Transplantation
Learning Objectives
• List three human leukocyte antigen (HLA) class I loci
• List the three HLA class II loci
• Compare and contrast the two antibody tests that are used to determine tissue compatibility
• Compare and contrast the three tests used to identify HLA antigens on the donor and recipient cells
• Define autograft, isograft, allograft, and xenograft
• Discuss the major impediments to successful use of xenografts
• Discuss the advantages of transgenic pigs as a source of organs for transplantation
• Compare and contrast the immunologic mechanisms active in hyperacute rejection and acute rejection
• Discuss the immunologic mechanisms active in chronic rejection
• Identify the role of minor HLA molecules in long-term transplant survival
• List the five families of drugs that are used to prevent graft rejection
• Discuss the mechanism of action (MOA) of corticosteroids
• Recognize the MOA of calcineurin inhibitors used to prevent graft rejection
• Identify the MOA of lymphocyte proliferation inhibitors used to prevent graft rejection
• List the monoclonal antibodies use to prevent graft prevention
• Identify the role of polyclonal antibodies in graft prevention
• Recognize surface molecules that are unique to hematopoietic stem cells
• Identify the three sources of hematopoietic stem cells
• Explain the advantages and disadvantages of using the three sources of stem cells
• Define graft-versus-host disease (GVHD)
• List the target organs involved in acute GVHD reactions
• Explain the immunologic mechanisms active in acute GVHD
• Identify the drugs used to treat acute GVHD
Key Terms
Acute rejection
Allogeneic graft
Antibody cross-matching
Autograft
Chronic rejection
Graft-versus-host disease
Hyperacute rejection
Induction immunosuppression
Isograft
Maintenance immunosuppression
Polymerase chain reaction
Rescue immunosuppression
Sequence-based oligonucleotides
Sequence-based typing
Sequence-specific primers
Xenograft
Introduction
The transplantation era began in 1944 when Peter Medawar demonstrated that human skin grafts between unrelated donors were rejected, but the rejection rate decreased when a graft was obtained from a related donor. He suggested that the immune system recognized the skin from unrelated donors as foreign and mounted a vigorous immune response. In 1958, Jean Dausset described the first human leukocyte-specific antigen (HLA-A2) and demonstrated that these antigens were important in the recognition of foreign tissue. Between 1962 and 1980, immunologists and geneticists identified different HLA loci and determined that HLAs on donated organs were the targets of an immune response to transplanted tissue or organs. Matching donor and recipient HLAs prior to transplantation greatly increased graft survival. Introduction of anti-rejection drugs such as azathioprine, cyclosporine, and tacrolimus has significantly lessened the risk of organ rejection over time.
Between 28,000 and 36,000 organ transplantations are performed in the United States yearly. The most commonly transplanted organs are kidney, heart, and liver. But other organs, including cornea, lung, liver, pancreas, intestine, bone, skin, and bone marrow, can be transplanted. Organ transplantation has been hampered by a scarcity of available organs and bone marrow donors. Currently, over 85,000 individuals in the United States are awaiting organ transplantation.
Types of Transplantations
The four types of transplantations are as follows: (1)When the same individual serves as the donor and the recipient of the transplanted tissue, the graft is termed an autologous graft, or an autograft. (2) Tissue transplanted between genetically identical twins is called an isograft, or a syngeneic transplant. (3) A graft between unrelated or mismatched individuals is an allogeneic transplant. (4) When organs are transplanted across species barriers from animals to humans, the grafts are termed xenografts (Figure 17-1).
Xenografts
Xenografts merit more discussion because they solve the problem of organ shortages and long waiting times for transplants. Pigs are useful for transplantation purposes because of the similarity of organ size, weight, and physiology between pigs and humans. However, xenotransplantation has proved impractical because humans have pre-existing immunoglobulin M (IgM) antibodies directed at porcine carbohydrate antigens containing α-galactosyl residues that are expressed on porcine cells. When porcine organs are transplanted into humans, hyperacute rejection occurs within hours.
To prevent xenograft rejection, recombinant deoxyribonucleic acid (DNA) technology has been used to create transgenic pigs that can be used as a source of tissue and organs. Cells from these pigs do not express the carbohydrate antigens that elicit the IgM response. Moreover, cells express molecules that downregulate natural killer (NK) cells (ecto-5´-nucleotidase) or prevent complement activation (CD55 and CD59).
Before xenotransplantation can be implemented in clinical practice, questions concerning the risk of microbial and viral transmission from pigs to humans must be addressed and resolved. Although pigs and humans have been in close contact for centuries and xenografts are unlikely to harbor new or novel infectious agents, little is known about exogenous and endogenous retroviruses in pigs. In vitro, endogenous porcine retroviruses can infect human cell lines.
Human Leukocyte Antigen Markers and Transplantation
Major histocompatibility complex (MHC) antigens in animals and HLAs are considered “self-molecules” and determine acceptance or rejection of transplanted tissue (see Chapter 4). The three class I loci (HLA-A, HLA-B, and HLA-C) and the three class II loci (HLA-DR, HLA-DQ, and HLA-DP) molecules in humans are important in transplantation.
Each individual has two complete sets of the six HLA molecules. One set is inherited from the father and another set from the mother. Each set of HLA molecules is called a haplotype. Since extensive polymorphism and multiple alleles exist in each locus, individuals may have homozygous or heterozygous alleles at each locus.
Test for Tissue Compatibility in Syngeneic and Allogeneic Grafts
Antibody Cross-Matching
As a consequence of blood or platelet transfusion, a previous organ transplantation, or multiple pregnancies, many individuals have antibodies directed at major (classes I and II) or minor (class III) HLA antigens. For example, approximately 20% of patients on the kidney transplantation list have high levels of anti-HLA antibodies in serum. The presence of pre-existing antibodies limits the population of potential donors and extends the waiting time for a kidney. Individuals with no pre-existing HLA antibodies usually receive a kidney within 500 days from the date their name appears on the transplantation registry. If high levels of pre-existing antibodies are present, the waiting time is 2300 days.
An initial test determines the presence of anti-HLA–specific antibodies in the recipient’s serum. In a lymphocytotoxicity assay, serum from the recipient is made to react with ethidium bromide–stained T and B cells from the donor. In the presence of antibodies and complement, dead cells stain red, and live cells stain green. Cytotoxicity is reported as a percentage of the donor’s cells killed in the assay. This means that the higher the concentration of cells that register as dead, the higher the antibody concentration. The presence of preformed antibodies to the HLA molecules on the donor tissue precludes the possibility of successful transplantation.
The recipient’s serum is also tested for non-HLA–specific antibodies directed at the donor’s endothelial cells. Endothelial cells are the initial contact point between the graft and host tissue, and rejection begins at this interface. The presence of anti–endothelial cell antibodies increases the risk of antibody-mediated organ rejection.
Tissue Cross-Matching
Several DNA-based molecular diagnostic techniques are used to type and cross-match tissue for transplantation. Polymerase chain reactions (PCRs) are used to amplify stretches of genomic DNA encoding HLA molecules. Allelic polymorphism within each locus is identified through hybridization with sequence-specific primers (SSP) or sequence-specific oligonucleotides (SSO) or sequence-based typing (SBT). The choice of assays depends on the clinician’s requirements with regard to resolution, sensitivity, and speed.
The PCR-SSO is a low-cost, high-volume assay that can screen a large number of potential donors with low or intermediate HLA resolution. Each probe is complementary to different motifs within an allelic hypervariable region of HLA molecules. Thus, the technique can identify heterozygous or homozygous combinations.
PCR-SSP has intermediate resolution for HLA-A, HLA-B, HLA-C, DR, and DQ and can be performed rapidly usually within 3 to 4 hours. It is especially useful in typing cadaver organs that are used for transplantation or identifying closely related HLA alleles.
PCR-SBT has the highest resolution for determining allelic polymorphisms. In the assay, the coding region of the entire HLA sequence on chromosome 6 is amplified by PCR. Coding sequences in exons 2 and 3 (HLA-A, HLA-B, and HLA-C) and exon 2 (DR, DQ, and DP) are then sequenced to determine HLA alleles. Employing different formats, SBT can be used to determine heterozygous sequences at each locus, haploid sequences, or a combination of heterozygous and homozygous sequences.
Antigen Presentation in Allogeneic Grafts
T cells are stimulated and activated either directly or indirectly. In direct antigen presentation, a recipient’s naïve T cells recognize donor antigen-presenting cells (APCs) and endothelial cells expressing non-HLA self-peptides. The role of the self-peptide in T cell activation is unclear. Self-peptides may act to stabilize the allogeneic HLA molecule or directly contribute to T cell recognition (Figures 17-2 and 17-3).
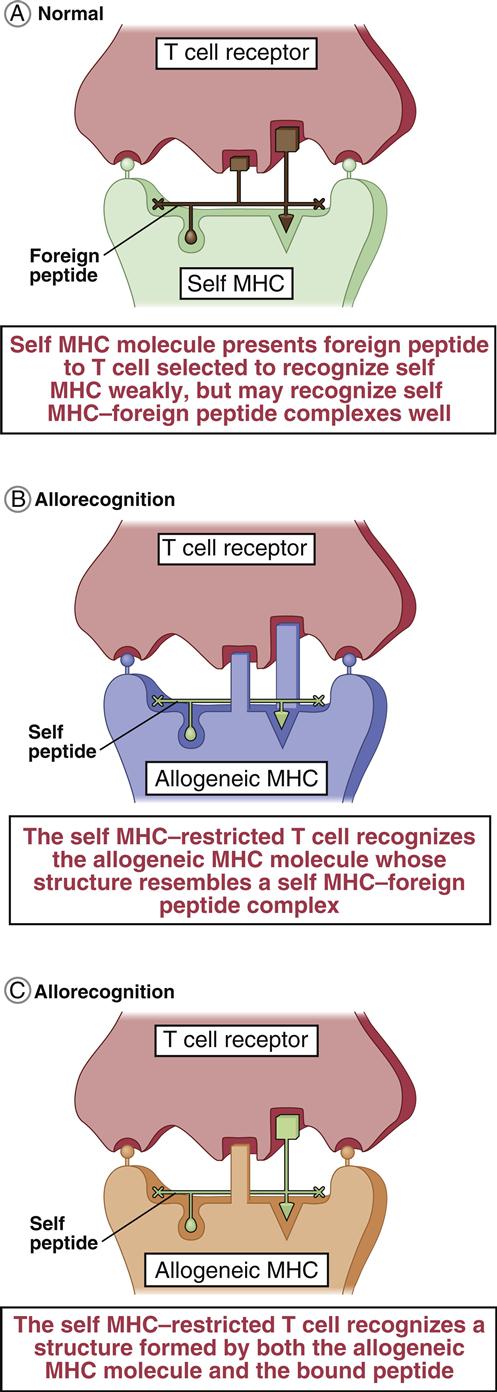