Chapter 21 Vitreous and Vitreoretinal Interface
Biochemistry of vitreous
Collagen
Collagen is the major structural protein, consisting of heterotypic fibrils (Fig. 21.1) similar to cartilage.1,2 Following vitrectomy, a type II procollagen is secreted in humans,3 but vitreous is not reformed, since reoperations reveal that the gel state of vitreous is not re-established.
Type II collagen4 comprises 75% of the total collagen content in vitreous. There are considerable similarities between vitreous and cartilage collagens,5,6 perhaps explaining why inborn errors of type II collagen metabolism result in “arthro-ophthalmopathies,”7 manifesting similar phenotypic expression in joints and vitreous. Type IX collagen accounts for up to 15% of vitreous collagen,8 where it always contains a chondroitin sulfate glycosaminoglycan chain9 covalently linked to the α2 (IX) chain at the NC3 domain, enabling the molecule to assume a proteoglycan form.
An important function of vitreous is maintaining transparency within the eye (Fig. 21.2).2 Studies10 have shown that one of the minor collagens of vitreous is type XVIII, progenitor of endostatin, a potent inhibitor of angiogenesis.11
Hyaluronan
Hyaluronan (HA) was first isolated from bovine vitreous in 1937. HA appears after birth, perhaps synthesized by hyalocytes,2 the ciliary body, and/or Müller cells. It is a large polyanion, which can influence the diffusion of drugs through vitreous.12,13 As a result of HA’s entanglement within the vitreous collagen fibril matrix, the mechanical force of HA’s extension and contraction can be transmitted to the retina, optic disc, and neovascular complexes, inducing untoward effects in conditions with fluctuations in ionic balance and hydration, such as diabetes.14
Chondroitin sulfate
Most vitreous chondroitin sulfate is in the form of versican,15 believed to form complexes with HA as well as with microfibrillar proteins such as fibulin-1 and fibullin-2 and play a crucial role in maintaining the molecular morphology of vitreous.16 Mutations that alter the splicing of the central chondroitin sulfate-bearing domains of versican have been implicated in Wagner syndrome, a condition with excess vitreous liquefaction.17
Noncollagenous structural proteins
Fibrillins
In Marfan syndrome defects in the gene encoding fibrillin-1 (FBN1 on chromosome 15q21) result in both ectopia lentis and vitreous liquefaction,18 important in rhegmatogenous retinal detachment (RD) in these patients.
Opticin
A major noncollagenous protein of vitreous is opticin (formerly vitrican).19 It is bound to the surface of the heterotypic collagen fibrils and prevents aggregation of adjacent collagen fibrils into bundles. Opticin binds heparan and chondroitin sulfates, suggesting that opticin may play a role in vitreoretinal adhesion.20,21 Similar to its role in articular cartilage,22 opticin may also stabilize vitreous gel structure by binding chondroitin sulfate proteoglycans.
Anatomy and histology
Vitreous body
Vitreous is a clear gel-like structure with a volume of 4.0 mL. During invagination of the optic vesicle the “primary” vitreous forms between the lens and the internal limiting lamina (ILL on Fig. 21.3A) of the retina. It is noteworthy that the ILL is continuous with Bruch’s membrane, demonstrating a common embryologic origin with analogous molecular composition and structure, suggesting important similarities later in life.23 The “secondary” vitreous begins to develop at the 13-mm stage of embryogenesis and is derived from the retina and mesoderm of the hyaloid vascular system (Fig. 21.3B).
Classic depictions of human vitreous structure are shown in Fig. 21.4. Modern concepts proposed membranous (Fig. 21.5A)24 and cisternal (Fig. 21.5)25 systems. Sebag and Balazs26 performed dark-field slit microscopy to define the posterior vitreous cortex as a thin, membranous structure continuous from the ora serrata to the posterior pole. Two round holes are present in the prepapillary and premacular areas (Fig. 21.6). Anteroposterior fibers (Fig. 21.7) comprised of parallel collagen fibrils (Fig. 21.8) arise from the vitreous base (Fig. 21.9A), where Gartner27 found “lateral aggregation” in older individuals. Vitreous base collagen fibers insert anterior to the ora serrata forming the anterior loop (Fig. 21.9B), important in anterior proliferative vitreoretinopathy (PVR).28 In the posterior pole, fibers extend through the premacular hole (Figs 21.6 and 21.7A), but a few attach to the rim of the hole. Condensed bundles of fibers insert into the vitreous cortex in the midperiphery and equator (Fig. 21.10).
Vitreoretinal interface
Posterior vitreous cortex
The posterior vitreous cortex is 100–110 µm thick and consists of densely packed collagen fibrils29 (Fig. 21.11). There is no vitreous cortex over the optic disc (Figs 21.6 and 21.7A), and the cortex is thin over the macula. The prepapillary hole can sometimes be visualized clinically following posterior vitreous detachment (PVD). If peripapillary tissue is torn away during PVD and remains attached around the prepapillary hole, it is called Vogt’s or Weiss’s ring. Gupta et al.30 demonstrated a lamellar organization of the posterior vitreous cortex (Fig. 21.12), confirmed in humans by three-dimensional optical coherence tomography (OCT) (Fig. 21.13).31 During anomalous PVD (APVD)32 these predispose to splitting along potential cleavage planes, resulting in vitreoschisis.33
Hyalocytes
Hyalocytes are mononuclear cells embedded in the posterior vitreous cortex 20–50 µm from the ILL posteriorly (Figs 21.6 and 21.14). The highest density of hyalocytes is in the vitreous base followed by the posterior pole, with the lowest density at the equator.34,35 Balazs36 suggested that these cells synthesize vitreous HA,37–40 but Swann5 disagreed. Evidence suggests that hyalocytes maintain ongoing synthesis and metabolism of glycoproteins41,42 and may also synthesize collagen43 and enzymes.44
The phagocytic capacity of hyalocytes has been described in vivo45 and demonstrated in vitro.46–48 Hyalocytes become phagocytic in response to inducting stimuli and are important in antigen processing and as initiators of the immune response, making possible intravitreal inoculation of antigens to promote systemic immunity.49 HA may have a regulatory effect on hyalocyte phagocytic activity.50,51 Various constituents of vitreous52,53 may be immunogenic and play a role in ocular inflammatory diseases. Sakamoto and Ishibashi have recently published an excellent review of hyalocytes.54
Hyalocytes are important in macular pucker when APVD28 and vitreoschisis26,29 leave these cells on the macula (Fig. 21.15). Under the influence of cytokines, hyalocytes proliferate55 on the surface of the retina, resulting in hypercellular membranes. Hyalocytes also recruit cells from the circulation and the retina (glial cells) via the release of connective tissue growth factor and induce collagen gel contraction in response to platelet-derived growth factor and other cytokines,56,57 causing tangential vitreoretinal contraction.
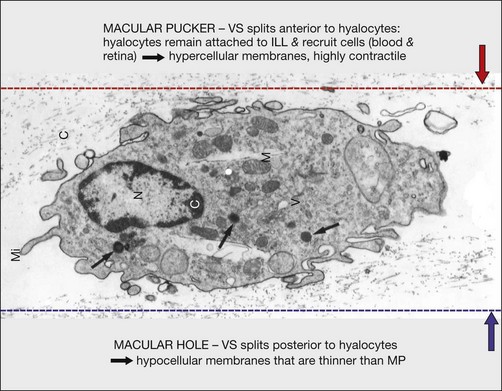
Fig. 21.15 Vitreoschisis (VS). Transmission electron microscopy of human hyalocyte (same as in Fig. 21.14B) demonstrating two potential levels splitting during vitreoschisis. Anomalous posterior vitreous detachment that induces vitreoschisis which splits the posterior vitreous cortex anterior to the level of hyalocytes (red dashes) leaves these cells attached to the macula, resulting in a hypercellular membrane. The lack of attachment to the optic disc allows inward (centripetal) tangential traction causing contraction and macular pucker. If vitreoschisis splits the posterior vitreous cortex posterior to the level of hyalocytes (blue dashes), the remaining membrane is thin and hypocellular. If there is also vitreopapillary attachment, the tangential forces will be outward (initially nasally), opening a central dehiscence and inducing a macular hole. ILL, internal limiting lamina; VS, vitreoschisis; MP, macular pucker; for other abbreviations see Fig. 21.14.
Internal limiting lamina (ILL) of the retina
The ILL is a multilaminar structure of variable thickness topographically. Adjacent to Müller cell foot plates is the lamina rara externa (0.03–0.06 µm) with no species variations or changes with topography or age. The lamina densa is thinnest at the fovea (0.01–0.02 µm) and thicker in the posterior pole (0.5–3.2 µm) than at the equator or vitreous base, where Foos58 found the ILL to be uniformly thin (51 nm) and the lamina rara to be 40 nm wide with traversing fibrils that were denser at sites corresponding to attachment plaques in Müller cells. The ILL is very thin over major retinal vessels (Fig. 21.16) where defects allow glial cells to extend on to the inner retina.59 Acquired ILL defects are in the foveola, retinal pits, retinal tufts, and retinal lattice. The ILL progressively thickens posteriorly to about 306 nm at the equator and about 1887 nm posteriorly. Müller footplates are less numerous at the equator than at the vitreous base. Posteriorly, no Müller footplates were observed and the inner aspect of the ILL remains smooth, while the outer aspect is irregular. Peripherally, both inner and outer surfaces are smooth.2,53
The ILL consists of type IV collagen, associated with glycoproteins,23,60,61 type VI collagen, which may contribute to vitreoretinal adhesion, and type XVIII,62 which binds opticin.63 Opticin binds to heparan sulfate, contributing to vitreoretinal adhesion.64 Type XVIII collagen also prevents cell migration from the retina into vitreous.65
Retinal sheen dystrophy66
This ILL dystrophy has cystic spaces under the ILL and in the inner nuclear layer (Fig. 21.17), and numerous areas of separation of the ILL from the retina with filamentous material (Fig. 21.18). Endothelial cell swelling and degeneration, pericyte degeneration, and basement membrane thickening of retinal capillaries suggest that this condition is primarily a retinal vasculopathy with edema, swelling, and degeneration of Müller cells. Alternatively, the primary defect could be in Müller cells.
Degenerative remodeling
Foos67 defined a spectrum of changes in the ILL as “degenerative remodeling.” Features include detachment and discontinuity of the ILL with vitreous collagen beneath the ILL (Fig. 21.19), cellular debris with macrophages, and absence of Müller cell attachment plaques. In larger lesions, vitreous may insinuate into degenerative crypts and adhere to the cell membrane of the lining Müller cells that have no basal lamina (Fig. 21.20). In the peripapillary area, retinal glial cells extend from the optic disc and are continuous with a glial epipapillary membrane that has vitreous fiber incarceration. Roth and Foos68 observed nasal epipapillary membranes associated with Bergmeister papillae in 27.6% of autopsy eyes.
Vitreoretinal interface
The interface between vitreous and adjacent structures consists of a complex formed by the vitreous cortex and basal laminae which are firmly attached to their cells.69 The only part of vitreous not adjacent to a basal lamina is the annulus of the anterior vitreous cortex, which is directly exposed to the zonules and the aqueous humor of the posterior chamber, similar to the surface of articular cartilage, which is exposed to synovial fluid.48 Zimmerman and Straatsma70 claimed that there are fibrillar attachments between the posterior vitreous cortex and the ILL. The composition of these fibrillar structures is not known and their presence has never been confirmed.
It is currently believed that an extracellular matrix “glue” of fibronectin, laminin, and other extracellular matrix components71 exists between vitreous and retina, causing adhesion to be fascial, as opposed to focal.55,56 Chondroitin sulfate is present at the sites of strong vitreoretinal adhesion such as the vitreous base and optic disc, forming the rationale for pharmacologic vitreolysis using avidin–biotin complex chondroitinase.
Topographic variations
Strength of vitreoretinal adhesion
Vitreous is attached to all contiguous structures, but is most firmly adherent at the vitreous base,72 which includes the posterior 2 mm of the pars plana and extends 1–4 mm posterior to the ora serrata, varying with age73 and topography (more posterior temporally). There are also topographic differences posteriorly, with greater adhesion at the posterior pole than the equator60 (Fig. 21.21).
Peripheral fundus and vitreous base
The vitreous base is a three-dimensional structure that straddles the ora serrata. There is a high density of collagen fibrils oriented at right angles to the inner surface of the ciliary epithelium and peripheral retina. The fibrils attach to the basement membrane of the nonpigmented epithelium of the pars plana and the ILL of the peripheral retina,74 intimately interwoven with an intervening extracellular matrix. Within the vitreous base, there are several anatomic variations where vitreoretinal adhesion is firm75 and associated with retinal breaks76,77:
1. Ora bays70,75,78 (Fig. 21.22)
2. Meridional folds77,79 (Fig. 21.23)
3. Meridional complexes77 (Figs 21.22 and 21.24)
4. Peripheral retinal excavations77,80 (Fig. 21.24)
6. Spiculate and nodular pigment epithelial hyperplasia87 (Fig. 21.28)
7. Retinal lattice “degeneration”58,84,89–105 (Figs 21.29–21.33)
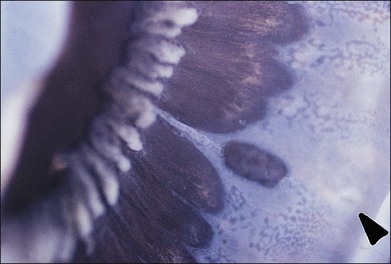
Fig. 21.24 Peripheral retinal excavation (arrowhead) in line with a meridional complex and an enclosed ora bay.
(Reproduced with permission from Green WR. Pathology of the retina. In: Frayer WC, editor. Lancaster course in ophthalmic histopathology, unit 9. Philadelphia: FA Davis; 1981.)
Interface along major retinal vessels
The ILL thins and is sometimes absent over major retinal vessels115,116 (Fig. 21.35). At such points, vitreous may be incarcerated into retina, directly continuous with perivascular tissue, attachments called “vitreoretinovascular bands,”117 or “spider-like bodies,”118 purported to be vitreous fibrils that traverse the ILL and coil about retinal blood vessels. It is common for retinal vessels to be associated with paravascular rarefaction (cystic degeneration), retinal pits and tears, and avulsion of retinal vessels.
Vitreomacular interface
Attachment of vitreous to the macula occurs in an irregular, annular zone of 3–4 mm diameter,2 generally not visible by clinical examination in normal adults but possibly evident in fetal and young adult eyes and in pathologic conditions. The posterior vitreous cortex is thinner over the macula in a disc-shaped area about 5 mm in diameter. Discontinuity of the ILL in the fovea may be a site where glial cells extend on to the inner surface of the retina (Fig. 21.36).
Vitreopapillary interface
At the rim of the optic disc the ILL ceases, although the basement membrane continues as the inner limiting membrane of Elschnig.119 This membrane is 50 nm thick and is believed to be the basal lamina of the astroglia in the optic nerve head. At the centralmost portion of the optic disc the membrane thins to 20 nm, follows the irregularities of the underlying cells of the optic disc, and is composed only of glycosaminoglycans and no collagen (central meniscus of Kuhnt). Given that the ILL prevents the passage of cells,47 the thinness and chemical composition of the central meniscus of Kuhnt and the membrane of Elschnig may account for frequent cell proliferation from or near the optic disc.
Vitreous attachment to the optic disc may persist even though the vitreous is detached elsewhere120 (Fig. 21.37). This adhesion may be fortified by epipapillary membranes.68 The entire complex may subsequently detach, resulting in a ring of tissue composed of fibrous astrocytes and collagen (Fig. 21.38) that flutters in and out of the visual axis, causing “floaters.” Vitreopapillary adhesion (VPA) contributes to macular holes121 and any vitreomaculopathy that features intraretinal cystoid spaces,122 presumably due to the influence upon the vectors of tangential traction upon the macula.
Physiology
Biochemical
Vitreous is important in maintaining transparency for maximal photon transmission to the retina. Vitreous may also maintain lens transparency by mitigating the effects of reactive oxygen species on lens proteins and thus preventing cataracts.123 This antioxidant effect is primarily the result of high concentrations of ascorbate in vitreous, an observation originally made in 1944 by Friedenwald and colleagues.124
Gisladottir et al.125 recently emphasized the influence of vitreous on various physiologic processes and showed that vitrectomy can have considerable effects, both beneficial and harmful.126 Vitrectomy reduces the risk of retinal neovascularization, but increases the risk of iris neovascularization, reduces macular edema, but stimulates cataract formation.
Biophysical
During ocular saccades, the rotational force of the eye wall is transmitted to the vitreous body via attachments to adjacent structures.127 During both acceleration and deceleration phases of saccades, vitreous movement lags behind the eye wall, resulting in markedly reduced acceleration.128 This “slack and lag” results from vitreous viscoelasticity, dampening the force at any given internal vitreous attachment. The inferonasal displacement of the optic disc and the shorter distance between the disc and ora inferiorly and nasally make the relief of torsional strain on equatorial and anterior vitreoretinal attachments greater nasally and inferiorly. Accordingly, the greatest torsional strain on anterior and equatorial vitreous attachments should occur during lateral saccades, with the point of maximum strain located somewhere in the superotemporal quadrant, the site of most frequent retinal tears.129
Age-Related Vitreous Degeneration
Liquefaction (synchysis)130,131
After age 40 years there is a significant decrease in the gel volume and a concurrent increase in the liquid volume of vitreous, primarily centrally.132,133 In the posterior vitreous such changes form pockets of liquid vitreous, called “lacunae” (Fig. 21.39). When a single, large pocket forms, the terms “bursa”24 or “precortical pocket”134 have been employed. This large posterior lacuna is a manifestation of age-related liquefaction (Fig. 21.39), and not an anatomic entity.135 Balazs and Denlinger130 found evidence of liquid vitreous after the age of 4 years and observed that, by the time the human eye reaches adult size (ages 14–18 years), 20% of the total volume is liquid vitreous. By the age of 80–90 years more than half the vitreous is liquid.
Pathogenesis of vitreous liquefaction
Changes in collagen136,137 or the conformation of HA with subsequent cross-linking of and aggregation of fibrils into bundles may result in vitreous liquefaction. Free radicals generated by metabolism and/or photons alter vitreous macromolecules and trigger dissociation of collagen from HA, leading to liquefaction.138 Vitreous liquefaction may also result from changes in the minor glycosaminoglycans and chondroitin sulfate profile of vitreous.139
Aging changes and vitreous biochemistry
Biochemical studies support the rheologic observations described above. Total vitreous collagen content does not change after ages 20–30 years. However, collagen concentration in gel vitreous at the ages of 70–90 years (0.1 mg/mL) was significantly greater than at the ages of 15–20 years (0.05 mg/mL; P < 0.05).130 Since the total collagen content does not change, this is likely due to the decreased volume of gel vitreous that occurs with aging and a consequent increase in the concentration of the collagen in the remaining gel. This concept is supported by the finding that vitreous HA concentration increases until about the age of 20, when adult levels are attained. Thereafter, until 70 years, there are no changes in the HA concentrations of either the liquid or gel compartments. This necessarily means that there is an increase in the HA content of liquid vitreous and a concomitant decrease in the HA content of gel vitreous, since the amount of liquid vitreous increases and the amount of gel vitreous decreases with age.
Structural changes
Vitreous body
The aforementioned rheologic and biochemical alterations induce significant structural changes during aging, consisting of a transition from a clear gel in youth (Fig. 21.2) to a fibrous structure in adults (Fig. 21.7A). In old age there is advanced liquefaction with thickening and tortuosity of vitreous fibers, and collapse (syneresis) of vitreous (Fig. 21.40). Postmortem studies140 found syneresis in 70% of subjects in the eighth decade. Syneresis occurs earlier and is more extensive in myopic eyes,141 and is accelerated with inflammation, trauma, and arthro-ophthalmopathies.7,142
Aging changes at the vitreoretinal interface
Teng and Chi73 found that the width (in the radial dimension) of the vitreous base posterior to the ora serrata increases with age to over 3.0 mm. There is also posterior migration of the posterior border of the vitreous base with age,73,103 mostly temporally. In addition, Gartner143 found “lateral aggregation” of the collagen fibrils in the vitreous base of older individuals. These changes play important roles in the pathogenesis of peripheral retinal breaks and rhegmatogenous RD.
Posterior vitreous detachment
Due to inadequate diagnostics,144 PVD is an inaccurate diagnosis. PVD begins at the posterior pole, perhaps in the perifoveal region.145 An innocuous PVD is clean separation between the ILL of the retina and the cortical vitreous.146 Whereas it is widely held that PVD is an “abnormal” event, it is possible that PVD may be a preprogrammed event that mitigates the risks of an attached vitreous, which in old age are more dangerous than a PVD.147 Fortunately, PVD is innocuous in most cases.
Epidemiology
The incidence of PVD is 66% between the ages of 66 and 86 years,148 and 53% after 50 years.149 Clinical examination,150 ultrasonography,151 and monochromatic photography152 have been standard, but nanotechnologies such as dynamic light scattering,153,154 are being developed to improve clinical evaluation.
In a postmortem study155 of 786 subjects aged >20 years, an upside-down suspension-in-air technique detected a 41% incidence of PVD over 65 years of age. Of 62 aphakic eyes, 94% had partial or complete PVD.
Symptomatic PVD
The sudden onset of “floaters” heralds the onset of PVD. Floaters have a significant negative impact on the quality of life.156 The incidence of retinal tears in patients with acute symptomatic PVD varies from 8 to 15%, to as high as 46%.157 In a study158 of 589 patients with “floaters,” diffuse dots, vitreous cells, and hemorrhage were high-risk factors for a retinal tear, since 93 of 176 (52.8%) of eyes with one or more of these risk factors had retinal tears. Novak and Welch159 reported that, in 172 eyes of 155 patients with acute symptomatic PVD, 31% had complications. Of these 155 patients, PVD developed in the fellow eye in 17 (11%) within 2 years.
Anomalous PVD (APVD)
APVD results from gel liquefaction without concurrent weakening of vitreoretinal adherence, causing various clinical manifestations based upon where vitreous is most liquefied and where the interface is most firmly adherent (Fig. 21.41).
Vitreous effects of APVD
An important consequence of APVD is vitreoschisis29,32 (Fig. 21.42), i.e., splitting of the posterior vitreous cortex with forward displacement of the anterior portion of the cortex, leaving the posterior layer attached to the retina. Vitreoschisis has been detected in proliferative diabetic retinopathy,14 macular pucker, and macular holes.160
Peripheral retinal effects of APVD
Retinal breaks
Retinal holes unrelated to PVD were observed in 326 (13.9%) of 2334 autopsy cases by Foos et al.96 Retinal tears (Fig. 21.43) result from fluid movements.161 Vitreous remains attached to the posterior margin of the retinal flap, which may be avulsed leaving a round or oval hole. The flap of retina remains attached to the posterior surface of the detached vitreous (operculum). Large detached flaps may form a cystic structure (Fig. 21.44).
The clinical incidence of retinal tears162 varies from 7.2%163 to 5.8%,164 with a high of 13.75%79 and a low of 0.59%.165 Postmortem incidences were 3.9%,166 8.6%,167 4.7%,168 8.8%,169 3.7%,170 and 7.3%.96 Although the role of retinal tears in causing RD is undisputed, management is controversial. Byer171 concluded that prophylactic treatment is not justified for asymptomatic retinal breaks in phakic eyes. However, in a natural history study of 166 eyes with retinal breaks, Davis105 observed that 31 (18%) progressed to RD. Neumann and Hyams172 reported that 2% of 153 eyes with retinal breaks developed RD. The incidence of retinal tears is much greater than RD, which varies between 9 (0.009%)173 and 24.4 (0.02%) per 100 000 per year.174 Benson175 promoted patient education while Combs and Welch176 concluded that prophylactic treatment of acute horseshoe tears with vitreous traction significantly reduces the incidence of RD. A particularly high-risk group are patients with vitreous hemorrhage that obscures fundus visualization. Ultrasound may be an effective means of identifying retinal tears in such eyes,177 but misdiagnosis at presentation bodes poorly, since there is a 67% incidence of retinal tears.178
Other sequelae
Macular effects of APVD
Exudative age-related macular degeneration
Recent studies181,182 determined that vitreomacular adhesion may be a risk factor for exudative age-related macular degeneration. Several subsequent studies have confirmed these findings.
Macular cysts190
Macular cysts that result from chronic edema (Fig. 21.51) need to be distinguished from the cystoid spaces created by vitreous traction in macular holes (Figs 21.52 and 21.53), and macular pucker with VPA.121
Macular holes
Macular holes are surrounded by a gray ring of cystoid spaces and slightly elevated retina, thinning and depigmentation of the underlying retinal pigment epithelium (RPE), yellow nodular opacities at the level of the RPE, a preretinal membrane in all cases with eccentric retinal pucker in 40%, and an operculum in 25% of patients (Fig. 21.53). Macular hole occurred in 8 of 37 (22%) fellow eyes in one study.191 Avila et al.192 reported axial traction as the cause, while Morgan and Schatz193 proposed that involutional macular thinning predisposes to a macular hole.
Histopathology and pseudo-operculum
Minor RPE hypertrophy and hyperplasia occur in the area of lamellar and full-thickness macular hole. At the onset of macular hole, there is likely vitreoschisis,32 leaving a thin hypocellular layer of the posterior vitreous cortex attached to the macula147,160,194 (Fig. 21.54). There is little cellular proliferation,195 suggesting that fluid countercurrents or vitreoschisis (Fig. 21.55) may be important. Healing of macular hole following surgery196 does, however, involve glial cell proliferation197 and Müller cell processes.198 Macular hole opercula are rarely composed of retinal tissue (Fig. 21.53), hence the name “pseudo-operculum.”
Pathogenesis
There have been various theories of macular hole pathogenesis: trauma, foveal degeneration, vitreous traction, and involutional thinning with PVD. It is clear from surgical experience199 that vitreous is the cause of macular hole. Johnson and Gass200 formulated the tangential traction theory by suggesting that shrinkage of the prefoveal vitreous induces macular hole formation in four stages. There are three possible mechanisms of tangential vitreous traction: (1) fluid vitreous movements and countercurrents; (2) cellular remodeling of cortical vitreous; and (3) contraction of a cellular membrane on the tapered cortical vitreous after vitreoschisis.27,31,32 OCT scanning laser ophthalmoscopy (OCT-SLO) imaging found vitreoschisis in half of eyes with macular hole29,160 (Fig. 21.56). In an ultrastructural study of epiretinal tissue removed during vitrectomy for impending macular holes, Smiddy et al.187 observed cortical vitreous in all eyes. VPA may be important, as this is present in 88.2% of macular hole eyes.121,122 VPA influences the vector of tangential forces on the macula and induces outward (centrifugal) traction, opening a central dehiscence. In macular pucker, there is usually no VPA and the vector of tangential traction is inward (centripetal), causing a macular pucker.
Some surgeons201 have advocated prophylactic surgery in symptomatic fellow eyes. However, a multicenter trial failed to demonstrate efficacy for vitrectomy in such eyes,202 and thus surgery is not routinely performed.
Optic disc effects
Anomalous PVD with persistent adhesion to the optic disc can cause vitreopapillary traction inducing hemorrhage,203 exacerbating neovascularization in proliferative diabetic vitreoretinopathy,204 and even inducing gaze-evoked visual disturbances.205 Vitreopapillary adhesion also plays a role in the formation of macular holes and cysts.121,122
Vitreoretinal Changes after Lens Extraction
Structural
2. Hemorrhage (APVD) with subsequent liquefaction.
3. Vitreous incarceration in the wound and vitreoretinal traction207 (Fig. 21.57).
Biochemical
Reduction of vitreous HA concentration208 results in decreased viscosity209 and shock absorption. This can be prevented by maintaining an intact posterior capsule.
PVD210,211
PVD occurred in 84% following intracapsular cataract extraction, 76% following extracapsular cataract extraction (ECCE) and surgical capsular discission, and in 40% of eyes following ECCE with an intact posterior capsule. The incidence of rhegmatogenous RD increases following YAG capsulotomy.212
Vitreoretinal changes after trauma
Blunt trauma
Blunt trauma may be transmitted to the retina in a direct and contrecoup fashion, resulting in a variety of rhegmatogenous sequelae (Fig. 21.58).213 Dialysis at the anterior border of the vitreous base typically occurs inferonasally. Less common are avulsion of the vitreous base and retinal dialysis at the posterior border of the vitreous base. Concussive forces following blunt trauma can induce retinal edema (commotio retinae).
Posterior penetrating and perforating trauma215
Wound healing at the perforation site allows fibrocellular proliferation into the eye, inducing traction RD (Fig. 21.60). Histopathologic studies revealed cyclitic and periretinal membranes.216 Intraocular proliferation starts 2–4 days after injury,217 PVD develops at 1–2 weeks,218 and traction RD occurs at 7–11 weeks. Poliferation can be prevented by vitrectomy,219 less hazardous after 2 weeks because of the development of PVD220,221 and more effective if complete.222 Yet Miller et al.223 found that vitreous plays a role in normal healing of retinal wounds.
Periretinal proliferation
Premacular membranes
Primary premacular membranes occur in the absence of associated conditions and are most likely the result of vitreoschisis,32 where APVD31 splits the posterior vitreous cortex leaving the outermost layer attached to the macula. The level of this split can vary, as do the consequences from no effects, such as in the case of so-called simple epiretinal membranes (Fig. 21.61) which have no contractile features, to macular pucker.147,160 Smiddy et al.224 observed the principal cell to be RPE in these cases, although it is likely that many of these cells are actually hyalocytes (Figs 21.6 and 21.14) and circulating monocytes recruited from retinal and choroidal vessels.30,160 Secondary premacular membranes occur in association with inflammation, accidental or surgical trauma, and retinovascular disease. Fibrous astrocytes are the predominant cell in secondary preretinal macular membranes (PMMs).
Macular pucker (Fig. 21.62) results from PMMs that induce centripetal tangential (inward to the fovea) traction upon the macula. Macular pucker can have as many as four separate centers of retinal contraction225 (Fig. 21.63). Eyes with three or four contraction centers had significantly more macular thickening and a higher prevalence of intraretinal cysts than eyes with one or two contraction centers.
Retroretinal membranes
These membranes typically occur after RD when RPE grows in sheets over the undersurface of the retina. Strands of fibrous tissue contract and prevent retinal reattachment.226
Complex membranes
Complex membranes (Fig. 21.64) develop after RD surgery or after trauma. Commonly called PVR,227 contraction of this proliferative tissue causes traction RD. Anterior PVR features anterior-loop contraction228 (Fig. 21.9).
Histopathologically, retinal glial cells gain access to the inner retinal surface via ILL discontinuities, such as at the optic disc, foveola, along major vessels, and at retinal tufts. Acquired sites of ILL discontinuity include retinal tags, pits (lamellar holes), holes, and tears (Fig. 21.65), avulsed retinal vessels, areas of degenerative remodeling, and retinal lattice. Retinal glial cells are the principal cells in membranes at the optic disc, in simple PMMs, in most secondary membranes associated with inflammatory diseases or retinovascular disease, after photocoagulation or RD surgery, and in retinitis pigmentosa. RPE gains access to the vitreous via retinal breaks, the ora serrata, and retinal lattice. RPE can also migrate through intact retina. RPE is the principal cell in PVR (Fig. 21.66), but glial cells are present in about 50% of cases. Myofibrocytes (Fig. 21.67) were observed by electron microscopy in 91% of membranes studied by Kampik et al.,229 and actin has been observed in cells of PVR membranes.230
Incomplete vitrectomy, intraoperative hemorrhage,217 and excessive cryopexy231 render most cases of PVR an iatrogenic disease. Fibronectin and platelet-derived growth factor are the chemoattractants that stimulate migration of RPE232 and glial cells.233 Incomplete vitrectomy leaves behind hyalocytes (Figs 21.6 and 21.14), which are also the first cells to be exposed to these growth factors and other stimuli. When stimulated, these cells become phagocytic (Fig. 21.68). These resident macrophages play an important role in early PVR pathogenesis. Thus, targeting these cells for pharmacotherapy, similar to what has been done during vitrectomy for RD,234 may significantly mitigate PVR. Alternatively, eliminating the role of vitreous via pharmacologic vitreolysis235 will have a great impact in PVR and all aforementioned conditions.
1 Eyre DR, Apon S, Wu JJ, et al. Collagen type IX: Evidence for covalent linkages to type II collagen in cartilage. Fed Eur Biochem Soc. 1987;220:337.
2 Sebag J. The vitreous: structure, function, and pathobiology. New York, NY: Springer-Verlag; 1989. p. 63–4
3 Itakura H, Kishi S, Kotajima N, et al. Embryonic synthesis of the inner limiting membrane and vitreous body. Graefes Arch Clin Exp Ophthalmol. 2005;243:994–998.
4 Schmut O, Mallinger R, Paschke E. Studies on a distinct fraction of bovine vitreous body collagen. Graefes Arch Clin Exp Ophthalmol. 1984;221:286.
5 Swann DA. Chemistry and biology of vitreous body. Int Rev Exp Pathol. 1980;22:1.
6 Snowden JM. The stabilization of in vivo assembled collagen fibrils by proteoglycans/glycosaminoglycans. Biochem Biophys Acta. 1982;703:21.
7 Maumenee IH. Vitreoretinal degeneration as a sign of generalized connective tissue diseases. Am J Ophthalmol. 1979;88:432–449.
8 Bishop PN, Crossman MV, McLeod D, et al. Extraction and characterisation of the tissue forms of collagen types II and IX from bovine vitreous. Biochem J. 1994;299:497–505.
9 Bishop PN, Reardon AJ, McLeod D, et al. Identification of alternatively spliced variants of type II procollagen in vitreous. Biochem Biophys Res Commun. 1994;203:289.
10 Bhutto IA, Kim SY, McLeod DS, et al. Localization of collagen XVIII and the endostatin portion of collagen XVIII in aged human control eyes and eyes with age-related macular degeneration. Invest Ophthalmol Vis Sci. 2004;45:1544–1552.
11 Ohlmann AV, Ohlmann A, Welge-Lussen U, et al. Localization of collagen XVIII and endostatin in the human eye. Curr Eye Res. 2005;30:27–34.
12 Kim H, Robison SB, Csaky KG. Investigating the movement of intravitreal human serum albumin nanoparticles in the vitreous and retina. Pharm Res. 2009;26:329–337.
13 Comper WD, Laurent TC. Physiological functions of connective tissue polysaccharides. Physiol Rev. 1978;58:255.
14 Sebag J. Diabetic vitreopathy. Ophthalmology. 1996;103:205.
15 Reardon A, Heinegard D, McLeod D, et al. The large chondroitin sulphate proteoglycans versican in mammalian vitreous. Matrix Biol. 1998;17:325–333.
16 Cain SA, Morgan A, Sherratt MJ, et al. Proteomics analysis of fibrillin-rich microfibrils. Proteomics. 2006;6:111–122.
17 Miyamoto T, Inoue H, Sakamoto Y, et al. Identification of a novel splice site mutation of the CSPG2 gene in a Japanese family with Wagner syndrome. Invest Ophthalmol Vis Sci. 2005;46:2726–2735.
18 Bishop PN. Structural macromolecules and supramolecular organization of the vitreous gel. Prog Retinal Eye Res. 2000;19:323.
19 Reardon AJ, LeGoff M, Briggs MD, et al. Identification in vitreous and molecular cloning of opticin, a novel member of the family of leucine-rich repeat proteins of the extracellular matrix. J Biol Chem. 2000;275:2123.
20 Sanders EJ, Walter MA, Parker E, et al. Opticin binds retinal growth hormone in the embryonic vitreous. Invest Ophthalmol Vis Sci. 2003;44:5404–5409.
21 Hindson VJ, Gallagher JT, Halfter W, et al. Opticin binds to heparan and chondroitin sulphate proteoglycans. Invest Ophthalmol Vis Sci. 2005;46:4417–4423.
22 Monfort J, Tardif G, Roughley P, et al. Identification of opticin, a member of the small leucine-rich repeat proteoglycan family, in human articular tissues. Osteoarthritis Cartilage. 2008;16:749–755.
23 Sebag J, Hageman GS. Interfaces. Eur J Ophthalmol. 2000;10:1.
24 Eisner G. Biomicroscopy of the peripheral fundus. New York: Springer-Verlag; 1979.
25 Worst JG. Cisternal systems of the fully developed vitreous body in the young adult. Trans Ophthalmol Soc UK. 1977;97:550–554. a
26 Sebag J, Balazs EA. Human vitreous fibres and vitreoretinal disease. Trans Ophthalmol Soc UK. 1984;104:123–128.
27 Gartner J. Electron-microscopic study on the fibrillar network and fibrocyte–collagen interactions in the vitreous cortex at the ora serrata of human eyes with special regard to the role of disintegrating cells. Exp Eye Res. 1986;42:21–23.
28 Sebag J. Surgical anatomy of vitreous and the vitreoretinal interface. Duane’s clinical ophthalmology. Tasman W, Jaeger EA, eds. Duane’s clinical ophthalmology. Philadelphia: JB Lippincott; 1994;vol 6. updated 2008
29 Streeten BA. Disorders of the vitreous. In: Garner A, Klintworth GK. Pathobiology of ocular disease – a dynamic approach. New York: Marcel Dekker; 1982:1381–1419.
30 Gupta P, Yee KMP, Garcia P, et al. Vitreoschisis in macular diseases. Br J Ophthalmol. 2011;95:376–380.
31 Sebag J. Vitreous – the resplendent enigma. Br J Ophthalmol. 2009;93:989–991.
32 Sebag J. Anomalous PVD – a unifying concept in vitreoretinal diseases. Graefes Arch Clin Exp Ophthalmol. 2004;242:690–698.
33 Sebag J. Vitreoschisis. Graefes Arch Clin Exp Ophthalmol. 2008;246:329–332.
34 Balazs EA, Toth LZ, Eckl EA, et al. Studies on the structure of the vitreous body. Exp Eye Res. 1964;3:57–71.
35 Gloor BP. Cellular proliferation on the vitreous surface after photocoagulation. Graefes Arch Clin Exp Ophthalmol. 1969;178:99.
36 Balazs EA. Structure of vitreous gel. Acta XVII Concilium Ophthalmologicum. 1954;11:1019.
37 Balazs EA, Sundblad L, Toth LZJ. In vitro formation of hyaluronic acid by cells in the vitreous body and by comb tissue. Abstr Red Proc. 1958;17:184.
38 Jacobson B, Osterlin S, Balazs EA. A soluble hyaluronic acid synthesizing system from calf vitreous. Proc Fed Am Soc Exp Biol. 1966;25:588.
39 Osterlin SE. The synthesis of hyaluronic acid in the vitreous. III. In vivo metabolism in the owl monkey. Exp Eye Res. 1968;7:524.
40 Osterlin SE. The synthesis of hyaluronic acid in the vitreous. IV. Regeneration in the owl monkey. Exp Eye Res. 1969;8:27.
41 Rhodes RH, Mandelbaum SH, Minckler DS, et al. Tritiated glucose incorporation in the vitreous body, lens and zonules of the pigmented rabbit. Exp Eye Res. 1982;34:921.
42 Jacobson B. Identification of sialyl and galactosyl transferase activities in calf vitreous hyalocytes. Curr Eye Res. 1984;3:1033.
43 Newsome DA, Linsemayer TF, Trelstad RJ. Vitreous body collagen. Evidence for a dual origin from the neural retina and hyalocytes. J Cell Biol. 1976;71:59–67.
44 Hoffmann K, Baurwieg H, Riese K. Uber Gehalt und Verteilung niederund hoch molekularer Substanzen in Glaskorper. II. Hock molekulare Substanzen (LDH, MDH, GOT). Graefes Arch Clin Exp Ophthalmol. 1974;191:231.
45 Teng CC. An electron microscopic study of cells in the vitreous of the rabbit eye. Part I. The macrophage. Eye Ear Nose Throat Monthly. 1969;48:91.
46 Szirmai JA, Balazs EA. Studies on the structure of the vitreous body. 111. Cells in the cortical layer. Arch Ophthalmol. 1958;59:34.
47 Grabner G, Baltz G, Forster O. Macrophage-like properties of human hyalocytes. Invest Ophthalmol Vis Sci. 1980;19:333.
48 Balazs EA. Functional anatomy of the vitreous. In: Duane TD, Jaeger EA, editors. Biomedical foundations of ophthalmology, vol 1. Philadelphia: Harper and Row; 1982, p. 6–12.
49 Sonoda KH, Sakamoto T, Qiao H, et al. The analysis of systemic tolerance elicited by antigen inoculation into the vitreous cavity: vitreous cavity-associated immune deviation. Immunology. 2005;116:390–399.
50 Forrester JV, Balazs EA. Inhibition of phagocytosis by high molecular weight hyaluronate. lmmunology. 1980;40:435.
51 Sebag J, Balazs EA, Eakins KE, et al. The effect of Na-hyaluronate on prostaglandin synthesis and phagocytosis by mononuclear phagocytes. Invest Ophthalmol Vis Sci. 1981;20:33.
52 Hultsch E. Vitreous structure and ocular inflammation. In: Silverstein AM, O’Connor GR. Immunology and immunopathology of the eye. New York: Masson, 1979.
53 Gartner J. The fine structure of the vitreous base of the human eye and pathogenesis of pars planitis. Am J Ophthalmol. 1971;71:1317–1327.
54 Sakamoto T, Ishibashi T. Hyalocytes: essential cells of the vitreous cavity in vitreoretinal pathophysiology? Retina. 2011;31:222–228.
55 Nishitsuka K, Kashiwagi Y, Tojo N, et al. Hyaluronan production regulation from porcine hyalocyte cell line by cytokines. Exp Eye Res. 2007;85:539–545.
56 Kita T, Hata Y, Kano K, et al. Transforming growth factor-beta2 and connective tissue growth factor in proliferative vitreoretinal diseases: Possible involvement of hyalocytes and therapeutic potential of Rho kinase inhibitor. Diabetes. 2007;56:231–238.
57 Hiryama K, Hata Y, Noda Y, et al. The involvement of the rho-kinase pathway and its regulation in cytokine-induced collagen gel contraction by hyalocytes. Invest Ophthalmol Vis Sci. 2004;45:3896–3903.
58 Foos RY. Posterior peripheral retinal tears. Ann Ophthalmol. 1974;6:679–687.
59 Kishi S, Numaga T, Yoneya S, et al. Epivascular glia and paravascular holes in normal human retina. Graefes Arch Clin Exp Ophthalmol. 1986;224:124–130.
60 Sebag J. Age-related differences in the human vitreoretinal interface. Arch Ophthalmol. 1991;109:966.
61 Kefalides NA. The biology and chemistry of basement membranes. In: Kefalides NA, ed. Proceedings of the First International Symposium on the Biology and Chemistry of Basement Membranes. New York: Academic Press; 1978:215–228.
62 Fukai N, Eklund L, Marneros AG, et al. Lack of collagen XVIII/endostatin results in eye abnormalities. EMBO J. 2002;21:1535–1544.
63 Hindson VJ, Gallagher JT, Halfter W, et al. Opticin binds to heparan and chondroitin sulphate proteoglycans. Invest Ophthalmol Vis Sci. 2005;46:4417–4423.
64 Ramesh S, Bonshek RE, Bishop PN. Immunolocalisation of opticin in the human eye. Br J Ophthalmol. 2004;88:697–702.
65 Bhutto IA, Kim SY, McLeod DS, et al. Localization of collagen XVIII and the endostatin portion of collagen XVIII in aged human control eyes and eyes with age-related macular degeneration. Invest Ophthalmol Vis Sci. 2004;45:1544–1552.
66 Polk TD, Gass DM, Green WR, et al. Familial internal limiting membrane dystrophy: a new sheen retinal dystrophy. Arch Ophthalmol. 1997;115:878–885.
67 Foos RY. Vitreoretinal juncture: topographical variations. Invest Ophthalmol. 1972;11:801–808.
68 Roth AM, Foos RY. Surface structure of the optic nerve head. I. Epipapillary membranes. Am J Ophthalmol. 1972;74:977–985.
69 Cohen AI. Electron microscopic observations of the internal limiting membrane and optic fiber layer of the retina of the rhesus monkey. Am J Anat. 1961;108:179.
70 Zimmerman LE, Straatsma BR. Anatomic relationships of the retina to the vitreous body and to the pigment epithelium. In: Schepens CL, ed. Importance of the vitreous body in retina surgery with special emphasis on reoperation. St Louis: CV Mosby; 1960:15–28.
71 Russell SR, Shepherd JD, Hageman GS. Distribution of glycoconjugates in the human internal limiting membrane. Invest Ophthalmol Vis Sci. 1991;32:1986.
72 Fine BS, Tousimus AJ. The structure of the vitreous body and the suspensory ligaments of the lens. Arch Ophthalmol. 1961;65:95–110.
73 Teng CC, Chi HH. Vitreous changes and the mechanism of retinal detachment. Am J Ophthalmol. 1957;44:335–356.
74 Hogan MJ. The vitreous, its structure and relation to the ciliary body and retina. Invest Ophthalmol. 1963;2:418–445.
75 Spencer LM, Foos RY, Straatsma BR. Meridional complexes and associated abnormalities of the peripheral retina. Am J Ophthalmol. 1970;70:697–713.
76 Byer NE. Cystic retinal tufts and their relationship to retinal detachment. Arch Ophthalmol. 1981;99:1788–1790.
77 Spencer LM, Straatsma BR, Foos RY. Tractional degenerations of the peripheral retina. Transactions of the New Orleans Academy of Ophthalmology. Symposium on Retina and Retinal Surgery. St Louis: Mosby; 1969.
78 Spencer LM, Foos RY, Straatsma BR. Enclosed bays of the ora serrata: relationship to retinal tears. Arch Ophthalmol. 1970;83:421–425.
79 Rutnin U, Schepens CL. Fundus appearance in normal eyes. II. The standard peripheral fundus and developmental variations. Am J Ophthalmol. 1967;64:840–852.
80 Foos RY, Spencer LM, Straatsma BR. Trophic degenerations of the peripheral retina. Transactions of the New Orleans Academy of Ophthalmology. Symposium on retina and retinal surgery. St Louis: Mosby; 1969.
81 Streeten BW, Bert M. The retinal surface in lattice degeneration of the retina. Am J Ophthalmol. 1972;74:1201–1209.
82 Foos RY, Allen RA. Retinal tears and lesser lesions of the peripheral retina. Am J Ophthalmol. 1967;64:643–655.
83 Spencer LM, Straatsma BR, Foos RY. Tractional degenerations of the peripheral retina. Transactions of the New Orleans Academy of Ophthalmology. Symposium on Retina and Retinal Surgery. St Louis: Mosby; 1969.
84 Foos RY. Vitreous base, retinal tufts, and retinal tears: pathogenic relationships. In: Pruett RC, Regan CD. Retina congress. New York: Appleton-Century-Crofts, 1974.
85 Foos RY. Zonular traction tufts of the peripheral retina in cadaver eyes. Arch Ophthalmol. 1969;82:620–632.
86 Inomata H. Electron microscopic observations of cystoid degeneration in the peripheral retina. Jpn J Ophthalmol. 1966;10:26–40.
87 Green WR. Retina. In: Ryan SJ, ed. Ophthalmic pathology: an atlas and text, 4th edn. Philadelphia: WB Saunders, 1996.
88 Bradford JD, Wilkinson CP, Fransen SR. Pseudophakic retinal detachment: the relationships between retinal tears and the time following cataract surgery at which they occur. Retina. 1989;9:181–186.
89 Byer NE. Lattice degeneration of the retina. Surv Ophthalmol. 1979;23:213–247.
90 Straatsma BR, Zeegen PD, Foos RY, et al. Lattice degeneration of the retina. Trans Am Acad Ophthalmol Otolaryngol. 1974;78:87–113.
91 Semes LP. Lattice degeneration of the retina and retinal detachment. Optom Clin. 1992;2:71–91.
92 Burton TC. The influence of refractive error and lattice degeneration on the incidence of retinal detachment. Trans Am Ophthalmol Soc. 1990;87:143–157.
93 Parelhoff ES, Wood WJ, Green WR, et al. Radial perivascular lattice degeneration of the retina. Ann Ophthalmol. 1980;12:25–32.
94 Schepens CL. Diagnostic and prognostic factors as found in pre-operative examination. Trans Am Acad Ophthalmol Otolaryngol. 1952;56:398–418.
95 Schepens CL. Subclinical retinal detachments. Arch Ophthalmol. 1952;47:593–606.
96 Foos RY, Simons KB, Wheeler NC. Comparison of lesions predisposing to rhegmatogenous retinal detachment by race and subjects. Am J Ophthalmol. 1983;96:644–649.
97 Byer NE. Clinical study of lattice degeneration of the retina. Trans Am Acad Ophthalmol Otolaryngol. 1965;69:1064–1077.
98 Byer NE. Changes in prognosis of lattice degeneration of the retina. Trans Am Acad Ophthalmol Otolaryngol. 1974;78:114–124.
99 Foos RY, Simons KB. Vitreous in lattice degeneration of retina. Ophthalmology. 1984;91:452–457.
100 Robinson MR, Streeten BW. The surface morphology of retinal breaks and lattice retinal degeneration: a scanning electron microscopic study. Ophthalmology. 1986;93:237–246.
101 Tillery WV, Lucier AC. Round atrophic holes in lattice degeneration: an important cause of phakic retinal detachment. Trans Am Acad Ophthalmol Otolaryngol. 1976;81:509–518.
102 Morse PH, Scheie HG. Prophylactic cryoretinopexy of retinal breaks. Arch Ophthalmol. 1974;92:204–207.
103 Wang J, McLeod D, Henson DB, et al. Age-dependent changes in the basal retinovitreous adhesion. Invest Ophthalmol Vis Sci.. 2003;44:1793–1800.
104 Byer NE. Lattice degeneration of the retina. Surv Ophthalmol. 1979;23:213–247.
105 Davis MD. Natural history of retinal breaks without detachment. Arch Ophthalmol. 1974;92:183–194.
106 Dobbie G. Discussion. New research on the aetiology and surgery of retinal detachment. Mod Probl Ophthalmol. 1975;15:113.
107 Schepens CL. Diagnostic and prognostic factors as found in preoperative examination. Trans Am Acad Ophthalmol Otolaryngol. 1952;56:398–418.
108 Tolentino FI, Schepens CL, Freeman HM. Vitreoretinal disorders: diagnosis and management. Philadelphia: WB Saunders; 1976.
109 Byer NE. The peripheral retina in profile: a stereoscopic atlas. Torrence, CA: Criterion Press; 1982.
110 Watzke RC. The ophthalmoscopic sign, “white with pressure”: a clinicopathologic correlation. Arch Ophthalmol. 1961;66:812–823.
111 Daicker B. Sind die Symptome “weiss mit Druck” und weiss ohne Druck durch die Periphere Netzshantsklerose bedingt? Mod Probl Ophthalmol. 1975;15:82–90.
112 Gartner J. Discussion. New research on the aetiology and surgery of retinal detachment. Mod Probl Ophthalmol. 1975;15:112.
113 Freeman HM. Fellow eyes of giant retinal breaks. Trans Am Ophthalmol Soc. 1978;76:343–382.
114 Dunker S, Glinz J, Faulborn J. Morphologic studies of the peripheral vitreoretinal interface in humans reveal structures implicated in the pathogenesis of retinal tears. Retina. 1997;17:124–130.
115 Foos RY. Vitreoretinal juncture over retinal vessels. Graefes Arch Clin Exp Ophthalmol. 1977;204:223–234.
116 Wolter JR. Pores in the internal limiting membrane of the human retina. Acta Ophthalmol. 1964;42:971–974.
117 Kuwabara T, Cogan DG. Studies of retinal vascular patterns. I. Normal architecture. Arch Ophthalmol. 1960;64:904–911.
118 Mutlu F, Leopold IH. Structure of the human retinal vascular system. Arch Ophthalmol. 1964;71:93.
119 Heergaard S, Jensen OA, Prause JU. Structure of the vitreal face of the monkey optic disc (Macacca mulatta): SEM on frozen resin-cracked optic nerve heads supplemented by TEM and immunohistochemistry. Graefes Arch Clin Exp Ophthalmol. 1988;226:377.
120 Foos RY, Roth AM. Surface structure of the optic nerve head. II. Vitreopapillary attachments and posterior vitreous detachment. Am J Ophthalmol. 1973;76:662–671.
121 Wang MY, Nguyen D, Hindoyan N, et al. Vitreopapillary adhesion in macular hole and macular pucker. Retina. 2009;29:644–650.
122 Sebag J, Wang M, Nguyen D, et al. Vitreopapillary adhesion in macular diseases. Trans Am Ophthalmol Soc. 2009;107:35–46.
123 Shui Y-B, Holekamp NM, Kramer BC, et al. The gel state of the vitreous and ascorbate-dependent oxygen consumption – relationship to the etiology of nuclear cataracts. Arch Ophthalmol. 2009;127:475–482.
124 Friedenwald JS, Bushke W, Michel HO. Role of ascorbic acid in secretion of intraocular fluid. Arch Ophthalmol. 1944;29:535–574.
125 Gisladottir S, Loftsson T, Stefansson E. Diffusion characteristics of vitreous humour and saline solution follow the Stokes Einstein equation. Graefes Arch Clin Exp Ophthalmol.. 2009;247:1677–1684.
126 Stefansson E. Physiology of vitreous surgery. Graefes Arch Clin Exp Ophthalmol.. 2009;247:147–163.
127 Balazs EA. Physiology of the vitreous body. In: Schepens CL, ed. Proceedings of the Second Conference of the Retina Foundation. St Louis: Mosby, 1960.
128 Hilding AC. Alterations in the form, movement, and structure of the vitreous body in aphakic eyes. Arch Ophthalmol. 1954;52:699–709.
129 Schepens CL. Clinical aspects of pathologic changes of the vitreous body. Am J Ophthalmol. 1954;38:8–21.
130 Balazs EA, Denlinger JL. Aging changes in the vitreous. Aging and human visual function. New York: Alan R Liss; 1982.
131 O’Malley P. The pattern of vitreous syneresis: a study of 800 autopsy eyes. In: Irvine AR, O’Malley C. Advances in vitreous surgery. Springfield, IL: Charles C Thomas, 1976.
132 Eisner G. Zur Anatomie des Glaskorpers. Graefes Arch Clin Exp Ophthalmol. 1975;193:33–56.
133 Sebag J, Balazs EA. Morphology and ultrastructure of human vitreous fibers. Invest Ophthalmol Vis Sci. 1989;30:1867–1871.
134 Kishi S, Shimizu K. Posterior precortical vitreous pocket. Arch Ophthalmol. 1990;108:979–982.
135 Sebag J. Letter to the editor. Arch Ophthalmol. 1991;109:1059–1060.
136 Snowden JM. The stabilization of in vivo assembled collagen fibrils by proteoglycans/glycosaminoglycans. Biochem Biophys Acta. 1982;703:21–25.
137 Aguayo J, Glaser B, Mildvan A, et al. Study of vitreous liquefaction by NMR spectroscopy and imaging. Invest Ophthalmol Vis Sci. 1985;26:692.
138 Ueno N, Sebag J, Hirokawa H, et al. Effects of visible-light irradiation on vitreous structure in the presence of a photosensitizer. Exp Eye Res. 1987;44:863–870.
139 Kamei A, Totani A. Isolation and characterization of minor glycosaminoglycans in the rabbit vitreous body. Biochem Biophys Res Commun. 1982;109:881–887.
140 Foos RY, Wheeler NC. Vitreoretinal juncture: synchysis senilis and posterior vitreous detachment. Ophthalmology. 1982;89:1502–1512.
141 Goldmann H. Senile changes of the lens and the vitreous. Am J Ophthalmol. 1964;57:1–13.
142 Takahashi M, Jalkh A, Hoskins J, et al. Biomicroscopic evaluation and photography of liquefied vitreous in some vitreoretinal disorders. Arch Ophthalmol. 1981;99:1555–1559.
143 Gartner J. Electron microscopic observations on the cilio-zonular border of the human eye with particular reference to the aging changes. Anat Entwicklungsgesch. 1970;131:263.
144 Sebag J. To see the invisible: the quest of imaging vitreous. Dev Ophthalmol. 2008;42:5–28.
145 Johnson M. Perifoveal vitreous detachment and its macular complications. Trans Am Ophthalmol Soc. 2005;103:537–567.
146 Foos RY. Ultrastructural features of posterior vitreous detachment. Graefes Arch Clin Exp Ophthalmol. 1975;196:103–111.
147 Sebag J. Vitreous – the resplendent enigma. Br J Ophthalmol. 2009;93:989–991.
148 Favre M, Goldmann H. Zur Genese der hinteren Glaskorperabhebung. Ophthalmologica. 1956;132:86–97.
149 Pischel DK. Detachment of the vitreous as seen with slit-lamp examination. Trans Am Ophthalmol Soc. 1952;50:329–346.
150 Buzney SM, Weiter JJ, Furukawa H, et al. Examination of the vitreous: a comparison of biomicroscopy using the Goldmann and El Bayadi-Kajiura lenses. Ophthalmology. 1985;92:1745–1748.
151 Fisher YL, Slakter JS, Friedman RA, et al. Kinetic ultrasound evaluation of the posterior vitreoretinal interface. Ophthalmology. 1991;98:1135–1138.
152 Ortiz RG, Lopez PF, Lambert HM, et al. Examination of macular vitreoretinal interface disorders with monochromatic photography. Am J Ophthalmol. 1992;113:243–247.
153 Ansari RR, Dunker S, Suh K. Quantitative molecular characterization of bovine vitreous and lens with non-invasive dynamic light scattering. Exp Eye Res. 2001;73:859–866.
154 Sebag J, Dunker S, Suh KI, et al. Dynamic light scattering measurements in vitreous. Invest Ophthalmol Vis Sci. 1997;38:662.
155 Foos RY. Posterior vitreous detachment. Trans Am Acad Ophthalmol Otolaryngol. 1972;76:480–497.
156 Sebag J. Floaters and the quality of life. Am J Ophthalmol. 2011;152:3–4.
157 Tasman WS. Posterior vitreous detachment and peripheral retinal breaks. Trans Am Acad Ophthalmol Otolaryngol. 1968;72:217–224.
158 Boldrey EE. Risk of retinal tears in patients with vitreous floaters. Am J Ophthalmol. 1983;96:783–787.
159 Novak MA, Welch RB. Complications of acute symptomatic posterior vitreous detachment. Am J Ophthalmol. 1984;97:308–314.
160 Sebag J, Gupta P, Rosen R, et al. Macular holes and macular pucker: The role of vitreoschisis as imaged by optical coherence tomography/scanning laser ophthalmoscopy. Trans Am Ophthalmol Soc. 2007;105:121–131.
161 Machemer R. The importance of fluid absorption, traction, intraocular currents, and chorioretinal scars in the therapy of rhegmatogenous retinal detachments. Am J Ophthalmol. 1984;98:681–693.
162 Sigelman J. Vitreous base classification of retinal tears: clinical application. Surv Ophthalmol. 1980;25:59–74.
163 Halpern JI. Routine screening of the retinal periphery. Am J Ophthalmol. 1966;62:99–102.
164 Byer NE. Clinical study of retinal breaks. Trans Am Acad Ophthalmol Otolaryngol. 1967;71:461–473.
165 Smith RE, Ganley JP. Ophthalmic survey of a community. I. Abnormalities of the ocular fundus. Am J Ophthalmol. 1972;74:1126–1130.
166 Teng CC, Katzin KM. An anatomic study of the periphery of the retina. I. Nonpigmented epithelial cell proliferation and hole formation. Am J Ophthalmol. 1951;34:1237–1248.
167 Boniuk M, Butler FC. An autopsy study of lattice degeneration, retinal breaks, and retinal pits. In: McPherson A, ed. New and controversial aspects of retinal detachment. New York: Hoeber, 1968.
168 Spencer LM, Foos RY. Paravascular vitreoretinal attachments: role in retinal tears. Arch Ophthalmol. 1970;84:557–564.
169 Barishak YR, Stein R. Retinal breaks without retinal detachment in autopsy eyes. Acta Ophthalmol. 1972;50:147–159.
170 Foos RY. Tears of the peripheral retina: pathogenesis, incidence, and classification in autopsy eyes. Mod Probl Ophthalmol. 1975;15:68–81.
171 Byer NE. The natural history of asymptomatic retinal breaks. Ophthalmology. 1982;89:1033–1039.
172 Neumann E, Hyams S. Conservative management of retinal breaks: a follow-up study of subsequent retinal detachment. Br J Ophthalmol. 1972;56:482–486.
173 Haimann MH, Burton TC, Brown CK. Epidemiology of retinal detachment. Arch Ophthalmol. 1982;100:289–292.
174 Bohringer HR. Statistiches zu Haufigkeit and Risiko der Netz-hautablosung. Ophthalmologica. 1956;131:331–334.
175 Benson WE. Prophylactic therapy of retinal breaks. Surv Ophthalmol. 1977;22:41–47.
176 Combs JL, Welch RB. Retinal breaks without detachment: natural history, management, and long-term follow-up. Trans Am Ophthalmol Soc. 1982;80:64–97.
177 DiBernardo C, Blodi B, Byrne SF. Echographic evaluation of retinal tears in patients with spontaneous vitreous hemorrhage. Arch Ophthalmol. 1992;110:511–514.
178 Sarrafizadeh R, Hassan T, Ruby AJ. Incidence of retinal detachment and visual outcome in eyes presenting with posterior vitreous separation and dense fundus-obscuring vitreous hemorrhage. Ophthalmology.. 2001;108:2273–2278.
179 Theodossiadis GP, Velissatopoulos P, Magouritsas N, et al. Behandlung und Nachuntersuchung von Netzhautrissen ohne Netz-hautablosung mit den Riss uberlagerndem abgehobenem Netzhautgefass. Klin Monatsbl Augenheilkd. 1977;170:411–415.
180 Lincoff H, Kreissig I, Richard G. Treating avulsed vessels with a temporary balloon buckle. Am J Ophthalmol. 1986;101:90–94.
181 Krebs I, Brannath W, Glittenberg K, et al. Posterior vitreomacular adhesion: a potential risk factor for exudative age-related macular degeneration. Am J Ophthalmol. 2007;144:741–746.
182 Robison C, Krebs I, Binder S, et al. Vitreomacular adhesion in active and end-stage age-related macular degeneration. Am J Ophthalmol. 2009;148:79–82.
183 Gass JDM, Norton WD. Cystoid macular edema and papilledema following cataract extraction: a fluorescein funduscopic and angiographic study. Arch Ophthalmol. 1966;76:646–661.
184 Irvine AR. Cystoid maculopathy. Surv Ophthalmol. 1976;21:1–17.
185 Jaffe NS. Vitreous traction at the posterior pole of the fundus due to alterations in the vitreous posterior. Trans Am Acad Ophthalmol Otolaryngol. 1967;71:642–652.
186 Sebag J, Balazs EA. Pathogenesis of cystoid macular edema: an anatomic consideration of vitreoretinal adhesions. Surv Ophthalmol. 1984;28(suppl):493–498.
187 Smiddy WE, Green WR, Michels RG, et al. Ultrastructural studies of vitreomacular traction syndrome. Am J Ophthalmol. 1989;107:177–185.
188 Patel A, de Bustros S, Michels RG. Pars plana vitrectomy for aphakic cystoid macular edema. Retina. 1985;5:11–15.
189 Fung WE, Vitrectomy–ACME Study Group. Vitrectomy for chronic aphakic cystoid macular edema: results of a national, collaborative, prospective, randomized investigation. Ophthalmology. 1985;92:1102–1111.
190 McDonnell PJ, Fine SL, Hillis AI. Clinical features of idiopathic macular cysts and holes. Am J Ophthalmol. 1982;93:777–786.
191 Bronstein MA, Trempe CL, Freeman HM. Fellow eyes of eyes with macular holes. Am J Ophthalmol. 1981;92:757–761.
192 Avila MP, Jalkh AE, Murakami K, et al. Biomicroscopic study of the vitreous in macular breaks. Ophthalmology. 1983;90:1277–1283.
193 Morgan CM, Schatz H. Involutional macular thinning: a premacular hole condition. Ophthalmology. 1986;93:153–161.
194 Guyer DR, Green WR. Idiopathic macular holes and precursor lesions. In: Franklin RM, ed. Symposium on vitreous and retinal disease. Proceedings of the 1992 New Orleans Academy of Ophthalmology. New York: Kugler, 1993.
195 Sadda SR, Campochiaro PA, de Juan E, Jr., et al. Histopathological features of vitreous removed at macular hole surgery. Arch Ophthalmol. 1999;117:478–484.
196 Kelly NE, Wendel RT. Vitreous surgery for idiopathic macular holes: results of a pilot study. Arch Ophthalmol. 1991;109:654–659.
197 Funata M, Wendel RT, de la Cruz Z, et al. Clinicopathologic study of bilateral macular holes treated with pars plana vitrectomy and gas tamponade. Retina. 1992;12:289–298.
198 Madreperla SA, Geiger GL, Funata M, et al. Clinicopathologic correlation of a macular hole treated by cortical vitreous peeling and gas tamponade. Ophthalmology. 1994;101:682–686.
199 Haritoglou C, Reiniger IW, Schaumberger M, et al. Five-year follow-up of macular hole surgery with peeling of the internal limiting membrane: update of a prospective study. Retina. 2006;26:618–622.
200 Johnson RN, Gass JDM. Idiopathic macular holes: observations, stages of formation, and implications for surgical intervention. Ophthalmology. 1988;95:917–924.
201 Jost BF, Hutton WL, Fuller DG, et al. Vitrectomy in eyes at risk for macular hole formation. Ophthalmology. 1990;97:843–847.
202 de Bustros S. Vitrectomy for prevention of macular holes. Results of a randomized multicenter clinical trial. Vitrectomy for Prevention of Macular Hole Study Group. Ophthalmology. 1994;101:1055–1059.
203 Cibis GW, Watzke RC, Chua J. Retinal hemorrhages in posterior vitreous detachment. Am J Ophthalmol.. 1975;80:1043–1046.
204 Kroll P, Wiegand W, Schmidt J. Vitreopapillary traction in proliferative diabetic vitreoretinopathy. Br J Ophthalmol. 1999;83:261–264.
205 Katz B, Hoyt C. Gaze-evoked amaurosis from vitreopapillary traction. Am J Ophthalmol. 2005;139:631–637.
206 Bradford RH, Wilkinson CP. Vitreous opacification after cataract extraction. Am J Ophthalmol. 1987;103:276–280.
207 McDonnell PJ, de la Cruz ZC, Green WR. Vitreous incarceration complicating cataract surgery: a light and electron microscopic study. Ophthalmology. 1986;93:247–253.
208 Osterlin S. Macromolecular composition of the vitreous in the aphakic owl monkey eye. Exp Eye Res. 1978;26:77–84.
209 Kawano SI, Honda Y, Negi A. Effects of biological stimuli on the viscosity of the vitreous. Acta Ophthalmol. 1982;60:977–991.
210 Heller MD, Straatsma BR, Foos RY. Detachment of the posterior vitreous in phakic and aphakic eyes. Mod Probl Ophthalmol. 1972;10:23–36.
211 McDonnell PJ, Patel A, Green WR. Comparison of intracapsular and extracapsular cataract surgery: histopathologic study of eyes obtained postmortem. Ophthalmology. 1985;92:1208–1223.
212 Erie JC, Raecker ME, Baratz KH, et al. Risk of retinal detachment after cataract extraction, 1980–2004: a population-based study. Trans Am Ophthalmol Soc. 2006;104:167–175.
213 Cox MS, Schepens CL, Freeman HM. Retinal detachment due to ocular contusion. Arch Ophthalmol. 1966;76:678–685.
214 Massicotte SJ, Folberg R, Torczynski E, et al. Vitreoretinal traction and perimacular retinal folds in the eyes of deliberately traumatized children. Ophthalmology. 1991;98:1124–1127.
215 de Juan E, Sternberg P, Michels RG. Penetrating ocular injuries: types of injuries and visual results. Ophthalmology. 1983;90:1318–1322.
216 Winthrop SR, Cleary PE, Minckler DS, et al. Penetrating eye injuries: a histopathological review. Br J Ophthalmol. 1980;64:809–817.
217 Faulborn J, Topping TM. Proliferations in the vitreous cavity after perforating injuries: a histopathological study. Graefes Arch Clin Exp Ophthalmol. 1978;205:157–166.
218 Cleary PE, Ryan SJ. Histology of wound, vitreous and retina in experimental posterior penetrating eye injury in the rhesus monkey. Am J Ophthalmol. 1979;88:221–231.
219 Abrams GW, Topping TM, Machemer R. Vitrectomy for injury: the effect on intraocular proliferation following perforation of the posterior segment of the rabbit eye. Arch Ophthalmol. 1979;97:743–748.
220 Cleary PE, Ryan SJ. Vitrectomy in penetrating eye injury: results of a controlled trial of vitrectomy in an experimental posterior penetrating eye injury in the rhesus monkey. Arch Ophthalmol. 1981;99:287–292.
221 Spalding SC, Sternberg P. Controversies in the management of posterior segment ocular trauma. Retina. 1990;10:76–82.
222 Gregor Z, Ryan SJ. Complete and core vitrectomies in the treatment of epiretinal posterior penetrating eye injury in the rhesus monkey. II. Histologic features. Arch Ophthalmol. 1983;101:446–450.
223 Miller B, Miller H, Patterson R, et al. Effect of the vitreous on retinal wound-healing. Graefes Arch Clin Exp Ophthalmol. 1986;224:576–579.
224 Smiddy WE, Maguire AM, Green WR, et al. Idiopathic epiretinal membranes: ultrastructural characteristics and clinicopathologic correlation. Ophthalmology. 1989;96:811–821.
225 Gupta P, Sadun AA, Sebag J. Multifocal retinal contraction in macular pucker analyzed by combined optical coherence tomography/scanning laser ophthalmoscopy. Retina. 2008;28:447–452.
226 Wilkes SR, Mansour AM, Green WR. Proliferative vitreoretinopathy: histopathology of retroretinal membranes. Retina. 1987;7:94–101.
227 The Retina Society Terminology Committee. The classification of retinal detachment with proliferative vitreoretinopathy. Ophthalmology. 1983;90:121–125.
228 Charles S. Vitreous microsurgery. Baltimore: Williams and Wilkins; 1981.
229 Kampik A, Kenyon KR, Michels RG, et al. Epiretinal and vitreous membranes: a comparative study of 56 cases. Arch Ophthalmol. 1981;99:1445–1454.
230 Wallow IHL, Stevens TS, Greaser ML, et al. Actin filaments in contracting preretinal membranes. Arch Ophthalmol. 1984;102:1370–1375.
231 Campochiaro PA, Gaskin HC, Vinores SA. Retinal cryopexy stimulates traction retinal detachment formation in the presence of an ocular wound. Arch Ophthalmol. 1987;105:1567–1570.
232 Campochiaro PA, Glaser BM. Platelet-derived growth factor is chemotactic for human retinal pigment epithelial cells. Arch Ophthalmol. 1985;103:576–579.
233 Harvey AK, Roberge F, Hjelmeland LM. Chemotaxis of rat retinal glia to growth factors found in repairing wounds. Invest Ophthalmol Vis Sci. 1987;28:1092–1099.
234 Sebag J. Shaken not stirred. Ophthalmology. 2001;108:1177–1178.
235 Sebag J. Pharmacologic vitreolysis – premise and promise of the first decade. Retina. 2009;29:871–874.