Cancer of the Breast
Antonio C. Wolff, Susan M. Domchek, Nancy E. Davidson, Virgilio Sacchini and Beryl McCormick
• Breast cancer is the most frequently diagnosed cancer in women in the United States, accounting for an estimated 226,870 new cases (163,570 invasive cancers and 63,300 in situ carcinomas) and 39,510 deaths in 2012.
• In the United States, the age-specific incidence of breast cancer increases with age, to a lifetime risk of breast cancer of 1 in 8 (to 110 years of age); by age 40 years, approximately 1 in 203 women will have been diagnosed with breast cancer annually; at 60 years of age, the figure is 1 in 28 women.
• Incidence rates rose 21% from 1973 to 1990, but then began to decline; mortality rates stayed relatively constant until recently, when annual decreases were seen.
• A sharp decline in the incidence of breast cancer in the early 2000s followed a decrease in the use of postmenopausal hormone replacement therapy.
• Age, family history, and both endogenous and exogenous ovarian hormone exposure have an important effect on risk and have been incorporated into models that predict individual risk of breast cancer; diet, alcohol use, and other factors play a smaller role.
• Inherited mutations in BRCA1, BRCA2, PTEN, and TP53 play a role in the development of breast cancer and can be directly tested in individuals.
Biology and Estimation of Risk
• The expression of nuclear estrogen and progesterone receptors plays an important role in the differentiation and growth of normal breast epithelium and the response of breast cancer cells to hormonal therapeutics.
• ERBB2 (human epidermal growth factor receptor 2 [HER2]) is a growth-signaling molecule on the surface of normal breast cells that is overexpressed in approximately 20% of breast cancer tumors, contributing to growth autonomy and genomic instability.
• Molecular analyses have defined at least four biological subtypes of breast cancer, including the basal type, two luminal types (so-called luminal-A and luminal-B), and the HER2-positive type.
• BRCA1 and BRCA2 are tumor suppressor genes that play a critical role in the cellular response to DNA damage; inherited mutations in these genes are associated with an increased risk of breast cancer.
• Regardless of the criteria used by an individual physician and patient to define high risk, four possible actions may be taken, some of which can be used simultaneously: (a) enhanced surveillance, (b) behavioral modification, (c) chemopreventive strategies, and (d) prophylactic mastectomy or oophorectomy.
• Screening guidelines currently differ between organizations. The 2009 United States Preventive Service Task Force breast cancer screening guideline recommends biennial screening mammography for women ages 50 to 74 years and an individualized (rather than standardized) approach for women ages 40 to 49 years. The American Cancer Society continues to recommend yearly mammogram starting at age 40 years. The American Cancer Society also recommends that breast magnetic resonance imaging (MRI) be used in addition to mammographic screening in women with a lifetime risk of breast cancer greater than 20%.
• Microcalcification and soft-tissue density are the major indications for biopsy in mammographic screening; the mammographic abnormality with the highest rate of malignancy is a mass density with associated calcification.
• For patients with breast symptoms or palpable abnormalities, mammography characterizes the suspicious area, evaluates the remainder of the breast for occult lesions, and assesses the contralateral breast.
• Malignant breast masses classically are nontender and firm, with irregular borders.
• Diagnostic methods include fine-needle aspiration cytology, needle-core biopsy with ultrasound or stereotactic guidance, and excisional biopsy, with or without wire or tack localization.
Management of Noninvasive Disease
• Lobular carcinoma in situ (LCIS) is a nonpalpable lesion that usually is discovered with another indicator for biopsy; it is more common in premenopausal women and accounts for 30% to 50% of cases of carcinoma in situ.
• LCIS has a propensity for multicentricity and bilaterality; it is an indicator of risk of subsequent invasive breast cancer. The rare pleomorphic variant of LCIS seems to have a more aggressive behavior and is considered more as a precursor of invasive lobular cancer rather than a marker of breast cancer risk. Some groups advocate complete excision with free margins.
• Management of LCIS has shifted toward observation after biopsy rather than mastectomy; increasing evidence shows that tamoxifen should be considered as a preventive approach.
• The multifocal nature of LCIS makes margin clearance an unrealistic and unnecessary goal.
• Unlike LCIS, ductal carcinoma in situ (DCIS) is almost always first identified by mammography; the peak incidence is between 51 and 59 years of age, and it accounts for most of the increasing number of carcinoma in situ lesions diagnosed.
• DCIS is more likely to be localized to one area of the breast; thus, most patients are candidates for breast conservation. Tamoxifen should also be considered following lumpectomy and radiation to reduce the risk of another ipsilateral or a new contralateral event, invasive or noninvasive.
Management of Early Stage Breast Cancer
• Patients should undergo a complete history and physical examination.
• Hemogram and renal and hepatic function tests as well as serum alkaline phosphatase evaluation should be performed in all patients.
• Bilateral mammography is indicated for all patients, and other breast imaging (ultrasound and MRI) should be used as needed for each individual patient; other imaging studies are recommended only to evaluate specific signs or symptoms or in patients with locally advanced disease.
• Prognostic factors include pathological tumor size, hormone receptors, axillary nodal status, histologic subtype, tumor grade, and perhaps age.
• HER2 is a strong predictive marker and accurate determination of HER2 status identifies patients with T1cN0 tumors and above who should be considered candidates for adjuvant therapy with a trastuzumab-based regimen.
• Expression of estrogen receptor and/or progesterone receptor is accepted as a predictive marker for response to endocrine therapy.
• Multiple gene expression profiling assays have been developed as prognostic tests and two of them are undergoing further prospective testing as a predictive marker for therapy selection.
• The current American Joint Committee on Cancer TNM staging system takes into account the increasing use of advanced imaging and pathology techniques, such as sentinel node biopsy and immunochemistry; it also considers the number of involved nodes as a strong prognostic factor.
• Sentinel lymph node mapping is now the standard of care for many women with early breast cancer as it is more accurate and less morbid than axillary dissection.
• For patients with stages I and II disease, breast conservation and modified radical mastectomy are the major therapeutic options; for most patients, breast conservation is the preferred approach.
• Factors that affect local control in breast conservation include pathological margin involvement, extensive intraductal component, age, and the presence of multiple tumors.
• Adjuvant therapy with cytotoxic drugs or endocrine treatment, or both, is recommended for patients with node-positive disease; anti-HER2–targeted therapy should also be considered for HER2-overexpressing tumors.
• Pathological tumor size, hormone-receptor and HER2 status, histologic subtype, and nuclear grade are used to select patients with node-negative disease for adjuvant therapy.
• The results of the serial meta-analyses performed by the Early Breast Cancer Trialists’ Collaborative Group (EBCTCG) show that the survival benefits of adjuvant chemotherapy and endocrine therapy persist after 15 years of follow-up.
• Challenges that require resolution include identification of biological parameters that more precisely predict the natural history of disease and the response to systemic therapy; more effective treatments are clearly needed.
Management of Locally Advanced Disease
• Patients with locally advanced disease are a heterogeneous group, including those with T3N1, T0-3N2-3, and T4N0-3 disease.
• Multimodality therapy is recommended for virtually all patients with locally advanced disease; the sequence of systemic therapy, surgery, and radiation depends largely on the operability of the primary disease.
• For women with inoperable or inflammatory breast cancer (or both), preoperative chemotherapy is recommended, followed by surgery, radiation, and endocrine therapy, if appropriate. Patients with HER2-positive disease may benefit from addition of anti-HER2–targeted therapy. Preoperative endocrine therapy for patients with receptor-positive disease is also a reasonable option.
Management of Locally Recurrent Disease
• Local recurrence is an indicator of systemic relapse in most cases; an exception may be local relapse in a breast that has undergone conservation therapy.
• Selection of the treatment modality depends largely on the extent of local and regional failure and the presence of distant metastases.
• Surgical removal alone may be sufficient in some cases, but it often is combined with locoregional radiation and/or systemic therapy.
Management of Metastatic Disease
• Although a small percentage of patients with metastatic breast cancer achieve long-term disease-free survival, this stage of disease is generally not curable, and therapy is largely palliative.
• A wide range of systemic, local, and supportive therapies are available for the palliation of metastatic breast cancer. Primary breast surgery may be indicated in selected patients who are seen with stage IV disease.
• The selection of endocrine, cytotoxic, or biological therapy usually is based on disease-free interval, receptor status, HER2 status, site of metastasis, performance status, age, and previous exposure to systemic therapy.
• Anti-HER2 agents, such as trastuzumab, lapatinib, and pertuzumab, have changed the natural history of patients with HER2-positive metastatic breast cancer. Treatment with bisphosphonates or denosumab is indicated to minimize bone complications in patients with bone metastases.
Epidemiology
Incidence
Worldwide, breast cancer is the most common type of cancer among women.1 In the United States, approximately 163,570 new cases of invasive breast cancer, 63,300 in situ cases, and 39,510 deaths were expected in 2012.2 In women, breast cancer accounts for 29% of new cases of cancer and 14% of cancer deaths, second only to lung cancer as a cause of cancer-specific death. Approximately 1% of breast cancers occur in males and 90% of these are estrogen receptor (ER)-positive.3 Incidence rates of breast cancer decreased by 2% per year from 1999 to 2005, and then remained relatively stable from 2005 to 2008. The decline appears to be partly the result of a decrease in the use of postmenopausal hormone replacement therapy.4 Although incidence rates (all races combined) are substantially higher for women older than age 50 years, approximately 23% of breast cancers are diagnosed in women younger than age 45 years (Table 91-1).
Table 91-1
Risk of Breast Cancer in U.S. Women
Age Range (years) | Breast Cancer Risk |
30–40 | 1 : 227 |
40–50 | 1 : 68 |
50–60 | 1 : 42 |
60–70 | 1 : 28 |
Lifetime (to age 110 years) | 1 : 8 |
Data from www.cancer.gov/cancertopics/factsheet/Detection/probability-breast-cancer. Accessed 10/1/2012
Although breast cancer is the leading cause of cancer for women in the United States (overall incidence 121 per 100,000 women) followed by lung cancer (overall incidence 55.8 per 100,000 women), lung cancer was the leading cause of cancer death (40.1 per 100,000 women) followed by breast cancer (23.5 per 100,000 women) between 2004 and 2008.5 Around the world, there are large variations in incidence, mortality, and survival, which may be a result of several underlying complex factors, including age, ethnicity, diet, and lifestyles (including reproductive issues such as age at first birth and breastfeeding).1 Breast cancer is increasing in less-developed countries, supposedly related to changes in lifestyle factors. Most of our knowledge regarding specific risk factors has been derived from large observational studies in developed countries, such as the Nurses’ Health Studies (NHS)6 in the United States, and the “Million Women Study” in the United Kingdom (www.millionwomenstudy.org).7 Some of these risk factors are briefly summarized in the following sections.
Diet
Alcohol consumption is the best-established dietary factor associated with increased risk for breast cancer, although there is considerable interest in other dietary components.8 Initial studies correlated fat intake and decreased intake of fruit and vegetables with an increased risk for breast cancer, but more recent prospective studies have failed to confirm those observations.9–12 One randomized study (Women’s Healthy Eating and Living) in breast cancer patients of a low fat/high fruit and vegetable diet did not demonstrate a decreased risk of breast cancer recurrence.11 However, a second randomized study (Women’s Intervention Nutrition Study) did demonstrate a lower risk of recurrence with a decreased intake of dietary fat associated with modest weight loss in breast cancer survivors.12
Dietary phytoestrogens, such as those found in soybeans, have a chemical structure that is similar to that of 17β-estradiol and can bind to the ER to compete with estrogen. Their consumption may have a weak protective effect against breast cancer. More recent studies suggest an inverse association of soy intake and breast cancer in Asian populations (in which soy intake is generally high from birth) but not in non-Asian populations.13 Although there is significant interest in the association of vitamin D intake and breast cancer risk, there are insufficient data to draw conclusions regarding potential benefit at this time.14
Ionizing Radiation
The accumulated knowledge about radiation-related breast cancer risk in women derives mainly from epidemiological studies of patients exposed to diagnostic or therapeutic radiation and of the Japanese atomic bomb survivors. Low-dose radiation exposure to the breast is carcinogenic, and risk increases as a linear function of increasing dose. The risk is particularly high in the developing breast (i.e., before the age of 20 years), and is minimal for women exposed after menopause.15 Therapeutic radiation, particularly extended field radiation for Hodgkin lymphoma, has been associated with an increased risk of breast cancer. The age of exposure is important with the highest breast cancer risk seen in women undergoing mantle radiation around puberty.16
Exogenous Hormones
The NHS originally was set up to determine whether oral contraceptive usage was associated with an increase in breast cancer. An analysis of data from that study and more than 50 additional studies has confirmed that current users of exogenous hormones have a slightly increased risk of developing breast cancer.17 However, the mortality associated with this risk appears to be offset by a decreased risk of colon and ovarian cancer, such that there is no increase in overall cancer mortality associated with their use.6 The Women’s Health Initiative randomized study of hormone replacement therapy (HRT) in postmenopausal women demonstrated an increased risk of invasive breast cancer with the combination of estrogen plus progesterone therapy,18 but not with estrogen alone.19 Other studies have also demonstrated that breast cancer risk depends on type of HRT (estrogen alone vs. combination) and time of initiation (with an increased risk seen when HRT was started soon after menopause).20 Age-adjusted incidences of invasive breast cancer decreased in the United States between 2002 and 2003 by 7%, reflecting an absolute decrease of approximately 14,000 breast cancer cases (Fig. 91-1). This decrease was limited to women 50 years of age or older, occurred primarily in ER-positive tumors, and coincided closely with a dramatic decrease in the use of HRT following the publication of the Women’s Health Initiative results that demonstrated no cardiovascular benefit for HRT.4
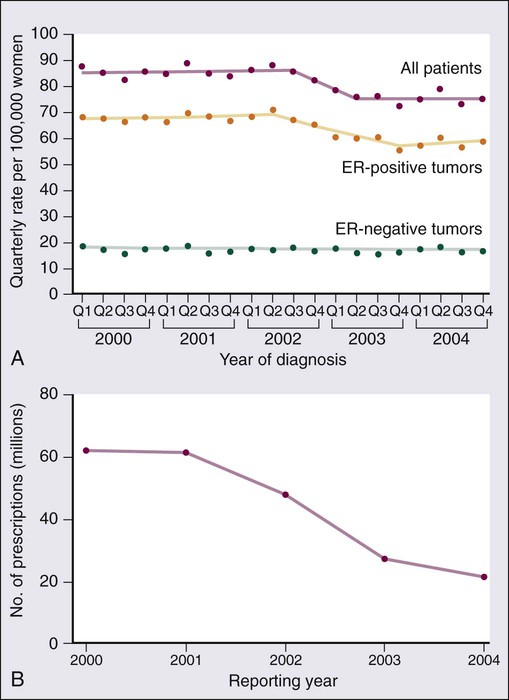
Reproductive Factors and Endogenous Hormones
Breast cancer risk consistently has been correlated with an earlier age of menarche, later age of menopause, nulliparity, and late age of first birth, all of which determine the cumulative number of ovarian cycles. This finding is consistent with the correlation between estrogen levels and breast cancer risk. In postmenopausal women, an increased risk of breast cancer has been demonstrated in those with estradiol levels in the highest quartile.21
Obesity and Body Habitus
There is a clear association between obesity and breast cancer risk. Women with a body mass index (BMI) of 25 to 30 (overweight) or BMI greater than 30 (obese) have been shown to have an elevated risk of breast cancer. In addition, an increased risk of breast cancer death has been seen with increasing BMI.22,23 The increased risk of breast cancer with obesity in postmenopausal women appears to be mediated, at least in part, by elevated endogenous estrogen and, possibly, insulin levels.24 An increased risk of breast cancer has also been associated with increasing height, with a relative risk of 1.17 (95% confidence interval [CI], 1.15 to 1.19) for every 10 cm of additional height.25
Familial History and Predictive Models of Breast Cancer Risk
Breast cancer is approximately twice as common among first-degree relatives of breast cancer patients as in those women with no family history of the disease. The two most important breast cancer susceptibility genes, BRCA1 and BRCA2, were identified by linkage analyses in the mid-1990s but account for less than 20% of familial clustering of breast cancer.26 Since then, rare germline mutations in several other genes, such as TP53 and PTEN, have been shown to confer a substantially increased risk of breast cancer. Given that linkage analyses have failed to yield additional susceptibility loci, it is likely that most of the remaining genetic susceptibility is a result of a number of moderate and low-penetrance alleles that coexist in highly penetrant combinations in a polygenic model, although some familial risk may still be a result of rare, undiscovered, high-penetrance gene mutations.27
Multiple moderate penetrance alleles including CHEK2, PALB2, ATM, BRIP, BARD, and others28 have been identified; mutations in these alleles are associated with a relative risk of breast cancer of 2 to 5. The clinical utility for testing for mutations in these genes is currently unclear. Recently breast cancer has been found to be a component of hereditary nonpolyposis colorectal cancer syndrome (HNPCC or Lynch) although the cumulative risk for breast cancer is less than for the classic component tumors (i.e., colon and uterine cancer).29
Apart from specific genetic susceptibility alleles, an increasing understanding of relevant risk factors has led to integrated efforts at evaluating a women’s individual risk,30 and several individualized risk-assessment tools are available, such as the Gail, Claus, Breast and Ovarian Analysis of Disease Incidence and Carrier Estimation Algorithm (BOADICEA), BRCAPRO, and Tyrer Cuzick models.31 One such tool is available on the National Cancer Institute (NCI) website at http://www.cancer.gov/bcrisktool/. These tools are being used for clinical counseling for healthy women perceived to be at risk and to determine candidates for chemoprevention with tamoxifen, raloxifene, and, most recently, exemestane. Given that for women living in the Western hemisphere the lifetime risk of breast cancer is approximately 11%, even a small relative risk reduction could translate into many thousands of lives saved.
Biology
Estrogen and Progesterone Receptors
Estrogen plays a key role in the development of both normal breast epithelium and breast cancer, and the modulation of estrogen levels is a key therapeutic modality for breast cancer. Estrogens interact with mammary epithelial cells via specific ERs that function as nuclear transcription factors.32 The two known receptors, ERα and ERβ, are encoded by different genes and share an overall sequence homology of approximately 30%, although the homology is higher in the DNA- and hormone-binding regions. ERα was the first ER to be isolated and is the receptor most closely associated with breast cancer; it is expressed mainly in the breast, uterus, and ovary. ERβ is more widely expressed and its relationship to breast cancer is less clear.33 Most of the literature that refers to ER (e.g., the staining of breast cancer cells as “ER-positive”) refers to ERα unless otherwise specified. The major ligand-dependent transcriptional activation activity (so-called AF-2) function of ER dislodges from a complex with heat shock proteins upon binding specific ligands, undergoes a conformational change, and homodimerizes. This homodimer then binds to the estrogen-responsive elements of the target genes, and recruits various coactivators (or corepressors) that further influence transcription.34 The participation in a multimeric signaling process likely contributes to the differential biological effects that estrogen and estrogen-related compounds (e.g., tamoxifen) can have in different target organs. ER also has a minor ligand-independent transcriptional activating function, which is modified by phosphorylation and can facilitate “cross talk” between ER activity and receptor tyrosine kinase signal transduction pathways. Approximately 70% of breast cancers express ER (i.e., they are ER-positive); these tumors tend to grow more slowly and appear better differentiated than ER-negative tumors. Moreover, antiestrogen therapy is effective both in preventing recurrence in the adjuvant setting and in prolonging survival once metastases have developed. However, the relationship between ER expression and cancer initiation is not clear, because ER-positive cells are nonproliferating in the normal breast epithelium that is found adjacent to proliferating cells, suggesting a paracrine effect. The paracrine effect hypothesis is further supported by evidence that even in most ER-positive tumors, only a minority of cells express ER by immunohistochemistry (IHC), and breast cancer stem cells do not express ER.
The progesterone receptor (PR), itself an estrogen-regulated gene, gives rise to two distinct isoforms, PRA and PRB, by alternative splicing. PRB appears to be more specific to breast cancer, whereas PRA is more widely expressed. Interestingly, a polymorphism in the PR promoter (+331 G/A) that increases transcription of PRB is associated with an elevated risk of endometrial and breast cancer.35 PR is variably expressed in ER-positive tumors, and this variability has prognostic relevance. ER-positive/PR-negative tumors occur more commonly in women older than 50 years, tend to be more aneuploid, and present as larger tumors with more frequent nodal involvement than ER-/PR-positive tumors. Furthermore, ER-/PR-positive tumors may be more likely to respond to antiestrogen therapy than ER-positive/PR-negative tumors.36
BRCA1, BRCA2, and Hereditary Susceptibility to Breast Cancer
It is estimated that 5% to 10% of all breast and ovarian cancer cases occur in the setting of inherited susceptibility because of a high-penetrance gene mutation, and the breast cancer susceptibility genes BRCA1 and BRCA2 have been identified as responsible for many of these cases.26 Women who carry a germline mutation in BRCA1 have a cumulative lifetime incidence of 50% to 85% of breast cancer and a 40% to 60% incidence of ovarian cancer, although estimates are somewhat lower in population-based series.26,37 There are a large number of BRCA1-interacting proteins including BARD, BRIP1, ABRAXAS, PALB2, RAD50, NBS1, and BRCA2. Germline mutations in the genes coding these proteins have also been associated with an increased risk of breast cancer, although the risk is significantly lower than with germline mutation in BRCA1 and BRCA2.38
The BRCA1 complex is integral in maintenance of genome integrity through roles in DNA damage repair, cell-cycle regulation, transcriptional regulation, and chromatin remodeling.39,40 Tumors that arise in heterozygote carriers of germline mutations usually have an inactivated wild-type allele and do not express a functional protein. Cells that lack BRCA1 or BRCA2 protein have a specific deficiency in the repair of DNA double-strand breaks by the conservative and relatively error-free mechanism of homologous recombination by gene conversion.40,41 Evidence for this defect is further supported by the finding that BRCA2 functions as part of the Fanconi anemia complex and actually is FANCD1. Fanconi anemia complementation group FA-N results from biallelic mutations in PALB2 (“partner and localizer of BRCA2”),42,43 whereas monoallelic mutations in the same gene are associated with an increased susceptibility to familial breast cancer.44 Moreover, several additional genes function in the same DNA repair pathway where germline mutations carriers are at increased risk of developing breast cancer—CHEK2, ATM, NBS1, RAD50, and BRIP1.45 Why these genes predispose primarily to breast and ovarian cancers remains unclear, and somatic mutations in them are not commonly found in sporadic breast cancers.
BRCA1-associated tumors usually are of the basal type (so-called triple negative: HER2-, ER-, and PR-negative). Conversely, sporadic triple-negative breast cancers may share phenotypic similarities with cancers arising in BRCA carriers.46 BRCA1 pathway dysregulation or “BRCAness” does also appear to be common in high-grade epithelial serous ovarian cancer.47,48 The identification of these genes and their function has important implications for prevention, diagnosis, and therapy of breast cancer in carriers and may have implications for sporadic ovarian cancer and, potentially, certain subtypes of triple-negative breast cancers.
Clinical management of carriers with BRCA1 or BRCA2 mutations is geared primarily toward preventing both ovarian and breast cancer.49 Prophylactic mastectomy, albeit an invasive procedure with cosmetic consequences, is the most effective strategy available; it is associated with a 90% reduction in the risk of breast cancer. Oophorectomy, in addition to reducing ovarian cancer risk by more than 80%, reduces the risk of breast cancer by 50%. Risk-reducing surgery may reduce the mortality for breast and ovarian cancer.50 Additionally, tamoxifen has been shown to reduce the risk of contralateral breast cancer by approximately 50% in both BRCA1 and BRCA2 carriers.51
Once cancer is diagnosed, therapy is dictated by standard prognostic and predictive features and no specific regimens are indicated on the basis of genetic predisposition at this time. The involvement of BRCA proteins in DNA repair by homologous recombination suggests that these tumors would be particularly sensitive to chemotherapies that induce DNA interstrand cross-links such as platinum salts. This hypothesis is supported by in vitro observations in both BRCA-null and FA cell lines. Significant responses to preoperative chemotherapy with cisplatin have been seen in a small number of BRCA1 mutation carriers52; however, it appears that breast cancers in BRCA1 mutation carriers are generally more chemosensitive compared with sporadic tumors.53 Finally, poly (ADP-ribose) polymerase 1 (PARP1) is involved in the base excision repair pathway; when this repair pathway is inhibited, double-strand breaks form at replication forks, and these are lethal to cells that cannot repair those breaks. Accordingly, BRCA-null cells are exquisitely sensitive to PARP inhibitors.54,55 Phase I and phase II studies have demonstrated exciting activity in BRCA1/2-related breast and ovarian cancer.56,57 It is hoped that more efficacious, less toxic treatment may be tailored to tumors that lack functional BRCA proteins.
TP53
TP53 (p53), also termed the guardian of the genome, is the most frequently mutated gene in human cancer. It plays a central role in sensing genotoxic and nongenotoxic stresses and transducing an antiproliferative effect (cell-cycle arrest or apoptosis) in response. It is activated and regulated by posttranslational modifications to the N-terminal region (e.g., phosphorylation and ubiquitination) and, binding as a tetramer to specific DNA sequences via a central DNA-binding core region, exerts its primary biological function by modulating the transcription of dozens of genes.58 In transgenic mice, loss of p53 is associated with multiple spontaneous tumors, although not of the mammary glands.59 However, p53 loss accelerates the appearance of mammary tumors in murine mammary tissue that also overexpresses MYC, HER2, IGF1, and/or WNT1. These genetic studies are consistent with a role for p53 loss late in tumor development.60 Indeed, the transgenic restoration of p53 function leads to tumor regressions.61
Inheritance of a p53 mutant allele causes the rare familial Li-Fraumeni syndrome, characterized by multiple early-onset cancers, including breast cancer in women who survive childhood cancers. Breast cancers in individuals with germline p53 mutations are frequently ER- and HER2-positive.62,63 Thirty percent to 50% of sporadic cases of breast cancers harbor somatic mutations in p53, based primarily on sequencing of exons 5 through 8 (the DNA-binding core), where approximately 90% of mutations are found. Currently, p53 mutations that have been observed in breast cancers are listed in the p53 database maintained by the International Agency for Research on Cancer.
Mutant p53 accumulates in the nucleus of neoplastic cells. Thus initial studies that described only a weak association between aberrant p53 and adverse prognosis in breast cancer relied on IHC detection; more recent analyses based on mutation detection have confirmed a strong association.64 Recently, p53 mutations from 1794 European patients with at least 10 years of follow-up were analyzed. Mutations in exons 5 through 8 were more common in ductal and medullary tumors, tumors with an aggressive phenotype (high-grade, large size, node-positive cases, and low hormone receptor content), and in women younger than 60 years. Furthermore, the presence of a mutation conferred an overall 2.27-fold increased relative risk of breast cancer-specific mortality, independent of other known prognostic markers (e.g., tumor size, node status, and estrogen and PR expression).65 Finally, although not all mutations confer the same biological properties (e.g., missense vs. nonmissense), all have similar prognostic utility.
Initial attempts at correlating p53 expression (by IHC) with resistance to chemotherapy have been supplanted by studies directly evaluating mutations. Some of these studies show breast cancers with p53 mutations to be associated with less benefit from both antiestrogens66,67 and anthracylines,68,69 although conflicting results also are reported.70 An overall analysis is complicated by different p53 assay methods, different disease settings (adjuvant, preoperative, and metastatic), and different combinations of drugs. In contrast, it appears that no clear relation between aberrant p53 and sensitivity to paclitaxel exists.73–73
p53 is an obvious target for cancer therapy, despite the apparent intractability of a loss-of-function genotype. Two approaches are being taken to overcome this limitation—small molecules to restore p53 function74 or inhibit its interaction with MDM2,75 or adenoviral-mediated gene delivery. Although the latter approach is approved for therapy of head and neck tumors in China, no clear activity in breast cancer has been demonstrated to date.76
ERBB2 (HER2)
The protooncogene ERBB2 (HER2) encodes a transmembrane tyrosine kinase receptor, one member of a family of related growth factor receptors that include EGFR (ERBB1), ERBB3 (HER3), and ERBB4 (HER4). The ligands for these receptors, termed heregulins, are a family of growth factors known to bind to ERBB3 and ERBB4, inducing heterodimerization with ERBB2 and subsequent transduction of downstream signals. Because HER2 does not bind ligand directly,77 the ability to heterodimerize with other family members that do bind ligand is crucial for its activity. Of these, HER3 appears to be the most important heterodimerization partner.78
The amplification and/or protein overexpression of HER2 is found in 20% of newly diagnosed breast cancers, and is associated with a more aggressive clinical course and decreased survival time compared with tumors with normal levels of HER2.79 Amplification leads to very high levels of protein expression and constitutive activation of the receptor tyrosine kinase signal transduction pathway, namely RAS-MEK and AKT,80 although the downstream effects may be more pleiotropic than originally believed.81 In contrast to EGFR, in which amplifications in lung cancers frequently harbor activating mutations in the kinase domain, such mutations have not been found frequently in breast cancer (see the Sanger Centre’s COSMIC database, http://www.sanger.ac.uk/genetics/CGP/cosmic/). The identification of HER2 as an oncogene in breast cancer facilitated the development of therapies directed against it. Trastuzumab, a humanized monoclonal antibody that binds to the extracellular domain of HER2, induces clinical responses as a single agent, prolongs survival in combination with chemotherapy, and decreases recurrence rates and improves survival in the adjuvant setting when given to women whose cancers overexpress HER2.84–84 Lapatinib, an oral, small-molecule tyrosine kinase receptor inhibitor active against HER2, and pertuzumab, a monoclonal antibody that inhibits dimerization between HER family members, have also been shown to be of benefit in metastatic, HER2-positive breast cancer. However, not all women with HER2-positive breast cancer respond to these agents, and studies aimed at elucidating mechanisms of resistance are providing further insight into the pathophysiology of HER2-positive breast cancer. For example, increased levels of insulin-like growth factor–1 receptor (IGF1R) may bypass HER2 blockade by trastuzumab and allow growth factor activation of AKT,85 and a similar outcome may result from PTEN loss, as discussed earlier. In addition, a truncated form of HER2 that lacks the extracellular domain but retains the kinase activity has been described to correlate with trastuzumab resistance.86
The amplicon containing HER2 frequently contains additional genes that influence therapeutic efficacy. Although initially it was thought that HER2-positive disease is more sensitive to anthracyclines, recent data suggest that this sensitivity is conferred by the topoisomerase II gene (TOP2A) when it is present in the amplicon.87 Modern genome-wide analysis methods currently are being applied to panels of cell lines,88 as well as to tissues from patients treated with anti-HER2 agents,89 to better understand mechanisms of resistance. The insights gained, in turn, are being exploited for further drug discovery. Both antibody and small molecule inhibitors of IGF1R as well as phosphatidylinositol 3-kinase (PI3K) and AKT kinase inhibitors currently are in clinical development for breast cancer, and synergy with anti-HER2 therapy has been demonstrated in vitro.90
PI3K and PTEN
Phosphatidylinositol-3,4,5-triphosphate (PIP3) is a lipid second messenger formed by PIP3 kinase (PI3K) in response to receptor tyrosine kinase signaling. PIP3 activates AKT and other related pathways to induce proliferation and inhibit apoptosis. Approximately 25% of human breast cancers harbor oncogenic activating mutations in the p110α catalytic subunits of PI3K (PIK3CA).91 This signal is inactivated by the opposing phosphatase action of the tumor suppressor gene PTEN.
Germline PTEN mutations cause a hereditary cancer predisposition syndrome known as Cowden syndrome, characterized by a high incidence of breast, uterine, thyroid, and skin neoplasms.92 PTEN is inactivated in a wide variety of human tumors as a result of either mutation or, more commonly, epigenetic silencing through methylation, including in breast cancer.93 PTEN loss is associated with genetic instability, and primary breast tumors that lack PTEN have increased aneuploidy.94 In addition, PTEN loss has been associated with a decreased likelihood of response to anti-HER2 therapy with trastuzumab.95 Recent data elucidating the relationship between presence of PIK3CA mutations and PTEN status show that they are inversely correlated. Furthermore, PIK3CA mutations are twice as common in HER2-positive or ER-positive tumors, suggesting that more than one aberration upstream of AKT may be necessary to overcome the effect of an intact PTEN.96 The relevance of PIK3CA mutations to the clinical activity of anti-HER2-directed therapy by trastuzumab and lapatinib is under active investigation.97
Breast Cancer Genome
The elucidation of genetic aberrations in cancer genomes (mutations, deletions, and amplifications) has led to a better understanding of cancer pathophysiology and is providing targets for rational drug development. With the determination of the DNA sequence of the normal human genome, efforts have shifted toward a more comprehensive analysis of cancer genomes. The first report of a comprehensive sequence analysis of 21,000 of the best studied and annotated human genes was published, including data from 11 breast and 11 colon cancer samples.98 This screen rediscovered cancer genes known to be associated with breast cancer (e.g., p53 and BRCA1) as well as genes not previously characterized as associated with cancer. This impressive effort suggests that an individual breast cancer harbors an average of about 90 mutant genes, of which a minority (approximately 12) are expected to contribute to the neoplastic process. Unfortunately, the functionally important mutations often differ among tumors (i.e., no tumor had more than six mutated cancer genes in common with any other tumor). However, when the genes were analyzed by functional groups, mutations in signal transduction pathway and transcription factor genes were found in nearly all breast tumor samples. As detailed below, rapid advancements in sequencing technology and bioinformatics have greatly expanded the information available.
Molecular Profiling in Breast Cancer
Simultaneous genome-wide determination of the relative abundance of RNA species (i.e., transcriptional profiling) combined with novel bioinformatic approaches has led to the development of molecular classifications based on continuous expression ranges of thousands of genes (as opposed to a binary determination of discrete factors, i.e., ER/PR and HER2). Tumors can be classified either according to transcripts that tend to segregate together according to underlying biological differences (unsupervised clustering) or sorted by a given end point such as prognosis or response to therapy (supervised clustering). The first of these studies identified an “intrinsic gene list” of 534 genes that defined five biological subtypes of breast cancer including the basal type (HER2-positive, cytokeratins 5 and 17 positive), the “triple negative” type (HER2-, ER-, and PR-negative), two luminal types (so-called luminal-A and luminal-B), and the normal-like type (Fig. 91-2).99 Studies by others have confirmed large-scale gene expression differences between ER-positive and ER-negative tumors, and have shown additional molecular subsets within these categories.100 This molecular classification of breast cancer is robust in that it is observed regardless of microarray platform type, and the various subtypes correlate with overall prognosis.101,102 Luminal-type cancers have the most favorable long-term survival, whereas HER2-positive and basal-like cancers may be more sensitive to chemotherapy but have the worst overall prognosis. Thus breast cancer is not a single disease with heterogeneous ER and HER2 expression but appears to be composed of three to five molecularly and clinically distinct subtypes.
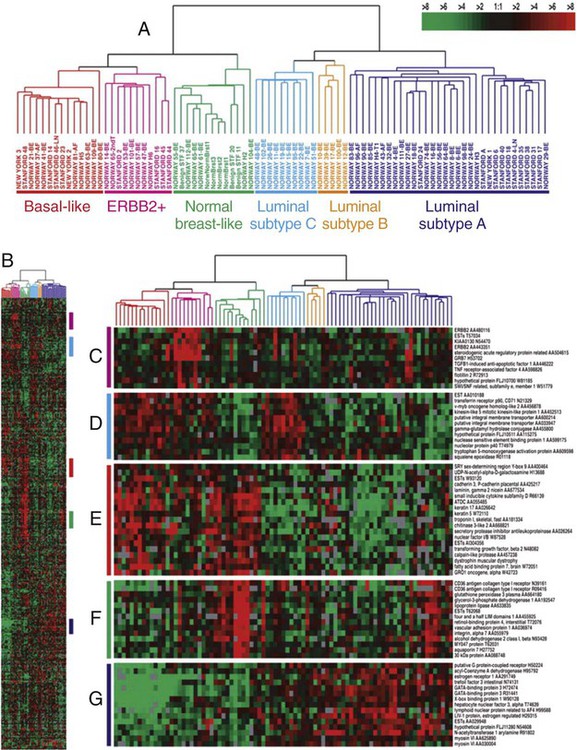
A similar approach can be taken by assaying DNA amplifications and deletions across the genome.103 Such data recently have been mapped against both the “intrinsic gene set” and clinical outcome data, and have the potential to identify high-level DNA amplification that, similar to HER2, may be useful as therapeutic targets.104 However, it should be noted that although these classifications are robust across a population, there is no standardized assay of measurement for assigning a molecular class to a new individual case. Furthermore, much of the molecular heterogeneity overlaps with conventional histopathology and is captured by determination of ER, PR, HER2 status, and tumor grade.100
Several multigene prediction scores that correlate with outcome and provide more accuracy than traditional histopathological markers have been retrospectively derived from microarray data, and, in some cases, prospectively validated on additional datasets. The best known examples are a 70-gene predictor set (Fig. 91-3)105,106 and a 21-gene predictor set,107,108 and both are commercially available. The 21-gene test consists of 16 target transcripts and five reference transcripts, including ER, PR, and HER2, and a “proliferation” group of five genes (which includes Ki-67 and cyclin B1); and a carefully constructed weighted formula is used to determine the overall “recurrence score.”107,108 Although this recurrence score provides a continuous risk variable, for clinical use it is pared down to three categories of low, intermediate, and high (of note, given the weighting schema, it is unlikely that a HER2-positive tumor would be classified as “low risk”). It is interesting that these two gene sets as well as the “intrinsic gene set” described earlier and yet another “wound healing” signature all seem to perform similarly when tested against the same clinical data set, even though they share few to none of the same genes.109 The ability of the 70-gene assay to determine which ER-positive node-negative patients are most likely to benefit from adjuvant therapy is being prospectively tested in the Microarray in Node-Negative Disease May Avoid Chemotherapy (MINDACT NCT00433589) trial. Similarly, the Trial Assigning Individualized Options for Treatment (Rx) (TAILORx) NCT00310180) study, which is testing the utility of adjuvant chemotherapy in patients who have an “intermediate risk” according to the test, has completed enrollment; low-risk patients received hormonal therapy only, whereas all high-risk patients were treated with both chemotherapy and hormonal therapy. The RxPONDER study (NCT01272037) is addressing a similar question in patients with node-positive disease, where patients with a recurrence score <=25 are being randomized to endocrine therapy alone or also receive chemotherapy. In the meantime, the Oncotype assay has been incorporated into the National Comprehensive Cancer Network (NCCN) algorithm for node-090 negative, ER-positive breast cancer patients.
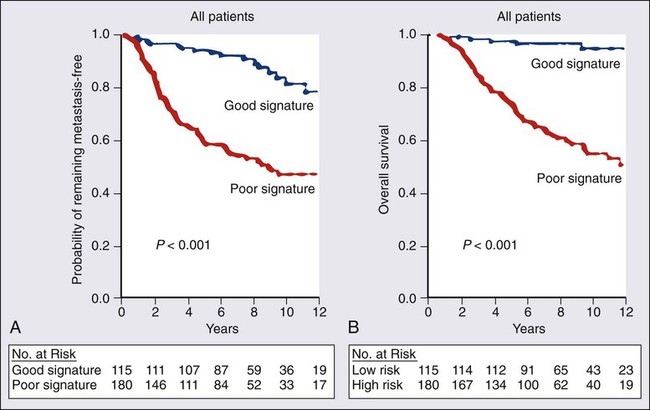
Comprehensive Genomic Analysis
More recently, comprehensive approaches have been undertaken to examine the molecular profiles of breast cancer. A comprehensive and integrated analysis of primary breast cancers by genomic DNA copy number arrays, DNA methylation, exome sequencing, messenger RNA arrays, microRNA sequencing and reverse-phase protein arrays (The Cancer Genome Atlas or TCGA) confirmed the existence of four main subtypes of breast cancer, and provided a wealth of data regarding genetic and epigenetic information for potential therapeutic targeting.110 In addition, the ENCODE project (Encyclopedia of DNA Elements) has recently mapped regions in non–protein-coding DNA of transcription factor association, chromatin structure, and histone modification that will no doubt enhance our understanding of breast cancer.111
Disseminated and Circulating Tumor Cells
From 20% to 45% of patients with primary operable breast cancer and 70% of patients with metastatic disease have disseminated tumor cells (DTCs) that can be detected in bone marrow112 or in lymph nodes.113 Historically, DTCs usually have been evaluated by IHC staining for various cytokeratin markers. Although their existence has been associated with a worse prognosis,112 the need for a relatively invasive procedure (a bone marrow biopsy) and the lack of sufficient specificity and sensitivity, as well as method standardization, have prevented the routine use of such information in clinical decision making.114 However, it is now possible to measure circulating tumor cells (CTCs) accurately by using an automated immunobead enrichment followed by pancytokeratin staining. Using this approach, patients with metastatic breast cancer and five or more CTCs per 7.5 mL of blood are predicted to have a significantly worse disease-free survival (2.1 months vs. 7 months) and overall survival (8.2 months vs. 18 months) than patients with fewer CTCs.115 This measurement may enable routine, minimally invasive assessment of disease progression and response to therapy. Furthermore, the ability to isolate CTCs allows the study of molecular markers such as HER2 amplification and their relationship to the primary tumor in real time. This approach has the potential not just to monitor patients but also to select specific therapy based on a molecular diagnosis obtained at the time of therapy (as opposed to relying on the original diagnostic biopsy). The clinical utility of CTCs is now being prospectively tested in a trial randomly assigning patients with 5 or more CTCs per 7.5 mL after 1 month of therapy to continue the same course or change systemic therapy.