Brain Metastases and Neoplastic Meningitis
Penny K. Sneed, Norbert Kased and James L. Rubenstein
• Central nervous system metastases are common, affecting as many as 25% of patients with cancer.
• Most central nervous metastases involve the brain.
• Less often, the dura, leptomeninges, skull base, or cranial nerves may be affected.
• The terms “neoplastic meningitis” and “carcinomatous meningitis” refer to the dissemination of cancer cells within the leptomeningeal space.
• The most frequent primary tumor types that give rise to brain metastases include lung cancer, melanoma, breast cancer, and renal cell carcinoma.
• Brain metastases are best detected with contrast-enhanced magnetic resonance imaging.
• Metastases generally appear as enhancing, well-circumscribed lesions with or without surrounding vasogenic edema.
• Biopsy or resection may be indicated to confirm the diagnosis, particularly in a patient with a single lesion and no cancer diagnosis or no known metastatic disease.
• Neoplastic meningitis often eludes early detection; meningeal enhancement is only visible on magnetic resonance imaging in about 50% of cases, and cerebral spinal fluid cytology may be negative initially in 40% to 50% of cases.
• The most important factors that predict longer survival of patients with brain metastases include age <65 years, good performance status, control of the primary cancer, and lack of extracranial metastases.
• The most standard treatment for brain metastases is whole-brain radiotherapy (WBRT).
• Patients with a good prognosis and a limited number of brain metastases may benefit from more aggressive therapy such as surgery (especially for a single brain metastasis) or stereotactic radiosurgery (SRS), with or without adjuvant WBRT.
• After WBRT alone, the following observations have been made:
At least 60% of symptomatic patients improve significantly.
Median survival time is typically 3 to 6 months.
One third to one half of patients die of brain metastases, and the remainder die of systemic disease.
Approximately one quarter of brain metastases have a complete response and one third have a partial response.
One-year actuarial local control probability may be as low as 14% or as high as 71%.
• Among patients with newly diagnosed brain metastases selected for surgery or SRS with or without WBRT, the following observations have been made:
Median survival time is approximately 9 to 11 months.
One-year actuarial local control rates are approximately:
85% for surgery and adjuvant WBRT.
75% to 90% for SRS with or without adjuvant WBRT.
The increased local control achievable with surgery plus WBRT or with SRS may only be meaningful in patients likely to live at least 6 to 12 months from the standpoint of their extracranial disease.
Systemic therapy is not generally used as a primary treatment for brain metastases, but it has some efficacy on its own.
There is increasing interest in combining systemic agents with radiation therapy.
• Intrathecal chemotherapy plays a major role in the management of neoplastic meningitis, alone or in combination with radiotherapy.
• Craniospinal radiotherapy causes significant acute toxicity and long-lasting myelosuppression.
• Accordingly, neoplastic meningitis may be managed with use of intrathecal chemotherapy combined with more limited radiotherapy.
• Examples of limited radiotherapy include the following:
Lumbar fields for gross disease in the cauda equina
Skull base fields for cranial nerve involvement
WBRT in patients with hydrocephalus or significant headache stemming from diffuse brain leptomeningeal involvement
Brain Metastases
Epidemiology
Brain metastases appear to be more common than primary malignant brain tumors, although the exact incidence is unknown. In a Memorial Sloan Kettering Cancer Center autopsy series, 24% of patients with cancer had CNS metastases and 15% had brain metastases.1 One population-based study in the Netherlands found that brain metastases developed in 8.5% of 2724 patients with melanoma, lung, breast, colorectal, or renal cell carcinoma. The 5-year cumulative incidence of brain metastases was 7.4% in patients with melanoma and 16.3%, 9.8%, 5.0%, and 1.2% for patients with lung, renal cell, breast, or colorectal carcinoma, respectively.2 Similarly, the population-based incidence of brain metastasis among patients with cancer within the Metropolitan Detroit Cancer Surveillance System diagnosed between 1973 and 2001 was 6.9% for patients with melanoma and 19.9%, 6.5%, 5.1%, and 1.8% for patients with lung, renal cell, breast, and colorectal cancer, respectively.3 The incidence of brain metastases appears to be increasing as a result of improvements in cancer management that are leading to longer survival time, as well as increased lesion detection with contrast-enhanced, high-resolution magnetic resonance imaging (MRI),4 although Schouten et al.2 found no evidence of increasing brain metastasis incidence during 1986 through 1995.
The most common primary tumors responsible for brain metastases include lung cancer (constituting approximately 40% to 50% of cases), breast cancer (15%), melanoma (10%), and unknown primary (5% to 10%), followed by renal cell carcinoma, colorectal cancer, gynecologic cancers, and other miscellaneous tumors.5,6 Brain metastases may arise from any primary cancer, but certain tumors such as melanoma and carcinomas of the lung, kidney, and breast have a tendency to spread to the CNS. On the contrary, some tumors rarely metastasize to the brain, such as prostate, oropharyngeal, and skin carcinomas. In children, the most common solid malignancies responsible for brain metastases include sarcomas, neuroblastomas, and germ cell tumors.7
Cancer may spread to the brain at various points in the course of disease. Synchronous brain metastases, found within 1 month of the primary cancer diagnosis, occur in up to one third of patients.2 More commonly, brain metastases are discovered after the diagnosis of cancer, often after other systemic metastases have developed, at a median of less than 1 year after lung cancer diagnosis and 2 to 3 years after diagnosis of melanoma, breast cancer, gynecologic cancer, or renal cell carcinoma.6 Overall, the median time from primary diagnosis to diagnosis of brain metastases is 12 months.6
Pathophysiology
Brain metastases arise primarily from arterial hematogenous spread to the brain. Tumor cells tend to become trapped where blood vessels decrease in caliber at the gray/white matter junction and distal-most vasculature (the border or “watershed” zones between arterial territories).8 Metastatic cells then adhere to the endothelial cells, penetrate into the brain parenchyma, and proliferate. Larger aggregates of tumor cells that gain access to the venous circulation are filtered out in lung capillaries before entering the systemic arterial circulation, but individual tumor cells may pass through to lodge in the brain. Tumor emboli may also break off from lung metastases or primary lung cancers to travel to the brain via the arterial circulation. Cells from pelvic or abdominal cancers may gain access to the posterior fossa or leptomeninges through Batson’s vertebral venous plexus without passing through the lungs.5 Intracranial spread may also occur via direct extension from bone or dural metastases or perineural extension along cranial nerves. The distribution of metastases is roughly proportional to the relative blood flow to different regions; approximately 80% of brain metastases are located in the cerebral hemispheres, 10% to 15% in the cerebellum, and 1% to 5% in the brain stem.5,6 Posterior fossa metastases appear to arise disproportionately from pelvic or abdominal primary tumors.5
Most brain metastases are very well circumscribed. Although extensive associated edema may be present, the tumor cells do not tend to infiltrate into surrounding brain tissue, in contrast to primary malignant brain tumors. Most brain metastases are solid, but they may appear to be heterogeneously enhancing or cystic because of necrosis, keratin deposits in squamous cell carcinoma, or mucin secretion from adenocarcinomas. Brain metastases may be hemorrhagic, particularly from melanoma, renal cell carcinoma, choriocarcinoma, and, less frequently, bronchogenic carcinoma.9
Single brain metastases are more common than multiple metastases in patients with renal cell, gastrointestinal, or unknown primary cancers.6 In the era of computed tomographic (CT) imaging, it appeared that about 50% of patients with brain metastasis had a single brain lesion.5 More recently, in the era of MRI, this number has decreased to approximately 25% to 35%, with improved sensitivity for detecting smaller metastases.10 Of note, the term “solitary brain metastasis” implies that a single brain metastasis is the only known site of metastatic disease.
Clinical Presentation
The possibility of brain metastases should be suspected in any patient with cancer who experiences new neurologic signs or symptoms. Two thirds of patients with cancer found to have brain metastases at autopsy had experienced neurologic symptoms from the metastases,11 and only 10% of a series of 729 patients diagnosed between 1973 and 1993 by CT or MRI were asymptomatic.6 The most common presenting symptoms are headache (24% to 53%), focal weakness (16% to 40%), altered mental status (24% to 31%), seizures (15% to 16%), and ataxia (9% to 20%).6,12 Symptoms may worsen gradually from a growing tumor and associated edema. Less often, acute neurologic symptoms may occur as a result of hemorrhage of a brain metastasis.
Diagnosis
It is widely accepted that MRI is the best diagnostic test to detect brain metastases. Standard imaging includes T2-weighted and pre- and postgadolinium-enhanced T1-weighted sequences; a postcontrast fluid-attenuated inversion-recovery sequence is also helpful in visualizing small metastases near cerebrospinal fluid (CSF) spaces (Fig. 50-1). Gadolinium-enhanced MRI is much more sensitive than nonenhanced MRI or contrast-enhanced CT imaging.13,14 In one study, 17 of 55 patients (31%) with a single metastasis based on contrast-enhanced CT were found to have multiple metastases on contrast-enhanced MRI.15 The sensitivity of MRI is improved by using “triple-dose” gadolinium (0.3 mmol/kg instead of 0.1 mmol/kg gadoteridol)16 and by using contiguous axial 3-mm slices and coronal three-dimensional spoiled gradient echo recovery volume imaging so that small lesions are not missed between slices. Functional imaging techniques such as positron emission tomography, magnetic resonance spectroscopy, and perfusion and diffusion MRI may aid in distinguishing metastatic lesions from necrosis, primary brain tumor, or nonmalignant processes.19–19
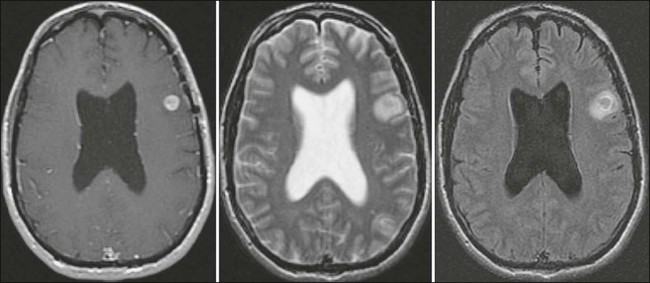
The differential diagnosis of an enhancing or hemorrhagic intracranial lesion includes brain metastasis, primary brain tumor, CNS lymphoma, abscess, encephalitis, cerebral infarct or hemorrhage, progressive multifocal leukoencephalopathy, tumefactive demyelinating disease, and radiation necrosis. Factors that aid in making a diagnosis based on imaging include characteristic appearance, a known cancer diagnosis, and a multiplicity of lesions. However, a biopsy may be warranted if there is doubt about the diagnosis or if a single brain lesion is seen in a patient with a history of cancer but no other known metastatic disease. In a study requiring biopsy or surgery before whole-brain radiotherapy (WBRT), 11% of 54 patients with a single brain lesion that was thought to be a metastasis turned out to have glioblastoma, low-grade astrocytoma, abscess, or an inflammatory process.20 A biopsy series in 100 patients with multifocal brain lesions and no known primary cancer found a variety of diagnoses: malignant glioma (37%), primary CNS lymphoma (15%), brain metastases (15%), low-grade glioma (12%), infectious processes (10%), and ischemic lesions (6%).21
Prognostic Factors
In general, brain metastases are associated with a poor prognosis. In the pre-CT era, the median survival time of patients with symptomatic brain metastases was approximately 1 to 2 months without treatment,24–24 2 to 2.5 months with corticosteroid therapy,25 and 3 to 6 months with WBRT.28–28
Despite major advances in cancer diagnosis, cancer treatment, and brain imaging, the overall survival time of unselected patients with brain metastases treated with WBRT has remained at 3 to 6 months since the 1950s.6,29–31 The majority of patients with brain metastases have or will soon experience disseminated systemic disease, and overall survival is often determined by the extent and activity of the extracranial disease. In patients with a relatively short life expectancy, the treatment goal is to achieve rapid palliation and a neurologic symptom-free remission interval commensurate with the life expectancy. However, long-term survival or even cure is possible in a small proportion of patients with brain metastases, and patients with a good prognosis from the standpoint of their extracranial disease may benefit from more aggressive therapy to achieve durable control of their brain metastases.
Prognostic factors have been evaluated in several large series. Lagerwaard et al.32 studied 1292 patients with CT-diagnosed brain metastases treated between 1981 and 1990. The overall median survival time was 3.4 months. The most important parameters prognostic for longer survival included better performance status, limited systemic tumor activity, and a normal serum lactate dehydrogenase level, followed by breast cancer versus other primary sites, age <70 years, and one to two versus three or more brain metastases.32
Gaspar et al.33 identified three prognostic groups by using a recursive partitioning analysis (RPA) of more than 1100 patients enrolled in three consecutive Radiation Therapy Oncology Group (RTOG) trials conducted from 1979 through 1993. The median survival time was 7.1 months for RPA class 1, consisting of patients <65 years old with a Karnofsky performance status (KPS) ≥70, controlled primary tumor, and no extracranial metastases. Patients with KPS <70 (RPA class 3) had a median survival time of only 2.3 months; the median survival time was 4.2 months for the remaining patients (RPA class 2) (Table 50-1).33 The prognostic value of the RTOG RPA classes has been validated in patients treated with stereotactic radiosurgery (SRS) ± WBRT or surgery ± WBRT, with median survival times of 10.9 to 25.4 months for RPA class 1, 5.9 to 9.9 months for RPA class 2, and 1.5 to 8.9 months for RPA class 3 (Table 50-1).34–39
Table 50-1
Median Survival Time by Radiation Therapy Oncology Group Recursive Partitioning Analysis Class
RPA Class 1 | RPA Class 2 | RPA Class 3 | ||||
Treatment | n | MST (mo) | n | MST (mo) | n | MST (mo) |
WBRT alone33 | 236 | 7.1 | 765 | 4.2 | 175 | 2.3 |
SRS alone initially35 | 23 | 25.4 | 74 | 5.9 | 20 | 4.2 |
SRS alone initially36 | 15 | 13.4 | 65 | 9.3 | 21 | 1.5 |
SRS alone initially34 | 39 | 14.0 | 197 | 8.2 | 29 | 5.3 |
SRS + upfront WBRT34 | 64 | 15.2 | 222 | 7.0 | 9 | 5.5 |
Surgery + WBRT37 | 26 | 14.8 | 63 | 9.9 | 36 | 6.0 |
Surgery ± WBRT38 | 26 | 10.9 | 69 | 9.8 | — | — |
Surgery ± WBRT39 | 50 | 21.4 | 208 | 9.0 | 13 | 8.9 |
Sperduto and colleagues40 developed a newer prognostic index for patients with brain metastases termed the Graded Prognostic Assessment, based on a database of 1960 patients accrued to several RTOG brain metastasis protocols. Subscores of 0 to 1 for each factor (age, KPS, number of metastases, and extracranial metastases) were summed to determine a Graded Prognostic Assessment score ranging from 0 to 4 (worst to best prognosis). This system was subsequently validated and refined with diagnosis-specific prognostic indices based on an independent, multi-institutional retrospective analysis of 4259 other patients with brain metastases treated with WBRT and/or SRS.41 Prognostic factors included KPS, age, presence of extracranial metastases, and number of brain metastases for patients with lung cancer; KPS and the number of brain metastases for patients with melanoma and renal cell cancer; tumor subtype, KPS, and age for patients with breast cancer; and only KPS for patients with gastrointestinal cancer.
Treatment
Corticosteroids
Corticosteroid therapy is generally instituted in symptomatic patients as soon as brain metastases are diagnosed to help alleviate symptoms until the brain metastases and edema improve from specific antitumor treatment.42 Corticosteroids reduce the permeability of leaky tumor blood vessels and thereby reduce the mass effect and edema caused by brain metastases.43 The most commonly used steroid is dexamethasone because of its relatively low mineralocorticoid activity; often, a loading dose of 10 mg is used, followed by 4 mg every 6 hours. Lower doses (2 to 4 mg twice daily or 2 to 4 mg three times a day) may be adequate in many situations. Patients usually improve within hours after the first dose, attaining maximal benefit after approximately 3 to 7 days. After patients become asymptomatic or reach maximal benefit, the dose should be gradually tapered and either discontinued or else maintained at the lowest dose level needed to manage symptoms. If headaches recur or neurologic symptoms worsen during the course of the taper, the dose should be increased as needed and then the taper should proceed more gradually. Occasionally patients experience steroid-withdrawal symptoms of depression, fatigue, nausea, or poor appetite, necessitating reinstitution of low-dose dexamethasone or hydrocortisone and a slow taper in the low-dose range.
Anticonvulsant Agents
Seizures are very unlikely to occur from infratentorial metastases, but they may be triggered by supratentorial metastases. About 15% of patients with brain metastases present with seizures when they first are seen by a clinician, and 30% to 40% experience seizures at some point in their disease course.44 An anticonvulsant agent such as phenytoin, carbamazepine, or levetiracetam should be prescribed for any patient with brain metastases who experiences a seizure. Prophylactic anticonvulsant agents are not recommended; prospective and retrospective studies and metaanalyses have failed to demonstrate a benefit for prophylactic anticonvulsant agents in patients with brain metastases.43–46 In addition, anticonvulsant agents may have adverse side effects or cause serious allergic reactions such as Stevens-Johnson syndrome. Importantly, certain anticonvulsant agents such as phenytoin can reduce the efficacy of corticosteroids and can activate the cytochrome P450 enzyme system.47 This latter property can affect patients undergoing chemotherapy by altering the metabolism of some chemotherapeutic agents, thus requiring chemotherapy dose adjustment.
External Beam Radiation Therapy
The most standard treatment for brain metastases consists of WBRT covering the entire intracranial contents while the patient’s eyes are shielded (Fig. 50-2). The benefits of WBRT were first described in the 1950s and 1960s. In these early studies, significant symptomatic improvement was noted in about 60% of patients, and the median survival time ranged from about 3 to 6 months with WBRT28–28 compared with an expected median survival time of 1 to 2 months without treatment.24–24
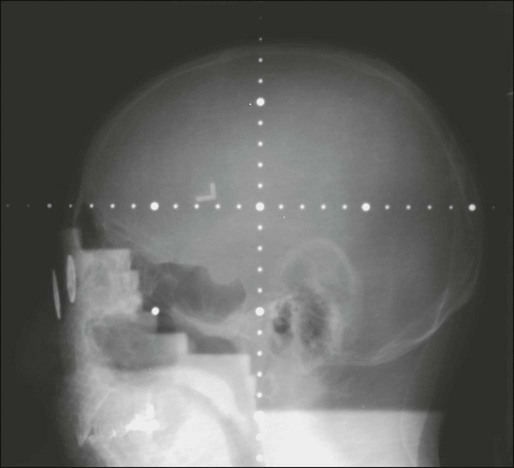
Selected Randomized Trials of WBRT Alone
Multiple large phase 3 randomized trials of WBRT have been conducted by the RTOG since 1970 (Table 50-2). The first two trials compared various WBRT fractionation schemes in more than 1800 patients treated from 1971 through 1976 and gathered a wealth of data.29,48 Complete or partial response of specific neurologic symptoms was observed in 60% to 90% of symptomatic patients; 47% to 52% of patients improved to a higher neurologic function class; the median duration of improvement was 10 to 12 weeks; and 75% to 80% of patients’ remaining survival time was spent in an improved or stable neurologic state. The overall median survival times were 4.1 months in the first study and 3.4 months in the second study, and brain metastases were reported to be the cause of death in 49% or 31% of the patients, respectively. There were no significant differences in symptomatic response rates, duration of response, or survival time according to the treatment regimen: 40 Gy in 15 or 20 fractions, 30 Gy in 10 or 15 fractions, or 20 Gy in 5 fractions.29,48 With the ultrarapid fractionation schemes (10 Gy in one fraction or 12 Gy in 2 fractions; Table 50-2), there was some concern that irradiation may have led to herniation and death within 48 hours of treatment in a small number of cases, and time to neurologic progression was shorter than with more protracted regimens.49,50 These findings were in agreement with conclusions of a study of 15 Gy in 2 fractions over 3 days compared with 30 Gy in 15 fractions.51 Two later RTOG trials failed to show any advantage of 50 Gy in 20 fractions or 54.4 Gy at 1.6 Gy twice daily over 30 Gy in 10 fractions (see Table 50-2),30,31 further solidifying 30 Gy in 10 fractions over 2 weeks as the most frequently used WBRT treatment regimen. Other common treatment regimens include 20 Gy in 5 fractions, 35 to 37.5 Gy at 2.5 Gy per fraction, 40 to 50 Gy at 2.0 Gy per fraction, and 45 to 50.4 Gy at 1.8 Gy per fraction. Shorter regimens may be selected in patients with a shorter life expectancy or when WBRT is delaying the chemotherapy needed to treat systemic disease.
Table 50-2
Selected Randomized Trials of Whole-Brain Radiotherapy Alone for Brain Metastases
Protocol | No. of Years | Fractionation Patients | Median Scheme | Survival Time (mo) |
RTOG 690129,48 | 1971-1973 | 233 | 30 Gy/10 fractions/2 wk | 4.8 |
“First study” | 217 | 30 Gy/15 fractions/3 wk | 4.1 | |
233 | 40 Gy/15 fractions/3 wk | 4.1 | ||
227 | 40 Gy/20 fractions/4 wk | 3.7 | ||
RTOG 736129,48 | 1973-1976 | 447 | 20 Gy/5 fractions/1 wk | 3.4 |
“Second study” | 228 | 30 Gy/10 fractions/2 wk | 3.4 | |
227 | 40 Gy/15 fractions/3 wk | 4.1 | ||
RTOG 690149 | 1971-1973 | 26 | 10 Gy/1 fractions/1 day | 3.4 |
RTOG 736149 | 1973-1976 | 33 | 12 Gy/2 fractions/2 days | 3.0 |
“Ultra-rapid” | ||||
RTOG 760630 | 1976-1979 | 130 | 30 Gy/10 fractions/2 wk | 4.1 |
“Favorable patients” | 125 | 50 Gy/20 fractions/4 wk | 3.9 | |
RTOG 910431 | 1991-1995 | 213 | 30 Gy/10 fractions/2 wk | 4.5 |
“Accelerated hyperfx” | 216 | 54.4 Gy at 1.6/2 days | 4.5 |
Hyperfx, Hyperfractionation; RTOG, Radiation Therapy Oncology Group.
Response and Local Control
Nieder et al.52 studied CT response of brain metastases to WBRT (30 Gy in 10 fractions). By lesion, the complete response rate was 24% and the partial response rate was 35%. The overall (complete plus partial) response rates were 81% for small cell carcinoma, 65% for breast cancer, 56% for squamous cell carcinoma, 50% for nonbreast adenocarcinoma, 46% for renal cell carcinoma, and 0% for melanoma. Complete response rates were 39% for solid metastases, 15% for those with less than 50% necrosis, and 11% for those with at least 50% necrosis, and 52%, 39%, 17%, 20%, 5%, and 0% for lesion volumes ≤0.5 mL, 0.6 to 1.0 mL, 1.1 to 3.0 mL, 3.1 to 6.0 mL, 6.1 to 10.0 mL, and >10 mL, respectively.52 In another study by the same group, there was a suggestion that a higher response rate was obtained with 40 Gy at 2 Gy per fraction with or without a partial brain boost to 50 or 60 Gy compared with 30 Gy at 3 Gy per fraction.53 For the WBRT-only arm of an RTOG trial of WBRT ± SRS, the complete response rate was 8%, the partial response rate was 54%, the stable disease rate was 22%, and the progression rate was 17% among 78 patients with imaging follow-up.54 Data on long-term local control of brain metastases after WBRT alone are limited and highly variable. The 1-year actuarial local control probability by patient ranged from 0% to 14% in the WBRT-only arms of randomized trials reported by Kondziolka et al.55 and Patchell et al.20 but as high as 71% in the WBRT-only arm of the RTOG randomized trial of WBRT ± SRS.54
Partial Brain Radiotherapy
Partial brain radiotherapy can be considered for a single metastasis in lieu of WBRT but is generally not advisable because it would complicate or preclude later WBRT if needed (unlike SRS, which delivers a very focal dose). Caution is advised when postoperative radiotherapy to the posterior fossa alone is contemplated because cerebellar metastases appear to be associated with an increased risk of leptomeningeal dissemination after resection.56,57 On the other hand, partial brain radiotherapy may be useful for treating recurrent brain metastases unsuitable for resection or SRS.
Toxicity of WBRT
Acute toxicity of WBRT includes hair loss, fatigue, and modest skin reactions in essentially all patients and mild acute ototoxicity in some patients. The skin reaction resolves by several weeks, and fatigue improves gradually over 1 or several months. Hair generally regrows by 6 months after WBRT, but alopecia may be permanent in a central strip on the top and back of the head from the reduced skin sparing of tangential radiation beams. In patients who are long-term survivors after WBRT, there is a risk of late hearing loss, retinopathy if the retina was included in the radiation field, and permanent neurocognitive toxicity. DeAngelis et al.56 reported an 11% risk of severe radiation-induced dementia among 1-year survivors after resection of a single brain metastasis and postoperative WBRT to 20 to 40 Gy with high-dose daily fractions. A separate report from the same group described 12 patients cured of brain metastases who experienced severe radiation-induced dementia with associated ataxia and urinary incontinence.58 Imaging with CT showed atrophy, ventricular dilatation, and hypodense white matter. The WBRT schemes included mostly mixtures of 3- to 4-Gy fractions with 5- to 6-Gy fractions, but two of the affected patients had received the standard regimen of 30 Gy in 10 fractions.58 Nieder et al.59 reported a 42% 2-year actuarial probability of symptomatic mild, moderate, or severe late radiation toxicity after resection of a single brain metastasis followed by WBRT at 30 Gy in 10 fractions or 40 Gy in 20 fractions. Among 112 patients on the WBRT-only arm of an RTOG randomized trial, Andrews et al.54 reported one case of grade 3 and one case of grade 4 late CNS toxicity and one case of grade 3 late ototoxicity.
Two prospective, randomized controlled studies assessed neurocognitive function in patients with brain metastases treated with SRS ± WBRT. Aoyama and colleagues60 assessed neurocognitive function through the Mini-Mental State Examination (MMSE). After treatment, 53% of the SRS + WBRT group versus 50% of the SRS-alone group had improvement >3 points. However, the average time until MMSE deterioration was 16.5 months in the WBRT + SRS group versus 7.6 months in the SRS-alone group (P = .05); this finding was attributed to the higher rates of brain tumor recurrence in the latter group. The authors concluded that brain tumor control is the most important factor for stabilizing neurocognitive function but that “the long-term adverse effects of WBRT on neurocognitive function might not be negligible.”60
In 2009, a group from the MD Anderson Cancer Center compared neurocognitive function as their primary end point in a prospective, randomized trial of SRS + WBRT versus SRS alone, using the Hopkins Verbal Learning Test–Revised (HVLT-R) total recall at 4 months.61 The authors found that patients treated with SRS + WBRT were significantly more likely to show a decline in learning and memory function at 4 months, and they recommended initial treatment with SRS alone in conjunction with close follow-up to better preserve learning and memory.
Finally, Sun et al.62 compared neurocognitive function in a group of patients with stage III non–small cell lung cancer (SCLC) who completed definitive therapy without progression and were randomly assigned to prophylactic WBRT or observation. The authors used the MMSE, the Activities of Daily Living Scale, and the HVLT to assess neurocognitive function. At 1 year, there were no significant differences in the MMSE or Activities of Daily Living Scale scores, but the WBRT group had a greater decline in both immediate recall (P = .03) and delayed recall (P = .008) on the HVLT.
Surgery
Surgery has important roles to play in certain subsets of patients, including confirming the diagnosis when needed, relieving mass effect from a large, symptomatic lesion, improving the likelihood of durable local control for a single metastasis, and salvaging a metastasis that failed to respond to prior therapy. Surgical resection may also be useful for lesions with considerable peritumoral edema despite use of steroids or for lesions causing refractory seizures, even if the lesion is sufficiently small that SRS would be a therapeutic option.63 Resection of brain metastases has become safer with advances in neuroimaging and neurosurgery such as image guidance, preoperative and intraoperative functional mapping, and intraoperative ultrasound and MRI.64
Randomized Trials of WBRT With or Without Surgery
Three prospective randomized trials have been performed to evaluate the addition of surgery to WBRT (Table 50-3). Patchell et al.20 reported on 48 patients with a single brain metastasis who were randomly assigned to biopsy and WBRT versus resection and WBRT with 36 Gy in 12 fractions. Patients who underwent resection had significantly improved local control (80% for resection and WBRT vs. 48% for biopsy and WBRT; P < .02), duration of functional independence (median, 38 weeks vs. 8 weeks; P < .005), and survival (median, 40 weeks vs. 15 weeks; P < .01). Factors associated with longer survival included younger age, no extracranial disease, surgical resection, and a longer interval from primary diagnosis to brain metastasis diagnosis.20
Table 50-3
Randomized Trials of Whole-Brain Radiation Therapy ± Surgery or Stereotactic Radiosurgery and Surgery or Stereotactic Radiosurgery ± Whole-Brain Radiation Therapy for Brain Metastases
First Author/Years | Treatment | No. of Patients | Patients With Extracranial Disease (%) | Median Local FFP (mo) | Median Functionally Independent Survival (mo) | Median Survival (mo) |
Patchell20/ 1985-1988 |
Biopsy + WBRT | 23 | 83 | 4.8 | 1.8 | 3.4 |
Surgery + WBRT | 25 | 76 | >13.6 | 8.7 | 9.2 | |
(36 Gy/12 fx) | (P < .0001) | (P < .005) | (P < .01) | |||
Noordijk65/ 1985-1990 |
WBRT | 31 | 68 | — | 3.5 | 6 |
Surgery + WBRT | 32 | 69 | — | 7.5 | 10 | |
(40 Gy at 2 Gy twice a day) | — | (P = .06) | (P = .04) | |||
Mintz66/ 1989-1993 |
WBRT | 43 | 84 | — | — | 6.3 |
Surgery + WBRT | 41 | 73 | — | — | 5.6 | |
(30 Gy/10 fx) | — | (P = NS) | (P = .24) | |||
Patchell70/ 1989-1997 |
Surgery | 46 | 65 | 6.2 | 8.0 | 9.9 |
Surgery + WBRT | 49 | 63 | >12.0 | 8.5 | 11.0 | |
(50.4 Gy/28 fx) | (P < .001) | (P = .61) | (P = .39) | |||
Kondziolka55/ 1985-1988 |
WBRT | 14 | 71 | 6 | — | 7.5 |
WBRT + SRS | 13 | 62 | 36 | — | 11.0 | |
(36 Gy/12 fx) | (P = .0005) | — | (P = .22) | |||
Andrews54/ 1996-2001 |
WBRT | 167 | 69 | [71% at 1 yr] | — | 5.7 |
WBRT + SRS | 164 | 68 | [82% at 1 yr] | — | 6.5 | |
(37.5 Gy/15 fx) | (P = 0.013) | — | (P = .136) | |||
Aoyama103/ 1999-2003 |
SRS | 67 | 43 | [73% at 1 yr] | [27% at 1 yr] | 8.0 |
WBRT + SRS | 65 | 37 | [89% at 1 yr] | [34% at 1 yr] | 7.5 | |
(30 Gy/10 fx) | (P = .002) | (P = .53) | (P = .42) | |||
Chang61/ 2001-2007 |
SRS | 30 | — | [67% at 1 yr] | — | 15.2 |
WBRT + SRS | 28 | — | [100% at 1 yr] | — | 5.7 | |
(30 Gy/12 fx) | — | (P = .012) | — | (P = .003) | ||
Kocher71/ 1996-2007 |
SRS |