Cardiac Effects of Cancer Therapy
Haoyi Zheng, Boris Kobrinsky, Stuart Katz and James L. Speyer
Cardiomyopathy/Chronic Heart Failure
• Anthracyclines: cardiomyopathy/chronic heart failure occurs in 5% to 20% of patients receiving cumulative doxorubicin (more than 450 mg/m2); the incidence is higher in children
• Other antineoplastics (e.g., mitoxantrone and cyclophosphamide): the incidence of cardiomyopathy/chronic heart failure is less than 2%
• Trastuzumab (not common as a single agent; increased risk with anthracycline)
• Radiation therapy: cardiomyopathy/chronic heart failure is common 5 to 20 years after use of a single anteroposterior port
• Arsenic trioxide (a black box warning from the U.S. Food and Drug Administration [FDA])
• Vandetanib (a black box warning from the FDA)
• Nilotinib (a black box warning from the FDA)
• 5-Fluorouracil: 1% to 4.5% with infusion schedules
• Docetaxel, interleukins, interferons, tumor necrosis factor, cytokines, granulocyte-macrophage colony-stimulating factor, monoclonal antibody (CD20)
• Granulocyte-macrophage colony-stimulating factor
• Radiation therapy: effusions in 6% to 30% of patients receiving radiation therapy directed at the chest; constriction in 2% of patients receiving radiation therapy directed at the chest with current techniques
• Anthracycline-induced cardiomyopathy
• HER2 signaling pathway and decreased repair
• Radiation-induced cardiomyopathy
• Radiation-induced myocardial ischemia
• Decreased systemic vascular resistance
• Clinical cardiac symptoms—American Heart Association/American College of Cardiology staging, New York Heart Association classification, and Common Terminology Criteria for Adverse Events for Heart Failure
• Radionuclide scans—serial scans including baseline
• Endomyocardial biopsy—Billingham histopathological grading system
• Echocardiography—pericardial effusion/constriction, left ventricular systolic/diastolic dysfunction
• Cardiac magnetic resonance imaging—left ventricular systolic/diastolic dysfunction, myocardial fibrosis/necrosis detected by late gadolinium enhancement
Cardiomyopathy/Congestive Heart Failure
• Sodium and fluid restriction
• Angiotensin-converting enzyme inhibitors or angiotensin receptor blocking agents
Risk Reduction with Anthracyclines
• Anthracyclines: limit dose (e.g., doxorubicin: <450 mg/m2); use less-toxic analogs
• Unique delivery systems (e.g., liposomes)
• Pegylated liposomal doxorubicin
• Blocking agent: dexrazoxane (Zinecard)
Radiation-Induced Pericardial Disease
• Acute pericarditis: nonsteroidal antiinflammatory drugs, steroids
Introduction
Cardiac disorders are common complications of cancer therapy. A standardized grading system used for reporting adverse events in clinical trials (http://evs.nci.nih.gov/ftp1/CTCAE/CTCAE_4.03_2010-06-14_QuickReference_8.5×11.pdf) provides a detailed description of the categories of cardiac disorders associated with cancer therapy.1 The prevalence of cardiac disorders associated with cancer therapy is not well characterized because of limited follow-up time in many clinical trials and inconsistencies in the definitions of cardiac disorders used in the existing medical literature. This chapter proposes the following definitions based on the existing common terminology criteria for adverse events and the pathophysiological model of heart failure associated with cancer therapy presented in the next section.
• Elevation of serum troponin T or troponin I levels above the normal range
• Transient or persistent decrease in left ventricular ejection fraction (LVEF) of >10 percentage points from pretreatment baseline or to a value <55% (or less than the lower limit of normal for the laboratory)
• New myocardial fibrosis and scar detected by the late enhancement of gadolinium with cardiac magnetic resonance imaging (MRI)
• New onset or worsening of diastolic dysfunction detected by echocardiography or cardiac magnetic resonance (CMR)
• Evidence of new or unstable myocardial ischemia
• Symptomatic or asymptomatic arrhythmias (supraventricular and/or ventricular)
• Symptomatic or asymptomatic cardiac conduction system abnormalities
• Symptomatic or asymptomatic abnormal valve structure and/or function
Pathophysiology of Heart Failure
Clinical heart failure occurs when the heart is unable to provide a sufficient oxygen supply to meet the metabolic needs of the body at normal cardiac filling pressures. In the setting of cancer therapy, the pathophysiological process leading to clinical heart failure is initiated by an extrinsic cardiac injury due to radiation, anthracyclines, or other toxins with consequent focal or segmental myocyte death (mediated by necrosis and/or apoptosis). The extrinsic cardiac injury triggers an intrinsic pathological process (ventricular remodeling) characterized by activation of cell repair and regenerative pathways, myocellular hypertrophy, and interstitial fibrosis in the surviving myocardium. The signal transduction pathways that regulate these processes in the heart overlap with pathways for cell growth and proliferation that are known to be important in tumor biology. Initial cellular responses to cardiac injury serve to preserve cardiac output (and oxygen delivery) and therefore are not typically associated with clinical manifestations of heart failure. The progression to chronic heart failure is an indolent process that frequently occurs 10 to 20 years after the initial injury. The development of clinical manifestations of chronic heart failure represents the end stage of a largely occult disease process. With the introduction of modern therapy, there has been a substantial reduction in mortality for patients with systolic heart failure. However, symptomatic heart failure continues to confer a worse prognosis than the majority of cancers in the United States, with 1-year mortality rate as high as 45% in the elderly patient population.2
Cardiotoxicity associated with radiation and chemotherapy can be categorized according to the type and clinical manifestations of cardiac injury.3 Five types of myocyte injury have been proposed with different pathophysiological mechanisms (Fig. 59-1). Among these, type I is primarily associated with anthracyclines and is attributable to direct myocyte toxicity with consequent cell death mediated by either apoptosis or necrosis. Type II is primarily associated with trastuzumab and related agents and is attributable to inhibition of specific cardiac repair pathways that may lead to transient myocellular dysfunction or cell death. The notion that trastuzumab-related cardiomyopathy (type II) can be reversed has been challenged by recent studies.4 Because myocytes have a limited repertoire of response to injury, the long-term clinical consequences of all five types of injury follow a final common pathway of pathological cardiac remodeling and progression to heart failure, as previously described.
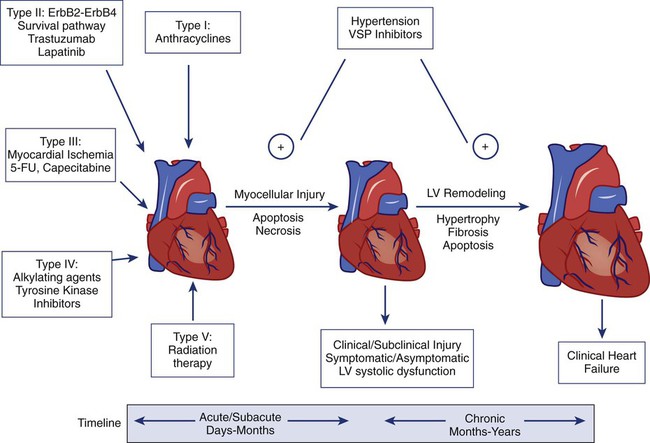
Anthracyclines (Type I)
The currently approved anthracyclines in the United States are doxorubicin (Adriamycin), liposomal doxorubicin and pegylated doxorubicin (Doxil), epirubicin (Ellence), daunorubicin (Cerubidine), and idarubicin (Idamycin PFS). Anthracyclines have played a major role in the treatment of hematologic malignancies and solid tumors, especially in the treatment of both metastatic and early breast cancer and sarcomas. Their dose-dependent cardiotoxicity is well documented, with the most common clinical presentation being dilated cardiomyopathy with chronic heart failure. Most available information on anthracycline-induced cardiomyopathy is derived from studies with doxorubicin and, to a lesser extent, with epirubicin and daunorubicin. However, other drugs of this class have also been associated with clinically indistinguishable cardiotoxic effects. The clinical presentations of cardiotoxicity can be acute, subacute, and/or chronic. No consensus exists for the definition of these terms. The previous definition for “acute” is the onset cardiac events within a few minutes after administration of anthracycline, which is mainly based on transient arrhythmias and rare cases of acute pericarditis and myocarditis.5 The elevation of troponin, new echocardiographic technology, and CMR allow the early detection of cardiotoxicity. We propose to define these terms as follows (Table 59-1).
Table 59-1
Clinical Presentations of Anthracycline-Induced Cardiotoxicity
Clinical Presentation | Time Course | Characteristics |
Acute | Within hours to 1 month of treatment; may be reversible after discontinuation of anthracycline | Elevated troponin, new onset of LV systolic and diastolic dysfunction, pericarditis, myocarditis, transient arrhythmias |
Subacute | Within 1 month to 1 year of treatment; may persist or progress after discontinuation of anthracycline | Dilated cardiomyopathy; rapid decline in LV function if treatment is continued after the onset of subclinical LV dysfunction |
Late onset | Years or decades after anthracycline treatment | Dilated cardiomyopathy; restrictive cardiomyopathy |
Data from Shan K, Lincoff AM, Young JB. Anthracycline-induced cardiotoxicity. Ann Intern Med 1996;125:47-58; and Bristow MR, Mason JW, Billingham ME, Daneils JR. Dose-effect and structure-function relationships in doxorubicin cardiomyopathy. Am Heart J 1981;102:709-18.
Acute Cardiac Toxicity
Acute cardiotoxicity may manifest as acute systolic dysfunction, arrhythmia, and/or clinical pericarditis-myocarditis during and/or soon after the administration of doxorubicin.6,7 These acute forms of cardiotoxicity are rare and usually transient or reversible. The most common form of acute cardiac toxicity is acute cardiomyocyte injury, which is associated with elevated serum troponin levels in the days or weeks after administration of anthracyclines. Elevation of troponin is associated with an increased risk for the development of chronic heart failure.8
Subacute and Chronic Cardiac Toxicity
Endomyocardial biopsy specimens have demonstrated that the dose-related cardiotoxicity associated with anthracyclines is biventricular. Retrospective studies indicate that the incidence of clinically recognizable heart failure with doxorubicin is 7% to 15% in patients who have received a cumulative dose greater than 450 mg/m2 to 500 mg/m2 without use of a cardioprotective agent (Table 59-2).9,10 Above this dose, the incidence of CHF rises steeply, with an estimated incidence of CHF of 50% when the cumulative dose of doxorubicin reaches 700 mg/m2. Cumulative cardiotoxic doses associated with the use of other anthracyclines vary, but the shape of the curve that plots cumulative dose against the incidence of CHF is comparable for all anthracyclines that have been studied. Careful surveillance for cardiotoxicity should be maintained from the start of therapy because isolated instances of CHF have been observed at lower cumulative doses.11
Table 59-2
Doxorubicin-Induced and Trastuzumab-Related Cardiotoxicity
Cardiotoxicity | Doxorubicin (High Dose; 350-400 mg/m2) | Doxorubicin (Very High Dose; 550 mg/m2) | Trastuzumab with Concurrent Use of Doxorubicin | Trastuzumab after Doxorubicin | Trastuzumab Alone |
Cardiotoxicity (clinical plus subclinical) | 18%-25% | 65% | 27% | 19% | 3%-7% |
Clinical heart failure | 3%-5% | 26% | 16% | 3%-5% | 1%-2% |
Prophylactic recommendation | Dexrazoxane | Dexrazoxane | None | None | None |
The incidence of CHF in clinical practice may be higher than that reported from retrospective trials.12,13 Prospective clinical trials indicate that the number of patients with evidence of CHF at cumulative doses of 450 mg/m2 may exceed 25%. Subclinical cardiotoxic effects of anthracyclines are likely present after each dose, with the likelihood of clinically apparent toxicity determined by the cumulative effects from prior doses of anthracyclines or from other co-morbid conditions. The natural history of the chronic anthracycline cardiotoxicity is highly heterogeneous. In its most virulent form, patients demonstrate refractory progressive symptoms despite optimal medical therapy with rapid onset of pump failure and death. Most patients present with permanent reduction in LVEF and persistent symptoms of CHF that progress slowly over time. In some patients, LVEF can improve over time, sometimes for years, with later recurrent deterioration of LVEF and eventually the appearance of CHF.
Risk Factors
The most important risk factor for cardiac toxicity is the total cumulative dose of anthracycline. Other factors associated with an increased risk of anthracycline-induced cardiotoxicity in adults include age greater than 70 years, exposure to ionizing radiation to the chest wall, prior exposure to anthracyclines, and preexisting cardiac disease or traditional cardiovascular risk factors including history of CHF, myocardial infarction, hypertension, and diabetes mellitus. Concomitant use of other agents with cardiotoxicity such as cyclophosphamide and paclitaxel also confer increased risk of anthracycline cardiotoxicity. Concurrent and sequential use of trastuzumab (Herceptin) with anthracycline-based adjuvant chemotherapy in patients with HER 2+ breast cancer significantly increases risk for cardiac toxicity (Table 59-2). The independent contributions of each of these factors (with the exception of trastuzumab) are not well defined, but having multiple risk factors appears to be associated with additive risk.
Heterogeneity in individual patient susceptibility to anthracycline cardiotoxicity suggests that genetic variation may also contribute to risk. Genetic variants in genes encoding proteins related to free radical metabolism, anthracycline transport, and drug biotransformation have been shown to be associated with increased risk in anthracycline-induced cardiotoxicity in both pediatric and adult populations of cancer survivors.16–16 These preliminary findings need to be further replicated and confirmed in larger prospective studies.
Mechanisms
The antitumor effects of anthracyclines are mediated by inhibition of DNA synthesis through direct interactions with DNA (intercalation) and inhibition of topoisomerase II (Top II).17 Top II has two isoenzymes in mammalian cells, Top IIα and Top IIβ. Doxorubicin or daunorubicin binds both DNA and Top IIα to form the topoisomerase IIα-doxorubicin-DNA ternary cleavage complex, which triggers cell death.18 These tumoricidal mechanisms do not likely contribute to cardiac toxicity because Top IIα is overexpressed in tumor cells and is not detectable in quiescent cells.19 Cardiomyocytes are terminally differentiated cells with very low rates of proliferation.
The primary mechanism for anthracycline cardiomyocyte injury was thought to be free radical damage to vital cell structures and subsequent cell death via apoptosis. Reduction of the quinone groups on the B ring of the anthracene structure results in a semiquinone radical before further reduction to the alcohol (e.g., doxorubicinol). Interaction of the semiquinone with oxygen yields superoxide anion (O2−). Dismutation of superoxide anion yields H2O2, which may react further with cardiac iron sources or the semiquinone to produce a reactive intermediate with the chemical characteristics of the hydroxyl radical. These reactions can proceed in either an iron-dependent or iron-independent fashion (Fig. 59-2).20 Doxorubicin also directly increases the labile intracellular iron pool by inhibiting iron regulatory protein–1 with consequent changes in transcription of transferrin receptors and ferritin.21,22 Formation of a Fe3+–doxorubicin complex can also enhance free radical generation via the Fenton reaction. Free radicals can cause damage at a variety of intracellular sites, including the nuclear envelope, cell membrane, mitochondria, DNA, and sarcoplasmic reticulum.23 Increased susceptibility of myocardial cells to free radical injury may be attributable to very low myocardial levels of catalase, one of major cellular enzymatic defenses against free radical injury, in cardiomyocytes when compared with other cells types. Doxorubicin also decreases levels of other antioxidant enzymes, including selenium-dependent glutathione-peroxidase-1 and cytosolic CuZn superoxide dismutase. Recently, Top II–mediated doxorubicin-induced DNA damage was shown to play an important role in cardiotoxicity. As previously mentioned, Top IIα is only expressed in proliferating and tumor cells and is the target of the antitumor effect of anthracyclines. Cardiomyocytes express Top IIβ, which is also a target for doxorubicin. Top IIβ-doxorubicin-DNA ternary complex can induce DNA double-strand breaks, leading to cell death. A recent study showed that cardiomyocyte-specific deletion of Top IIb (encoding Top IIβ) protects cardiomyocytes from doxorubicin-induced DNA double-strand breaks and transcriptome changes that are responsible for defective mitochondrial biogenesis and reactive oxygen species formation. Furthermore, cardiomyocyte-specific deletion of Top IIβ protects mice from the development of doxorubicin-induced progressive heart failure.24 Anthracyclines may also mediate cardiotoxicity through other iron-independent mechanisms related to changes in myofibrillar structure and function (see Fig. 59-2), changes in calcium and energy metabolism, and secondary changes in nitric oxide signaling in response to altered redox state in the myocardium.25
Diagnosis
Despite its limitations (discussed in the next section), serial measurement of LVEF remains the most commonly used method for detection of anthracycline cardiotoxicity. CHF may occur with preserved or decreased EF. In patients with preserved EF, heart failure is thought to be primarily attributable to changes in diastolic properties of the heart (increased diastolic LV wall stiffness and/or increased end-diastolic LV volume as a consequence of cardiac injury or overload). The classic clinical signs of congestion associated with heart failure (rales and edema) have both low specificity and low sensitivity. More than half of patients with documented extreme elevation of cardiac filling pressures do not present with rales or radiographic signs of pulmonary congestion. Accordingly, the clinical oncologist must maintain a high level of suspicion in patients receiving chemotherapy associated with potential cardiotoxicity. New onset of otherwise unexplained exertional dyspnea and/or fatigue may be the sole manifestation of heart failure regardless of change in LVEF. Unexplained sinus tachycardia at rest and after exercise in an otherwise oncologically stable patient is another potential early sign of cardiotoxicity. A system of staging anthracycline-induced hemodynamic changes in humans was developed by Bristow.26 Early referral to cardiology is recommended in the setting of suspected cardiotoxicity, even in asymptomatic patients, because accumulating evidence suggests that medical therapy may forestall disease progression. The relationship between staging criteria for heart failure developed by the American Heart Association, American College of Cardiology, and New York Heart Association27 in comparison with the Common Terminology Criteria for Adverse Events is summarized in Table 59-3.
Table 59-3
The Relationship between Staging Criteria for Heart Failure Developed by the American Heart Association, American College Of Cardiology, and New York Heart Association in Comparison with the Common Terminology Criteria for Adverse Events
ACC/AHA Staging27 | NYHA Classification | CTCAE* for Heart Failure | CTCAE* for LV Systolic Dysfunction | Clinical Toxicity Criteria, Version 2.0† |
Stage A; at high risk without structural heart disease or symptoms | Class I: no limitation of activities | — | — | — |
Stage B; structural heart disease without signs or symptoms | Class I | Grade I: asymptomatic with laboratory (e.g., BNP) or cardiac imaging abnormalities | — | Grade I: asymptomatic decline in resting LVEF of at least 10% but <20% Grade II: asymptomatic resting EF less than LLN for laboratory or decline of resting LVEF more than 20% of baseline value |
Stage C; structural heart disease with prior or current symptoms | Class II: mild limitation of activity Class III: marked limitation of activity |
Grade II: symptoms with mild to moderate activity or exertion Grade III: severe symptoms at rest or with minimal activity or exertion; intervention indicated |
Grade III: symptomatic due to drop in ejection fraction responsive to intervention Grade IV: refractory or poorly controlled heart failure due to drop in ejection fraction; intervention such as ventricular assist device, intravenous vasopressor support, or heart transplant indicated |
Grade III: CHF responsive to treatment |
Stage D; refractory CHF requiring specialized interventions | Class IV: confined to bed or chair | Grade III-V Grade IV: life-threatening consequences; urgent intervention indicated (e.g., continuous IV therapy or mechanical hemodynamic support) Grade V: death |
Grade: IV Grade V: death |
Grade IV: severe or refractory CHF |
*Adverse events are cardiac general and left ventricular systolic dysfunction; http://evs.nci.nih.gov/ftp1/CTCAE/CTCAE_4.03_2010-06-14_QuickReference_8.5×11.pdf.
†Adverse events are cardiovascular (general) and cardiac left ventricular function; http://ctep.cancer.gov/protocolDevelopment/electronic_applications/docs/ctcv20_4-30-992.pdf.
Routine Monitoring of Cardiac Toxicity
A commonly used strategy for patient monitoring is to obtain a baseline study before therapy commences and then obtain repeat studies at intervals as the cumulative dose increases, with more frequent observations at higher cumulative doses. Scans are examined for sequential changes in wall motion and global LVEF. Any decrease in LVEF, including those that remain within the “normal” range and those that decrease to clearly abnormal values, may indicate anthracycline myocyte damage.26,28 Careful monitoring with serial MUGA scans may permit treatment with greater cumulative doses of doxorubicin beyond the empiric stopping dose of 450 to 500 mg/m.
Endomyocardial Biopsy
Endomyocardial biopsy remains the gold standard for diagnosis because of its high sensitivity and specificity. Anthracyclines cause a unique pattern of histologic damage as originally demonstrated in animal models and confirmed by endomyocardial biopsies in patients.29 Billingham grade has been used as a histopathological scale for doxorubicin-induced cardiomyopathy (Box 59-1).29 A continuum of change is well described, from dilation of vacuoles to mitochondrial swelling, myofibrillar dropout, interstitial fibrosis, and, ultimately, cell death.31–31 The changes seen on endomyocardial biopsy appear to parallel clinical findings.32 The consistency of these findings in nonmammalian species has provided the basis for a number of animal models of anthracycline cardiomyopathy.33 However, endomyocardial biopsy is rarely used in clinical practice because of the risks associated with an invasive procedure, absence of data to demonstrate that the results of the test alter treatment approach or outcome, and the availability of noninvasive alternatives to monitor cardiac toxicity.
Early Detection of Cardiac Toxicity
Cardiac Imaging
Advanced echocardiographic techniques may be useful for earlier detection of cardiac toxicity. Diastolic dysfunction assessed by spectral Doppler echocardiography of mitral valve inflow patterns may be a sensitive method for early detection of cardiac dysfunction.34,35 Stress echocardiography using exercise or dobutamine has also been used to detect subclinical cardiotoxicity.36,37 Strain and strain rate assessment by speckle tracking echocardiography and tissue velocity imaging echocardiography are promising areas for the early detection of cardiac toxicity. In a recent study,38 tissue velocity imaging and strain allowed for early detection of subclinical cardiac dysfunction before conventional echocardiography in patients receiving adjuvant trastuzumab. Another study demonstrated that a decrease in left ventricular longitudinal strain assessed by speckle tracking echocardiography was an independent predictor of later development of cardiotoxicity in patients undergoing chemotherapy.39 Further study utilizing these new echocardiography-derived indexes is required to establish the value of these measures for routine clinical use.
CMR can be used to characterize myocardial tissue and evaluate the LV systolic and diastolic performance. CMR is known to differentiate transient and permanent myocardial injury in various systemic and inflammatory diseases and thus may be able to visualize myocardial tissue changes after chemotherapy before any measurable change in LVEF.40 The presence of late gadolinium enhancement (indicative of scar formation in the myocardium) was reported in patients with breast cancer with trastuzumab-related cardiomyopathy after doxorubicin-based adjuvant chemotherapy.39 The lack of late gadolinium enhancement detected by CMR might predict reversibility of impaired LV function in patients with chemotherapy-induced cardiomyopathy,3 but more confirmatory studies are needed.
Cardiac Biomarkers
Troponin I and troponin T are highly specific and sensitive biomarkers of cardiomyocyte injury from any cause. Measurement of serum troponin can detect chemotherapy-induced cardiac toxicity in its early stage, before the onset of impaired LV function.41 Elevated troponin at 3 days and 1 month after receiving anthracycline-based chemotherapy is a significant prognostic factor for development of a future cardiac event defined as a >15 percentage point reduction of LVEF and CHF.8,41 Elevated troponin has been observed in patients treated with standard doses of anthracycline and even after the administration of the first dose of doxorubicin.42,43 This observation is consistent with the concept that cardiomyocyte injury and/or cell death begins early in the course of anthracycline therapy. In patients with elevated troponin levels after chemotherapy with either a high cumulative dose of anthracycline or standard-dose anthracycline and trastuzumab, treatment with enalapril has been reported to reduce risk of cardiac events.44 Furthermore, in patients with HER2-positive breast cancer treated with trastuzumab after anthracycline-based adjuvant chemotherapy, elevated troponin might help distinguish between reversible and irreversible cardiac damage.45
Strategies to Reduce Risk of Anthracycline Cardiotoxicity
Risk Reduction
Empiric limitation of cumulative doses of doxorubicin to a range of 400 mg/m2 to 450 mg/m2 or to lower doses in patients with other clinical risk factors for cardiotoxicity is a common strategy that can reduce but not eliminate the risk of cardiotoxicity in all patients. The current maximum recommended dose for doxorubicin is 500 mg/m2, and for epirubicin it is 900 mg/m2. The risk and benefit must be carefully assessed for each person because limiting or discontinuing therapy prematurely may deprive patients from receiving the full therapeutic benefit of anthracycline-based chemotherapy. A combination of individualized dose restriction with careful cardiac monitoring may offer the safest practical approach to patients receiving doxorubicin.12
Dose Schedule Changes
Alterations in the dose schedule of anthracyclines are based on the hypothesis that chronic cardiac toxicity is related primarily to peak drug concentration, whereas the antitumor effect is more related to total drug exposure (concentration time, or area under the curve). Several studies have demonstrated decreased cardiac toxicity with prolonged continuous infusions (e.g., 24 to 96 hours) compared with standard rapid infusion schedules. A metaanalysis showed that bolus administration of anthracycline-based chemotherapy was associated with an odds ratio of 4.13 (confidence interval [CI]: 1.75-9.72) for the development of cardiac toxicity versus administration by continuous infusion.50
New Delivery System
Liposomal anthracyclines were designed with the intent of reducing the risk of cardiac toxicity while preserving antitumor efficacy. Pegylated liposomal doxorubicin consists of STEALTH technology-based liposomes containing doxorubicin in an aqueous core. Its stable pegylated cover protects the drug from enzymatic degradation with a dramatic increase in the half-life compared with conventional doxorubicin formulation. The small size of the liposome (100 nm) enables preferential drug penetration through compromised tumor vasculature and accumulation in tumors, thereby producing less systemic and cardiac toxicity. In a phase 3 study of 509 women with metastatic breast cancer, pegylated liposomal doxorubicin demonstrated comparable response rates and survival when compared with conventional doxorubicin with reduced toxicity. Cardiotoxicity (defined as a >20 percentage point reduction in LVEF within the normal range, or a >10 percentage point reduction in LVEF to below the normal range) occurred in 18.8% of patients in the conventional doxorubicin arm versus 3.9% of patients in the pegylated liposomal doxorubicin arm (hazard ratio 3.16, P < .001).46 The reduction in cardiotoxicity was most marked for cumulative doses in excess of 450 mg/m2.47,48
Analogs
Epirubicin is one of most commonly used anthracycline analogs. The results from studies designed to compare the cardiotoxicity between equimolar doses of epirubicin and doxorubicin have yielded inconsistent findings. A metaanalysis of five trials showed no evidence for a significant difference in the occurrence of clinical heart failure between the treatment groups (relative risk [RR] = 0.36; 95% CI 0.12-1.11; P = .07) with no difference in tumor response or overall survival.48 The magnitude of the risk reduction is high, and the lack of statistical significance could be attributable to lack of power as a result of a small number of CHF cases (3 among 521 patients randomized to epirubicin and 12 among 515 patients randomized to doxorubicin). No randomized trials have been conducted regarding the occurrence of combined clinical and subclinical heart failure (change in LVEF) in patients treated with epirubicin versus doxorubicin.
Dexrazoxane
Dexrazoxane (Zinecard), a cyclic derivative of edetic acid, is the only drug approved by the U.S. Food and Drug Administration (FDA) for the prevention of anthracycline-induced cardiotoxicity. The compound is a bisdioxopiperazine that is hydrolyzed intracellularly to form a bidentate chelator that is similar in structure to edetic acid; it effectively binds intracellular free iron and iron bound in anthracycline complexes, thereby preventing free radical generation (Fig. 59-2), which is considered to be the primary mechanism of the cardioprotective effect of dexrazoxane. However, as previously discussed, Top IIβ is expressed in cardiomyocytes and appears to be a critical component of the causal pathway for doxorubicin-induced cardiotoxicity. An in vitro study demonstrated that dexrazoxane degraded Top IIβ in cardiomyocytes, antagonized the formation of Top IIβ DNA cleavage complex, and reduced doxorubicin-induced DNA damage.49 These findings suggest that dexrazoxane may provide cardioprotection via multiple mechanisms of action. In the United States, the current indication for dexrazoxane is for prevention of cardiotoxicity in patients with breast cancer who receive a cumulative dose of doxorubicin greater than 300 mg/m2 or a cumulative dose of epirubicin greater than 550 mg/m2