult. Radiation optic neuropathy can temporarily cause decreased vision, with later improvement over several months. This entity involves the anterior optic nerve and is characterized acutely by hyperemia and disc edema. Peripapillary hemorrhages and subretinal fluid also may be present. With EBRT, the mean total fractionated dose causing this effect is 55 Gy, with a range of 36 to 72 Gy. The mean latent period after radiotherapy is 19 months (5 to 36 months).38 The risk of toxicity increases significantly at doses over 60 Gy at approximately 1.8 Gy/fraction and at over 12 Gy for single-fraction radiation. Generally, the radiation tolerance is increased with a reduction in the dose per fraction.39 Current reports indicate that it is possible to detect the characteristics of radiation optic neuropathy quantitatively by optical coherence tomography (OCT).40
Eyelid and Orbital Tissues
The main eyelid changes associated with EBRT include acute erythema, depigmentation, atrophy, telangiectasias of the eyelid skin, and loss of eyelashes; lid malpositions and fibrosis of the puncta are also seen.41 Eyelid skin behaves like skin of other sites, but it is thinner. The first reaction is erythema (typically seen at 2 to 4 weeks after initiation of treatment), followed by dry and moist desquamation. Erythema usually is transient and subsides rapidly. The higher the radiation dose, the more quickly the erythema develops. Erythema increases during the first week and usually fades during the second week; it then may return 2 to 3 weeks after the initial insult and last for 20 to 30 days. The early reddening presumably is due to release of vasoactive amines. The second phase of erythema is due to vessel damage; thermographic studies demonstrate increased blood flow during the first 2 to 3 months. Desquamation usually is healed by the time treatment has ended because compensatory regeneration occurs in the basal layer of the skin. Moist desquamation is more common after doses of 50 to 60 Gy (in 1.8- to 2.2-Gy daily fractions) given over 5 to 6 weeks and also is more common where superficial lesions break the skin. Healing typically is slow and may take up to 4 weeks. No radiation-related scar usually results unless an unusually high dosage is used or a complication such as secondary infection occurs.
Doses in the range of 30 to 40 Gy can be delivered to the entire orbit without functional effect on the main lacrimal gland. On the other hand, evidence of histopathological atrophy of the lacrimal gland has been reported with single doses of 20 Gy and after 50 to 60 Gy given over a 6-week period.41 Although the mesenchymal tissues of the orbit including the extraocular muscles, fibroconnective tissue, and fat are rather resistant to radiation, orbital deformity leading to fascial asymmetry remains a major problem, particularly in children younger than 6 to 8 years who receive a tumor dose of approximately 50 Gy 41 (Fig. 67-4).
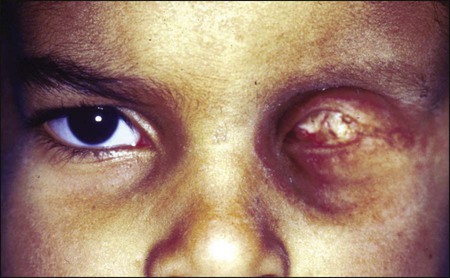
Another very serious adverse effect of orbital EBRT is that it increases the incidence of multiple primary malignancies in patients with retinoblastoma and other childhood malignancies.42–45 The relative risk of death was found to exceed the expected rates for malignant tumors of bone and soft tissues by 300-fold, for melanoma by 100-fold, and for brain tumors by about 25-fold.46,47
Hypothalamic and pituitary dysfunction with growth hormone deficiency with weight loss is also seen in children who undergo irradiation for optic nerve glioma.48,49
Intraocular Tumors
Uveal Melanoma
Uveal melanoma is the most common primary malignancy of the adult eye, but it constitutes only about 5% of all melanomas in the body.50 Up to 85% of ocular melanomas are uveal (primarily choroidal) in origin. The annual incidence of this tumor is approximately 4 per million population in the United States; this incidence is similar to that reported from European countries. The most recently reported male-to-female ratio is 4.9 to 3.7.50
Pathogenesis
Approximately 98% of cases of uveal melanoma occur in the white population. This racial predisposition to uveal melanoma has been explained on the basis of the susceptibility of white persons to the oncogenic effects of sunlight. Although this hypothesis is convincing for skin melanoma, the evidence with regard to uveal melanoma is conflicting.51 It is well known that intraocular melanoma develops as a result of the proliferation of uveal melanocytes, but the knowledge regarding this tumor’s molecular pathogenesis is rather limited. It seems conceivable that these cells are intrinsically resistant to apoptosis because of constitutive Bcl2 expression. Hypothetically, the main event in the neoplastic conversion of the uveal melanocyte appears to be the inhibition of the Rb pathway. Genetic alterations that subvert the Rb and p53 pathways probably occur early, allowing the altered melanocytes to reenter the cell cycle and proliferate. The proliferation of the melanocytes may then become arrested by other tumor suppressor mechanisms, resulting in a dormant nevus; most of the nevi are permanently arrested at this stage. For further growth to occur, activation of a “malignant switch,” such as a reinhibition of the p53 pathway, may be necessary subsequent to another genetic shift.52
During the past 15 years, considerable attention has been directed to the relevance of genetic testing of uveal melanoma, suggesting that the presence of chromosome 3 monosomy is a reliable predictor of worse prognosis.52,53