Acute Leukemias in Adults
• There were 13,780 new cases of acute myeloid leukemia (AML) and 6,050 new cases of acute lymphocytic leukemia (ALL) in the United States in 2012, resulting in 10,200 deaths from AML and 1440 from ALL. The incidence of AML is relatively low until age 35 and then rises almost exponentially, whereas ALL peaks in incidence in young children. Risk factors for the development of acute leukemia include exposure to benzene, ionizing radiation, or previous chemotherapy.
• The acute leukemias are clonal disorders, with all leukemic cells in a given patients descending from a common precursor. Recent genomewide analyses of leukemia have demonstrated considerable complexity with a number of recurrent potential “driver” mutations and a much larger number of random “passenger” mutations in individual cases.
• The diagnosis of acute leukemia is generally made by bone marrow examination. In the past, the classification of acute leukemia depended heavily on morphologic examination of the leukemic cells. This is no longer the case, and currently the most important elements of classification are the immunophenotype (to distinguish AML from ALL), cytogenetics, and mutational analyses. In AML, three primary-risk groups are recognized. Favorable-risk patients are those with CBF translocations, and those with normal cytogenetics and either NMP1 or CEBPA mutations without mutations in FLT3-ITD. Unfavorable-risk patients are those with abnormalities of 3q, 5 or 7, and those with complex cytogenetics. The intermediate-risk group includes all those not classified as favorable or unfavorable. Acute promyelocytic leukemia, characterized by t(15;17), is a separate entity and requires specific therapy. In ALL, the favorable-risk group includes those with high hyperdiploidy, and del9q. The unfavorable-risk group includes t(4;11), low hypodiploidy/near triploidy, and those with complex cytogenetics. All others comprise the intermediate-risk group. Patients with t(9;22) (Ph+ ALL) and mature B-cell ALL including Burkitt leukemia comprise separate categories of ALL that require specific treatment.
Patients With AML Who Are Candidates for Intensive Therapy
• Induction chemotherapy that includes an anthracycline plus cytarabine will result in a complete remission in approximately 70% of patients. Postremission therapy depends on the risk group. Favorable-risk patients are generally treated with three or four cycles of consolidation chemotherapy, including high-dose cytarabine. With such treatment, approximately 50% of patients will be cured. Patients with intermediate-risk disease should undergo allogeneic transplantation while in first remission if they have a matched sibling or matched unrelated donor. The use of partially matched donors in this setting is less agreed on. Allogeneic transplantation using matched siblings, matched unrelated donors, or partially matched cord blood is recommended for patients with unfavorable-risk disease in first remission.
Patients With AML Who Are Not Candidates for Intensive Therapy
• Low-dose cytarabine is a generally accepted therapy for patients with AML who are not candidates for intensive therapy. Recent alternatives include decitabine, azacitidine, or clofarabine.
• Induction therapy should include all-trans-retinoic acid (ATRA) alone with an anthracycline and, for higher-risk patients, cytarabine. The inclusion of arsenic trioxide during consolidation therapy appears to prolong survival.
Patients With ALL Who Are Candidates for Intensive Therapy
• Induction therapy in ALL includes a combination of vincristine, prednisone, an anthracycline, and asparaginase, with cyclophosphamide sometimes included. With such regimens, 75% to 90% of patients will achieve a complete remission. Postremission therapy generally involves six to eight courses of intensive consolidation therapy, several of which contain high-dose methotrexate, cytarabine, and asparaginase, and several of which include the same drugs used for initial remission induction. Some form of central nervous system (CNS) prophylaxis is required, as is low-dose maintenance therapy. High-risk patients should receive an allogeneic transplant in first remission if at all possible. The role of allogeneic transplantation for patients with standard-risk disease is more controversial.
• A tyrosine kinase inhibitor (imatinib, nilotinib, or dasatinib) should be included as part of induction and consolidation therapy. Allogeneic transplantation is recommended in first remission if an appropriate donor is available.
• Therapy should include, along with the usual drugs used for remission induction, high doses of fractionated cyclophosphamide, high-dose methotrexate and cytarabine, and rituximab.
Epidemiology
According to the American Cancer Society, there will be 13,780 new cases of AML and 6050 cases of ALL in the United States in 2012, resulting in 10,200 deaths from AML and 1440 from ALL.1 The incidence of AML has a bimodal pattern with a modest peak among infants, a decline in childhood, and then an exponential rise with advancing age (Figure 98-1). ALL likewise has a bimodal pattern, but the initial peak occurs among children aged 1 to 4, with a decline between ages 20 and 60, and a more modest rise in advanced years.2 The rates for all forms of acute leukemia are higher in males than females, and tend to be higher in non-Hispanic whites, with the exception of higher rates of acute promyelocytic leukemia (APL) and B-cell ALL among Hispanic whites.2
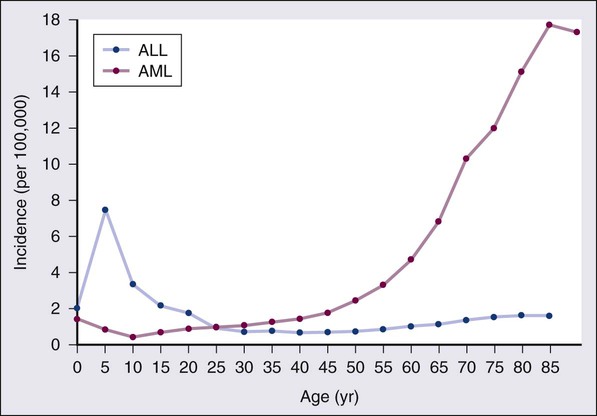
Etiology
Genetic Predisposition
The concordance rate of acute leukemia in identical twins is virtually 100% if one twin develops leukemia during the first year of life, but then declines with age. “Pure familial leukemia” is a term used to describe those rare syndromes caused by a single mutation that leads to leukemia without other manifestations; to date, three have been described involving RUNX1, CEBPA, and GATA2.3,4 The incidence of acute leukemia is also increased in primary bone marrow failure syndromes, such as Diamond-Blackfan, Shwachman-Diamond, and dyskeratosis congenita, as well as in syndromes involving defective DNA repair, such as Fanconi anemia and Bloom syndrome. Acute leukemia is also increased in some of the syndromes associated with loss of tumor suppressor gene function such as Li-Fraumeni syndrome, and in syndromes caused by abnormal chromosomal numbers, including 21+ and XXY.3 Aside from these rare syndromes, there does not appear to be any familial aggregation of acute leukemia; that is, first-degree relatives of patients with acute leukemia are not at increased risk for developing the disease.5
Viruses
Human T cell lymphotropic virus type 1 (HTLV1) is an enveloped, single-strand RNA virus that is associated with the development of a distinct form of adult T cell leukemia found in southwestern Japan, the Caribbean basin, and Africa. The virus can be spread vertically from mother to fetus or horizontally by sexual contact or blood products. Although endemic in the areas noted above, the virus only causes leukemia in 2% to 4% of those infected, and has a very long latency period, estimated at 30 years or more.6 Epstein-Barr virus, a DNA herpes family virus, is associated with the endemic African form of Burkitt lymphoma/leukemia.
Radiation
Ionizing radiation is leukemogenic. An increased incidence of AML, ALL and chronic myelogenous leukemia (CML) was seen in survivors of the atomic bombings of Hiroshima and Nagasaki, which began approximately 1.5 years after exposure, peaked at 7 years, and returned to baseline 25 years later. The risk of leukemia was also increased in individuals treated with radiation for ankylosing spondylitis in the 1940s. Although most of the data from humans showing the leukemogenic potential of ionizing radiation come from individuals exposed to high dose rates, occupational exposure to low dose-rate radiation has more recently been associated with the development of leukemia.7
Prior Therapy
Although the exact percentage is uncertain, as much as 10% of AMLs and a smaller percentage of ALLs are thought to be the consequence of prior exposure to chemotherapy or radiation for a primary malignancy or autoimmune disorder.8 Treatment-related leukemias can be grouped into several syndromes. AML developing after alkylating agent therapy has a latency of 5 to 7 years, often first appears as a myelodysplastic syndrome (MDS), and is frequently associated with abnormalities involving the long arms of chromosomes 5 or 7. All alkylating agents are likely leukemogenic, with an increased incidence of leukemia observed after prolonged exposure, following the use of dose-intense regimens, or when used in certain combinations. Treatment-related AML is also seen following exposure to topoisomerase II inhibitors, such as etoposide, teniposide or anthracyclines. These leukemias develop relatively rapidly, often within 2 years of exposure, are not generally preceded by a myelodysplastic phase, and frequently have rearrangements involving 11q23, the locus for MLL (the mixed-lineage leukemia gene) or 21q22. A number of other drugs have also been implicated in the subsequent development of acute leukemia, including bimolane, an agent used to treat psoriasis, and recently, lenalidomide, an agent used in the treatment of myeloma or myelodysplasia.9 Patients with lymphoma who receive autologous hematopoietic cell transplants are at increased risk for the development of leukemia, which may, in part, be predicted by the therapy received before the transplant.
Not all treatment-related leukemias fall into the discrete categories described here. Secondary leukemias with inv(16), t(9;22), and abnormalities involving 3q21 have been reported. Although there remains some debate, in general, patients with treatment-related leukemias do not fare as well as patients with de novo leukemia, even after adjusting for known risk factors.10
Pathobiology
Clonality and Clonal Architecture
Recent genome-wide analysis of leukemia has demonstrated considerable complexity with a number of recurrent potential “driver” mutations, perhaps up to 10, and a much larger number of random “passenger” mutations in each individual case. Thus overt leukemia is not the result of a single mutation. Careful studies in both AML and ALL have further found considerable variability in the mutations among the individual leukemic cells in any single case. The pattern of variability suggests that the development of leukemia is not a simple progressive accumulation of mutations in an essentially linear direction but rather is a dynamic process shaped by multiple cycles of mutation acquisition and clonal selection.11,12
The Leukemic Stem Cell
Within the clonal populations of cells in patients with acute leukemia, there must be a subpopulation of cells capable both of self-renewal and proliferation. Such cells have been termed “leukemia stem cells.” Although there is no gold standard for their identification, the ability of human leukemic cells to engraft long-term in immunodeficient NOD/SCID mice is generally accepted as a marker for a leukemia stem cell. In human AML, the subpopulation of cells capable of long-term engraftment in immunodeficient mice is within the CD34++CD38– CD 123+ fraction and comprises only a small percent (0.2 to 100/106) of the leukemic cells present.13 However, many cases of AML do not engraft even in the more permissive NOD/SCID/IL2R immunodeficient mouse, including almost all cases of acute promyelocytic leukemia (APL), and thus the ability of leukemic cells to grow in an immunodeficient mouse is not a true assay for the leukemia stem cell.
Morphology
AML cells are typically 12 to 20 µm in diameter with large nuclei, multiple nucleoli, and azurophilic granules in the cytoplasm. ALL cells are typically smaller than AML blasts and are devoid of granules (Figure 98-2). For more than 3 decades, the French-American-British (FAB) system was used to describe and classify morphologic subtypes of AML and ALL. Morphologic subclassification of acute leukemia adds little prognostic or therapeutic information beyond that provided by cytogenetic and mutational categorization, but still remains part of the overall World Health Organization (WHO) classification schema.
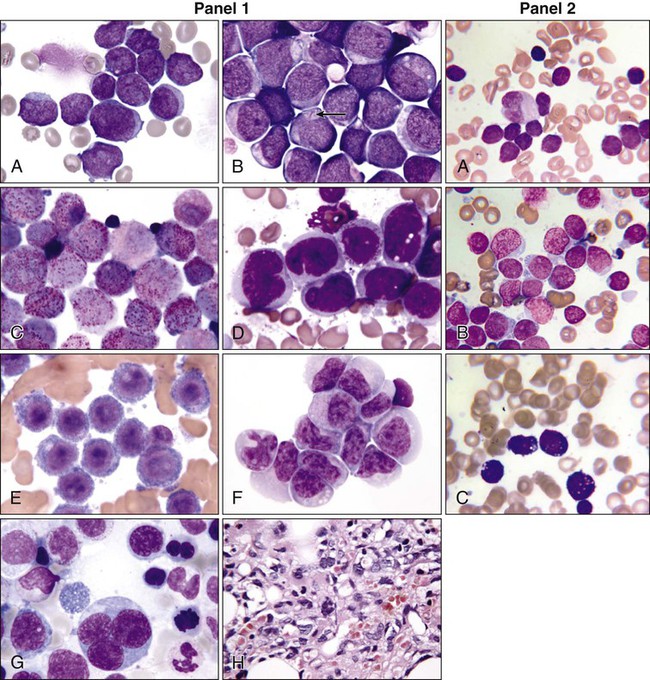
Immunophenotyping
Acute leukemias can be categorized according to the cell surface antigens they express as determined by their reactivity to a panel of monoclonal antibodies (Table 98-1). The cell surface antigens expressed largely reflect the level of differentiation of the leukemic cell, and this information provides some prognostic information, especially in ALL. In addition, the antigens on leukemia blasts are almost always present in abnormal combinations or concentrations, allowing for the detection of as few as 0.01% abnormal cells in an otherwise normal looking bone marrow using multidimensional flow cytometry.
Table 98-1
Immunophenotype of Acute Leukemia
AML | |
Precursor stage | CD34, CD117, HLA-DR |
Granulocyte marker | CD13, CD 33, cMPO |
Monocytic marker | CD14, CD15, CD11b, NSE |
Megakaryocytic marker | CD41a, CD61 |
Erythroid marker | CD36, CD71 |
ALL | |
Pro-B | C19 or CD22+ CD10– |
Common ALL | CD10+ |
Pre-B | Intracytoplasmic Ig+ |
Mature B | Surface immunoglobulin |
Early T | CD7+ CD1a–, surface CD3– |
Cortical T | CD1a+, surface CD3– |
Mature T | Surface CD3+ |
A small percentage of cases of acute leukemia express characteristics of both lymphoid and myeloid cells, and have recently been defined by the WHO as mixed-phenotype acute leukemia (MPAL).14 To fulfill the WHO criteria, the leukemia must express the myeloid-specific marker myeloperoxidase (either by flow cytometry or cytochemistry), and either a strictly specific T lymphoid marker (cytoplasmic CD3) or a B lymphoid marker (strong expression of CD19 or weak expression of CD19 together with expression of at least three other B-cell-associated markers). Many of these patients will have rearrangements of BCR/ABL or MLL or have complex cytogenetics. The median survival for this rare group of patients appears to be short, in the range of 18 months, and they should be considered as a high-risk group when selecting appropriate therapy.
Cytogenetics
For AML, the Southwest Oncology Group (SWOG) and the Medical Research Council (MRC) each developed and adopted cytogenetic risk classifications over two decades ago based on the outcomes of large prospective clinical trials.15,16 The resulting classifications, though similar, are not identical. For example, as shown in Table 98-2, in the SWOG categorization, patients with clonal abnormalities not recognized as within one of the three major groups are assigned to a fourth category with a predicted treatment outcome somewhere between the intermediate- and unfavorable-risk groups. And there are minor differences between classification schemas in the distinction between intermediate and unfavorable risk cytogenetics. More recently, in 2010, the MRC revised their classification system, with the major change being the movement of patients with various translocations involving chromosome 11 (with the exception of t(9;11) and t(11;19)) from the intermediate-risk group into the unfavorable group.17 Although these classification schemas differ slightly, they are each able to distinguish three major groups of patients. Among patients age <65 treated with standard chemotherapy, those with a favorable karyotype have complete response (CR) rates in the 85% to 90% range and a 5-year overall survival (OS) of 50% to 60%. Those with intermediate-risk cytogenetics have CR rates of 65% to 75%, and a 5-yr OS of 35% to 45%. And for those with unfavorable-risk cytogenetics, a CR rate of 45% to 55% and a 5-yr OS of 10% to 20% can be expected.
Table 98-2
Cytogenetic Classification of AML
Risk Status | SWOG15 | MRC (1998)16 | MRC (2010)17 |
Favorable | t(15;17), t(8;21), inv(16)/t(16;16)/del(16q) | t(15;17), t(8;21), inv(16)/t(16;16)/del(16q) | t(15;17)(q22;q21), t(8;21)(q22;q22), inv(16)(p13q22)/t(16;16)(p13;q22) |
Intermediate | Normal, +8, +6, -Y, del(12p) | Normal, 11q23 abn, +8, del(9q), del(7q), +21, +22, all others | Abnormalities not classified as favorable or unfavorable |
Unfavorable | abn(3q), del(5q)/-5, -7/del(7q), t(6;9), t(9;22), 9q, 11q, 20q, 21q, 17p, complex (≥ 3 unrelated abnormalities) | abn(3q), del(5q)/-5, -7, complex (≥ 5 unrelated abnormalities) | abn(3q) [excluding t(3;5)(q21~25;q31~35)], inv(3)(q21q26)/t(3;3)(q21;q26), add(5q)/ del(5q)/-5, add(7q)/del(7q)/-7, t(6;11) (q27;q23), t(10;11)(p11~13;q23), t(11q23) [excluding t(9;11)(p21~22;q23) and t(11;19) (q23;p13)],t(9;22)(q34;q11), -17/ abn(17p),complex (≥ 4 unrelated abnormalities) |
Unknown | All other abnormalities | Category not recognized | Category not recognized |
The finding of a single monosomy is a relatively common finding in AML, with monosomy 7 being the most frequent. The presence of a single monosomy (excluding loss of a sex chromosome) is associated with a negative outcome, with a 12% OS at 4 years in the Dutch experience.18 Patients with two or more distinct monosomies, or a single monosomy but with an additional structural abnormality, have an even worse prognosis, with a predicted 4-year survival of less than 4%.18 Thus this category of patient (two monosomies or one plus an additional structural abnormality) has been defined as having a “monosomal karyotype” and identifies a group of patients with a very dismal prognosis if treated with conventional chemotherapy. There is obvious overlap among patients with unfavorable, complex, and monosomal karyotypes. All patients with complex karyotypes fall within the unfavorable-risk group and comprise between a third and half of the total, and almost all patients with a monosomal karyotype are within the complex category, accounting for about two-thirds of those patients.
As in AML, cytogenetic analysis plays an important role in the evaluation of patients with ALL (Table 98-3). The most common abnormality in ALL, seen in 20% to 30% of cases, is t(9;22)(q34;q11), which results in the Philadelphia chromosome. Other common translocations in adult B-cell ALL include t(4;11)(q21;q23), which is seen in 7% of cases, involves the MLL and AF4 gene and is associated with a poor prognosis, and t(8;14)(q24.1;q32), which is seen in 2% to 4% of adult B-cell ALL, involves c-MYC and the immunoglobulin heavy chain, and is the translocation associated with Burkitt lymphoma. T-cell ALLs often have translocations involving chromosomes 7 or 14 at T-cell receptor enhancer gene sites. The other most common cytogenetic changes seen in adult ALL involve del(9p) seen in 5% to 9% of cases, del(6q) seen in 5% to 7% and del(13q) seen in 3% to 5%. Abnormalities in chromosome number are also common. High hyperdiploidy (51 to 65 chromosomes) is seen in as many as 10% of cases, whereas low hypodiploidy (30 to 39 chromosomes)/near triploidy (60 to 78 chromosomes) is seen in 4%. Approximately 5% will have a complex karyotype. Compared with those cases of ALL with normal cytogenetics, those with del(9q) or high hyperdiploidy appear to have a more favorable outcome, whereas those with t(4;11), t(8;14), and del(6q), low hypodiploidy/near triploidy, or complex cytogenetics do worse (Figure 98-3).19
Table 98-3
Major Cytogenetic Categories in Adult ALL
Incidence (%) | |
FAVORABLE | |
High hyperdiploidy | 10 |
del 9p | 9 |
UNFAVORABLE | |
t(9;22) | 19 |
t(4;11) | 7 |
t(8;14) | 2 |
Low hypodiploidy/near triploidy | 4 |
Complex | 5 |
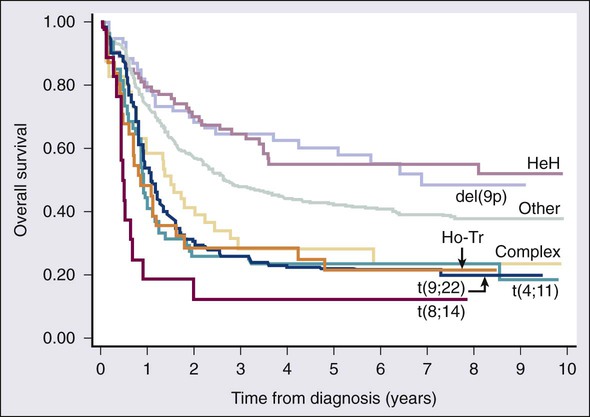
Mutational Events
AML-Associated Mutations
Retinoic Acid Receptor Alpha Translocations
Acute promyelocytic leukemia (APL), which accounts for approximately 8% of adult AML cases, is almost always associated with t(15;17)(q22;q11.2), a translocation that fuses the promyelocytic leukemia gene (PML) on chromosome 15 to the retinoic acid receptor alpha (RARa) gene on chromosome 17. The resultant PML/RARa fusion protein acts as a dominant negative inhibitor of normal PML function and of the function of RXRa, an important heterodimeric partner of RARa. PML/RARa recruits a nuclear co-repressor (NCoR) and the molecules sin3 and histone deacetylase (HD) (Figure 98-4). HD deacetylases histones, a process that in turn inhibits binding of transcription factors, thus inhibiting the expression of genes required for hematopoietic differentiation. The unique activity of all-trans-retinoic acid (ATRA) in APL appears to be explained by its ability to bind to the PML/RARa fusion, changing its configuration and releasing the attached nuclear co-repressor. This then allows subsequent transcription and gene expression with subsequent maturation of the APL blasts. A number of other translocations, including t(5;17) and t(11;17), involve RARa and result in an APL-like phenotype. These leukemias are, however, generally unresponsive to ATRA because clinically achievable concentrations of the drug do not result in release of the NCoR-HD complex.
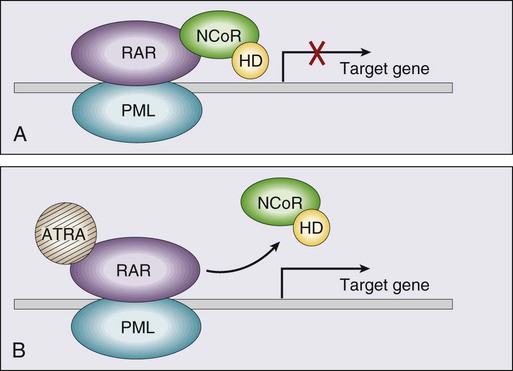
Core Binding Factor Translocations
Core binding factor is a heterodimeric transcription factor made up of two subunits, CBFα (also known as RUNX-1) and CBFβ. CBF plays a role in the transcriptional activation of a number of genes required for normal hematopoietic differentiation. The t(8;21) abnormality seen in approximately 8% of adult AML cases results in the fusion of the RUNX1 gene on chromosome 21 to the MTG8 gene on chromosome 8. The RUNX1/MTG8 fusion protein acts as a dominant negative inhibitor of the wild-type RUNX-1 gene (Figure 98-5). AML characterized by t(8;21) has a favorable prognosis. Similarly, inv(16)(p13;q22) and t(16;16)(p13;q22) both result in the fusion of CBFβ at 16q22 to the smooth-muscle myosin heavy chain (MYH11) gene at 16p13. As in the case of t(8;21), the resultant fusion protein acts as a dominant negative regulator of transcription. AML cases with involvement of 16q22 account for approximately 9% of adult AML cases, have a unique myelomonocytic morphology, and like t(8;21) leukemias have a favorable prognosis.
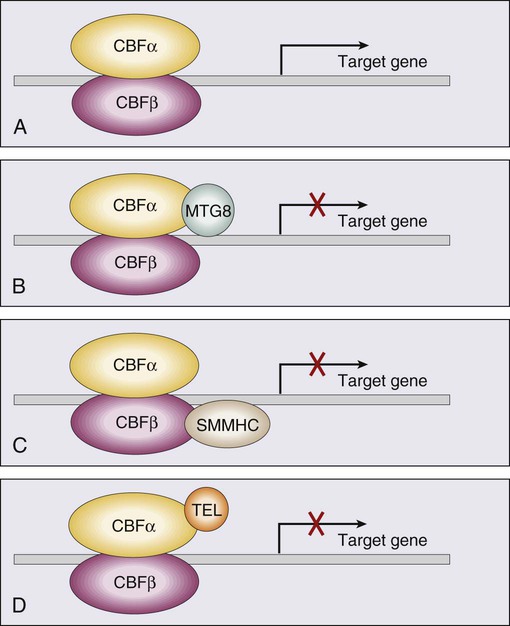
ALL-associated Mutations
Nonrandom Mutations in B-cell ALL
Cytogenetic studies of B-cell ALL reveal that a portion of cases are associated with the translocation of the MYC gene on chromosome 8 and immunoglobulin enhancer response genes on chromosomes 14 or 22. High-resolution single nucleotide polymorphism array analysis of B-cell ALL has found that in more than 40% of B-cell ALLs, deletion, amplication, point mutation, or structural rearrangements of gene encoding regulators of B cell development can be found.20 The two most frequently involved genes are PAX5, which is altered in about 30% of cases, and IKZF1 (IKAROS5), altered in approximately 25% of cases. Only a limited number of studies have been conducted analyzing the association of alterations in these genes with outcome. Available data suggest that mutations in IKZF1 may be linked with a poor prognosis.
Nonrandom Mutations in T-cell ALL
Cytogenetic studies of T-cell ALL show that the disease is often characterized by translocations involving chromosomes 7 or 14 at the sites of T-cell receptor enhancer genes on those chromosomes. More recent studies have found recurrent mutations in NOTCH1 or FBXW7 in sizable subsets of T-cell ALL patients.21 NOTCH1 is located on chromosome 9q34 and plays a key role in cell fate choice. NOTCH1 appears to be mutated in anywhere from 34% to 71% of T-ALL cases; the impact of NOTCH1 mutations on treatment outcome is not yet clear. FBXW7, located at 4q31.3, codes for a component of the ubiquitin ligase complex, and is mutated in 10% to 15% of T-cell ALL cases. The prognostic impact of mutations in FBXW7 is not yet clear. A variety of other genes including FLT3, BCL11B, and PTPN2 have been found to be mutated in occasional cases of T-cell ALL, but no prognostic importance has been identified with their presence.22
Gene and MicroRNA Expression
The mutational events that underlie acute leukemia result in aberrant gene and microRNA expression.23 Abnormal expression of a number of single genes, including BAALC, MN1, ERG, and EVI1, is commonly seen in AML and has been tied to prognosis. Distinct clusters of AML and ALL based on genomewide expression signatures have been described. These clusters tend to be associated with specific cytogenetic or molecular alterations. MicroRNAs are noncoding RNAs that hybridize to imprecisely complementary mRNA of protein-coding genes, leading to their downregulation. Aberrant expression of multiple microRNAs has recently been reported in acute leukemia. Studies of alternations in gene and microRNA expression have contributed to our understanding of normal and altered hematopoiesis, but they have not yet advanced to the point of affecting clinical practice.
Classification of Acute Leukemia
The World Health Organization classification of acute leukemia is based on clinical, morphologic, immunophenotypic, cytogenetic, and molecular features (Table 98-4).24 Though comprehensive, this classification system is not organized according to prognosis and is not widely used in the development of treatment guidelines.
Table 98-4
World Health Organization Classification of Acute Leukemia with Corresponding FAB Classification Subtypes (2008)
B lymphoblastic leukemia/lymphoma, NOS
B lymphoblastic leukemia/lymphoma with recurrent genetic abnormalities
B lymphoblastic leukemia/lymphoma with t(9;22)(q34;q11.2);BCR-ABL 1
B lymphoblastic leukemia/lymphoma with t(v;11q23);MLL rearranged
B lymphoblastic leukemia/lymphoma with t(12;21)(p13;q22) TEL-AML1 (ETV6-RUNX1)
B lymphoblastic leukemia/lymphoma with hyperdiploidy
B lymphoblastic leukemia/lymphoma with hypodiploidy
B lymphoblastic leukemia/lymphoma with t(5;14)(q31;q32) IL3-IGH
B lymphoblastic leukemia/lymphoma with t(1;19)(q23;p13.3);TCF3-PBX1
Among the most broadly used classifications schemas for AML is one developed by the European Leukemia Net (ELN) (Table 98-5).25 This classification system combines the already well-established cytogenetic risk categories illustrated in Table 98-2 with newer mutational analyses. Briefly, the favorable category continues to include the core binding factor leukemias, t(8;21) and inv(16), but adds those with a normal karyotype and with either mutated CEBPA or with mutated NPM1 without FLT3/ITD. The intermediate category is split into intermediate-I and intermediate-II. The intermediate-I category includes all those with a normal karyotype except those in the favorable subgroup, whereas intermediate-II includes all of those with cytogenetic abnormalities not defined as favorable or unfavorable. The unfavorable group remains essentially the same as in the traditional cytogenetic schemas. A recent retrospective study analyzed survival in 1550 AML patients according to the suggested ELN definitions.26 Figure 98-6, A and B, present the survival of newly diagnosed AML patients younger than (A) or older than (B) age 60 according to ELN categorization. In both age groups, those in the favorable group have the best overall survival; those with intermediate-II disease appear to do somewhat better than those with intermediate-I disease; whereas those in the unfavorable category have a very poor outcome. More complex systems attempting to integrate additional mutations or mutations and gene expression markers have been published, but none of these systems has gained wide usage as yet.27,28
Table 98-5
ELN Risk Stratification for Adult AML
Genetic Group | Subsets |
Favorable | t(8;21)(q22;q22); RUNX1-RUNX1T1 |
inv(16)(p13.1q22) or t(16;16)(p13.1;q22); CBFB-MYH11 | |
Mutated NPM1 without FLT3-ITD (normal karyotype) | |
Mutated CEBPA (normal karyotype) | |
Intermediate-I | Mutated NPM1 and FLT3-ITD (normal karyotype) |
Wild-type NPM1 and FLT3-ITD (normal karyotype) | |
Wild-type NPM1 without FLT3-ITD (normal karyotype) | |
Intermediate-II | t(9;11)(p22;q23); MLLT3-MLL |
Cytogenetic abnormalities not classified as favorable or adverse | |
Adverse | inv(3)(q21q26.2) or t(3;3)(q21;q26.2); RPN1-EVI1 |
t(6;9)(p23;q34); DEK-NUP214 | |
t(v;11)(v;q23); MLL rearranged | |
−5 or del(5q); −7; abnl(17p); complex karyotype |
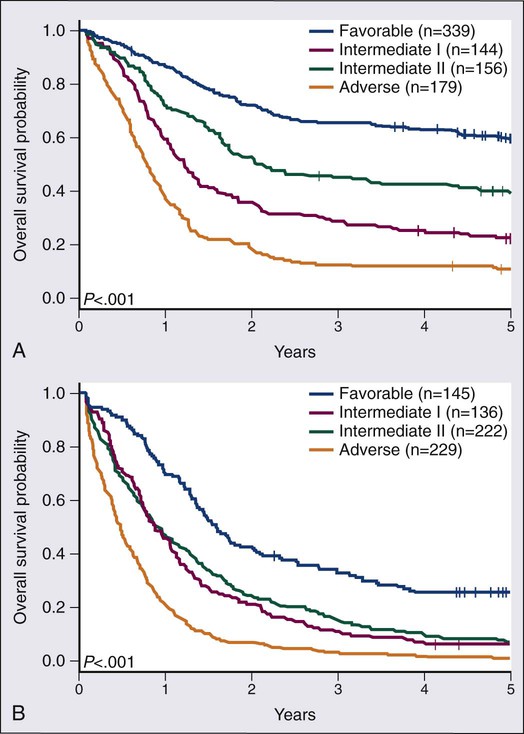
The WHO classification schema for ALL defines subcategories of the disease according to immunophenotype and specific translocations, and as for AML, does not create categories of disease according to prognostic risk. A large number of clinical groups have developed their own definitions of risk categorization. These definitions vary, but in general, most have settled on three risk categories: standard risk with an estimated 5-year survival of around 50%, intermediate risk with an estimated survival of approximately 30%, and high risk with an estimated survival of less than 20% (Table 98-6).29 These schemas typically define a number of risk factors, and patients with no risk factors make up the standard risk group, those with one or two factors make up the intermediate-risk group and those with three or more comprise the high-risk group. Risk factors vary between studies, but typically include age >35, high WBC at diagnosis (>30,000/mm3 for B-cell ALL and >100,000/mm3 for T-cell ALL), cytogenetics with t(4;11), t(9;22), MLL rearrangements, or low hypodiploidy/near triploidy, or CD10-negative pro-B phenotype, and in some studies, slow initial response with greater than 4 weeks required to obtain first remission.
Clinical Manifestations
AML tends to develop more gradually, often over weeks to months. Chloromas, which are local collections of blasts, can present as rubbery, fast-growing, soft tissue masses. Gingival hyperplasia due to leukemic infiltration of the gums is sometimes seen, particularly with M5 AML (Figure 98-7). AML sometimes infiltrates the skin and results in a raised, nonpruritic rash termed leukemia cutis (Figure 98-8). Although uncommon, an occasional AML patient (1% to 2%) may present with meningeal signs or cranial neuropathies (most often affecting cranial nerves IV or VII) because of infiltration of the central nervous system (CNS) with leukemia, and another 2% will have unsuspected involvement if a diagnostic lumbar puncture is performed.
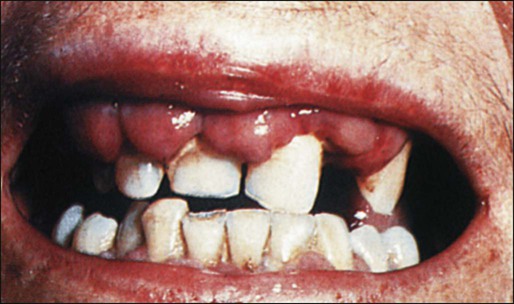
Therapy
Preparing the Patient for Treatment
Acute Myeloid Leukemia
Many treatment guidelines for AML provide separate suggestions for patients younger or older than an arbitrary age cutoff, usually around 60 years, based on the implicit assumption that younger patients can tolerate more intensive therapy than their older counterparts. This assumption was recently questioned by examining the outcome of therapy in 3365 patients with AML; a multicomponent model that accurately predicted treatment related mortality was created and included age, performance status, platelet count, and whether patients had primary versus secondary disease among other factors.30 Although age was a component of the model, eliminating age from the model only minimally affected the model’s accuracy. Thus recommendations for initial induction therapy should be based on whether patients are eligible for intensive therapy or not, and not on age per se. In general, intensive therapy is appropriate for patients at least up to age 70 if they have good performance status and limited comorbidities.
Remission Induction
Standard induction therapy includes a combination of an anthracycline and cytarabine. Prospective randomized trials have shown that higher doses of daunorubicin (60 to 90 mg/m2/d for 3 days) or idarubicin (12 mg/m2/d for 3 days) are superior to a lower dose of daunorubicin (45 mg/m2/d for 3 days).31;32 There does not appear to be any advantage to doses of cytarabine higher than 200 mg/m2/d by continuous infusion for 7 days.33 A bone marrow examination should be performed approximately 7 days after completing initial chemotherapy, and if residual leukemia is present, the induction cycle should be repeated. With this approach, 65% to 80% of patients will achieve an initial remission. Approximately 5% of patients die of bleeding or infection during the period of profound myelosuppression that follows induction therapy, and approximately 20% will have persistent leukemia despite intensive induction therapy. Attempts to improve the outcome of induction therapy by adding other agents have not convincingly improved outcomes, although there are some recent encouraging results with the use of gemtuzumab ozogamicin.
Postremission Chemotherapy
If no further therapy is given after patients enter remission, all will inevitably relapse and will do so rapidly, on average in about 4 months, and thus further therapy is needed. Three types of therapy are available, chemotherapy, allogeneic hematopoietic cell transplantation (allogeneic HCT), or autologous HCT. The best form of chemotherapy is unsettled. One trial showed that four cycles of high-dose cytarabine administered at 3 g/m2 every 12 hours on days 1, 3, and 5 yielded superior outcome compared with less-intensive cytarabine therapy given at either 100 mg/m2 or 400 mg/m2 by continuous infusion for 5 days.34 The survival benefit with the high-dose arm was largely restricted to patients with AML with favorable-risk cytogenetics. More recently, consolidation with intermediate-dose cytarabine (1 g/m2 every 12 hours days 1 to 6) was compared with high-dose cytarabine (3 g/m2 every 12 hours days 1 to 6); no advantage was seen with the higher-dose regimen.35
Allogeneic Hematopoietic Cell Transplantation
Multiple prospective studies have been conducted in which younger AML patients, generally those under age 55, who have achieved a complete remission are assigned to allogeneic HCT if they have a matched sibling or to aggressive chemotherapy or autologous HCT if they lack a donor. Several meta-analyses have been conducted of such studies and show that survival is improved with allogeneic transplant in first remission.36 When examined by cytogenetic risk category, the survival advantage is lost for patients with favorable-risk disease and is most marked for patients with unfavorable-risk disease.
Based on these analyses, currently patients with favorable-risk AML are generally treated with multiple cycles of consolidation chemotherapy with allogeneic HCT employed only if patients relapse. As noted earlier, patients with intermediate-risk cytogenetics can be further categorized according to mutational analyses. Those with normal cytogenetics but mutations in either NPM1 or CEBPA without FLT3/ITD constitute a more favorable group, whereas the remainder makes up a less favorable group. A prospective analysis was conducted in 872 patients with cytogenetically normal (CN)-AML in first remission.37 Among the 135 patients with mutant NPM1 without FLT3/ITD, there was no benefit for having a donor and presumably proceeding to allogeneic HCT (which 80% of patients did), as compared with the no-donor group (hazard ratio for relapse or death during CR, 0.92; 95% CI, 0.47 to 1.81). Conversely, there was a clear benefit for having a donor and proceeding to allogeneic HCT in the remaining patients with CN-AML (hazard ratio, 0.61; 95% CI, 0.40 to 0.94).
The benefit of allogeneic HCT is most marked for patients with unfavorable-risk cytogenetics. However, only about a third of all patients will have a matched sibling to serve as a donor. With the development of unrelated donor registries, it is now possible to find a matched unrelated donor (URD) for many patients. Data from single centers, and more recently from transplant registries, show that the outcomes of transplants from matched siblings and matched unrelated donors are very similar.38 For example, in a study from the Center for International Blood and Marrow Transplant Research (CIBMTR), transplantation for high-risk AML in 226 recipients of matched related HCT was compared to that in 358 recipients of matched URD HCT.38 OS at 3 years was 45% with matched related donors versus 37% for matched URDs. In multivariate analysis, these outcomes were indistinguishable (RR = 1.06). Although matched unrelated donors can be found for many patients, for others no such donors are available. This is a particular problem for blacks, Hispanics, and patients of mixed racial background. In the study from the CIBMTR, outcomes were significantly worse with the use of partially matched unrelated donors. Recently, the results of transplantation from matched related donors, matched unrelated donors, and partially matched double cord blood transplants were compared in a study from Seattle and Minnesota.39 Although transplant-related mortality was higher with double cord transplants, relapse rates were less, leading to equivalent overall survival using matched siblings, matched unrelated donors, and double cord transplants.
The CIBMTR and Seattle/Minnesota study suggest that if a matched related donor is not available for a younger patient with unfavorable-risk AML, consideration should be given to proceeding to either a matched unrelated or cord blood transplant. However, these were retrospective studies and considerable patient selection bias could have influenced results. Recently, the results of AMLHD98A from the German-Austrian group were published.40 In this study, 844 patients were enrolled and 267 were determined to be high risk either by virtue of having high-risk cytogenetics and achieving a CR (n = 51), or not achieving a CR with initial induction therapy (n = 216). Attempts were made to transplant all high-risk patients and, in fact, 61% were actually transplanted. Among high-risk patients, the 5-year OS rates were 6.5% in those not transplanted, versus 25.1% in those receiving a transplant. There was no difference in outcome using matched related and matched unrelated donors.
The role of transplantation for fit, but older, patients is less clear. There have been essentially no sufficiently sized prospective trials yet reported where older patients have been followed from diagnosis and the outcomes of transplantation versus chemotherapy compared. The largest retrospective trial comes from the Japanese National Cancer Center who examined the outcome of 1036 patients aged 50 to 70 with AML who achieved a first CR and were treated with either allogeneic HCT (n = 152) or chemotherapy (n = 884).41 Both overall survival and relapse-free survival were significantly improved in the HCT group, but patient selection bias could have a major potential impact on the study results.
Autologous Hematopoietic Cell Transplantation
In the decade between 1989 and 1998, six prospective randomized trials were performed in which patients with AML in first remission were randomized to receive either autologous HCT with bone marrow as the source of stem cells or further chemotherapy. A meta-analysis of these trials showed that autologous HCT was associated with better disease-free survival but similar overall survival. In the decade since the completion of these trials, there have been at least three similar studies conducted using peripheral blood rather than marrow as the stem cell source. The general conclusions of these more recent studies were similar to those using marrow, and a meta-analysis of all trials comparing autologous HCT to chemotherapy published through 2010 concluded that autologous HCT is associated with improved disease-free survival but no benefit in overall survival. Based on these outcomes, an evidence-based review of the field conducted by the American Society for Blood and Marrow Transplantation concluded that for patients with AML first remission “there is no significant advantage for autologous HCT over chemotherapy.”42
Treatment of Recurrent AML
Occasionally, second remissions can last for periods beyond 3 years; such long second remissions are restricted to the rare patients with favorable risk cytogenetics who had a long first remission. Generally, however, second remissions are short, with an average duration of 6 months. A prognostic index for overall survival of patients with AML in first relapse identified the duration of first remission, age, and cytogenetics as the three most important predictors, with the best overall survival seen in younger patients with a longer first remission and favorable risk cytogenetics.43 No matter the risk category, improved survival was seen in those patients who were able to receive an allogeneic HCT.
Treatment of AML in Patients Not Candidates for Intensive Therapy
If the choice is made to not pursue aggressive treatment, the proven therapeutic options for patients with AML are limited. Palliative care alone may be an appropriate choice for patients with substantial comorbidities. In a randomized trial, low-dose cytarabine was found to be superior to hydroxyurea. Single-agent clofarabine has been studied in a cohort of 112 patients older than age 60 and resulted in an encouraging complete response rate of 42% in patients with unfavorable-risk cytogenetics. Azacitidine and decitabine have both been found to have activity in this population of patients. In an interesting recently published trial, Fenaux et al. asked physicians to pick one of three approaches as their “standard therapy,” either supportive care alone, low-dose cytarabine, or standard daunorubicin plus cytarabine, and then randomized 113 patients to either the selected standard care or azacitidine.44 Median OS for the azacitidine group was 24.5 months compared with 16 months in the conventional treatment group. In the small subset of patients with unfavorable-risk cytogenetics (27 total), those treated with azacitidine had a median survival of 12 months, compared to 5 months when treated with conventional care. The longer survival did not depend on achieving a CR.
Treatment of Acute Promyelocytic Leukemia
APL is a distinct subtype of AML, accounting for 5% to 15% of cases (average, approximately 10%), with unique clinical, morphologic, and cytogenetic features.45 Compared with most patients with AML, patients with APL tend to be somewhat younger (median age, 30 to 40 years), rarely have a myelodysplastic prodrome, and usually have a lower white blood cell count at the time of diagnosis. Compared to other subtypes of AML, APL appears to be overrepresented among Hispanics. Although many of the clinical and laboratory features are similar to other forms of AML, APL almost always presents with some elements of a hemorrhagic syndrome, including hypofibrinogenemia, decreased normal coagulation factors, elevated fibrin degradation products, and increased platelet consumption. These findings are the result of both DIC and primary fibrinolysis. As noted earlier, leukemic blasts virtually always have the characteristic translocation t(15;17)(q22;q11.12).
Unique features of APL are its sensitivities to treatment with ATRA and arsenic trioxide. As a single agent, ATRA results in complete response rates of 80% or more for patients with recurrent disease who have not previously been exposed to the drug. Similarly, arsenic trioxide, when used as a single agent, results in complete remission for 85% of patients with arsenic-naïve recurrent disease. The robust activity of these agents led to studies combining them with conventional chemotherapy as initial therapy for APL. Randomized trials have demonstrated that the addition of ATRA to conventional chemotherapy improves complete response rates to approximately 90% and decreases the incidence of bleeding complications. These trials also demonstrated a clear role for ATRA as maintenance therapy. More recently, a large randomized trial demonstrated that the use of arsenic trioxide during consolidation therapy further improves disease-free and overall survival.46 With current therapies, survival at 3 years from diagnosis can be expected for more than 85% of patients presenting with a WBC count of less than 10,000/mm3 and for 75% of those presenting with a WBC count of more than 10,000/mm3. A small fraction of patients with APL morphology will have a different translocation, for example, t(11;17), and respond poorly to ATRA and arsenic.
Measuring Minimal Residual Disease in AML
Measurement of minimal residual disease (MRD) can theoretically be used in AML to direct therapy after induction and to monitor response. There are two general approaches to measurement of MRD in AML, PCR-based methods and multidimensional flow cytometry (MFC).47 PCR-based techniques can be used in three settings. Approximately 30% of cases of AML are associated with a fusion gene, such as t(8;21), inv(16), or MLL translocations that can be assayed by PCR. In an additional 30% to 40% of cases, gene mutations in fusion-negative AML cases exist and can be monitored by PCR. Examples here include FLT3-ITD, NPM1, and CEBPA. Overexpression of a gene such as WT1 might also serve as a marker for a PCR-based assay of MRD. The advantages of PCR-based assays are their remarkable sensitivity and the relative ease of their performance. Their shortcomings include the fact that such assays are only available for perhaps 70% of the AML population; there are as yet no generally agreed-upon standardized assays and cutoffs, and for some mutations, there is a question about their longitudinal stability.
Acute Lymphocytic Leukemia
Remission Induction
Similar to AML, the initial goal of therapy in ALL is to eliminate the vast majority of the leukemic cells allowing recovery of normal hematopoiesis. Most contemporary induction regimens include combinations of vincristine, prednisone, an anthracycline, and asparaginase, with cyclophosphamide sometimes included. With such regimens, complete response rates of 75% to 90% have been achieved.29,48 No one regimen has been convincingly demonstrated to be superior. However, the addition of cyclophosphamide and more intensive dosing of asparaginase are generally accepted as being beneficial, particularly for patients with T-cell ALL. As will be discussed, patients with Ph+ ALL and those with mature B-cell phenotype (Burkitt leukemia) require specific therapies. Patients who present with CNS leukemia are often treated with triple intrathecal therapy (methotrexate, cytarabine, and prednisone) biweekly until clearing of the CSF, followed by cranial irradiation.
Hematopoietic Cell Transplantation
The role of allogeneic HCT for adult patients with ALL is unsettled. There is general agreement that allogeneic HCT from a matched sibling is appropriate for patients with high-risk ALL, including those with Ph+ ALL, t(4;11) ALL, adverse immunophenotype or cytogenetics, CNS involvement, and higher leukocyte counts at diagnosis. Depending on the actual high-risk feature, cure rates of 15% to 25% are seen with standard chemotherapy, but are increased to 35% to 60% with transplantation. There is also general acknowledgment that outcomes using matched unrelated and cord blood donors approach those with matched siblings. There is less agreement about the role of allogeneic HCT for patients with standard-risk disease. A recent evidence-based review by the American Society of Blood and Marrow Transplantation led to the conclusion that new data indicates that myeloablative allogeneic HCT is an appropriate treatment for adult ALL in first remission for all disease groups. Allogeneic HCT provides a significant improvement in overall and leukemia-free survival in younger (<35 years), standard risk Ph- patients compared with less intensive chemotherapy regimens. In older (>35 years), standard risk Ph- ALL patients, a higher transplant-related mortality diminishes the significant survival advantage with allogeneic HCT.49
Measuring Minimal Residual Disease in ALL
As in AML, there are two general approaches to measuring MRD in ALL, PCR-based approaches and those based on MFC.50 One PCR-based approach relies on the fact that during B-cell development, the V, D, and J segments of the immunoglobulin genes are randomly rearranged, creating a unique gene sequence for each cell and its progeny. A similar process occurs in the genes encoding the components of the T-cell receptor. Because ALL arises from a single lymphoid precursor, all the cells in any one case of ALL will have the same immunoglobulin or T-cell receptor rearrangement, which can be used to distinguish the malignant cells from normal. For measurement of MRD, the specific rearrangement must be sequenced in the case upfront, but once this is done, an assay exists that has a sensitivity as low as 0.001%. An alternative approach is to use PCR assays of gene fusions, such as BRC-ABL or MLL-AFF1. Although this technique is much less laborious and does not require the patient-specific primers needed with the immunoglobulin and T-cell receptor approach, gene fusions are present in no more than 50% of adult ALL cases, making this approach unavailable for most patients.