Antibodies and In Vivo Therapy
Learning Objectives
• Discuss the problems associated with the use of animal antisera
• Explain the immunologic mechanisms involved in the evolution of serum sickness
• Recognize the constituents of fractionated immunoglobulin (Ig)
• Identify the conditions and diseases treated with intravenous immunoglobulin (IVIG)
• Identify the signs and symptoms of idiopathic thrombocytopenic purpura (ITP)
• Recognize the immunologic mechanism involved in ITP
• Explain the role of IVIG in inhibiting the progression of ITP
• Discuss the pathophysiology of Kawasaki disease
• Explain the relationship between IVIG and complement in Kawasaki disease
• Recall the role of pooled Ig in treatment of Rh blood group incompatibility
• Identify the role of IVIG in the downregulation of T cell–mediated autoimmune diseases
• Compare and contrast the advantages and limitations of pooled human Ig, and monoclonal antibodies
• Compare and contrast DNA de novo synthesis and the nucleic acid salvage pathways
• Explain the biologic basis for HAT (hypoxanthine-aminopterin-thymidine) selection
• Compare and contrast murine, chimeric, humanized, and human monoclonal antibodies
• Recall the guidelines used in the generic names for monoclonal antibodies used in immunotherapy
• Define bi-specific antibodies
• Recognize the characteristics of bi-functional and flex antibodies
• Define single-chain variable fragments (scFv)
• Restate the three critical issues in the development of radiolabeled antibodies
• Identify two antibodies used for the treatment of non-Hodgkin’s lymphoma
Key Terms
Active immunity
Chimeric antibodies
Classic nucleic acid pathway
Erythroblastosis fetalis
HAT selection
Hybridoma
Humanized monoclonal antibodies
Idiopathic thrombocytopenic purpura
Kawasaki disease
Monoclonal antibody
Nucleic acid salvage pathway
Passive immunity
Serum sickness
Single-chain variable fragment (scFv) protein
Introduction
Immunity can be generated by active or passive means. Active immunity results from exposure to microbes such as Streptococcus and Pneumococcus or vaccination with dead or weakened microbes. In contrast, the administration of antibodies to correct an immunodeficiency or provide short-term immunity against microbial infections is called passive immunity. Transfer of antibodies in colostrum from mother to child or administration of pooled immunoglobulins are examples of natural and artificial passive immunity.
Heterologous Antisera to Provide Passive Immunity
The administration of antibodies to provide passive immunity is not a new concept. In the 1890s, Emil von Behring introduced the concept of passive immunity for the treatment of diseases. He demonstrated that equine polyvalent antiserum was efficacious in the treatment of diphtheria infections in children. In the early 1900s, equine antiserum therapy was used to treat streptococcal pneumonia, bacterial meningitis, and Haemophilus infections. By 1950, antibody therapy had been supplanted by antibiotics, which were cheaper to produce. However, equine antisera are still used for the neutralization of anthrax, tetanus, gas gangrene and diphtheria exotoxins; snake venoms; and the rabies virus. Immunogenicity is a major problem associated with heterologous antisera. Anaphylaxis and serum sickness have been frequently encountered in their use.
Serum Sickness
Serum sickness is caused by the administration of large amounts of any foreign protein. Within 7 to 10 days after administration, an immunoglobulin M (IgM) antibody response to the foreign protein develops. When equal molar concentrations of antigens and antibodies are generated, large complexes settle out in the internal elastic lamina of arteries and perivascular regions. Activation of the complement cascade and the release of cytotoxic factors from endothelial cells cause the necrosis of the capillary vessels in the skin and kidneys.
Patients develop clinical manifestations of fever, arthralgia, lymphadenopathy, and skin eruptions. Serious side effects also have been reported as a consequence of antibody therapy. High concentrations of infused antibody (0.5 to 2.0 mg/kg) can cause renal failure, aseptic meningitis, and thrombosis.
Pooled Human Immunoglobulin
To reduce the morbidity associated with heterologous antisera, fractionated human immunoglobulin is now used as a therapeutic agent. Pooled human immunoglobulin is prepared from a panel of 3000 to 10,000 donors and contains 95% intact, unmodified IgG with trace amounts of IgA and IgM. Preparations also contain the macrophage–granulocyte stimulating factor, interleukin 1 (IL-1), and complement regulators.
Initially, pooled human immunoglobulin was administered by the intramuscular (IM) route. Although effective in reducing the frequency of infections, it was difficult to maintain optimal antibody levels in blood. In 1981, intravenous preparations of human immunoglobulin (IVIG) became available. IVIG allows the infusion of large volumes of antibodies while reducing the cost and the pain associated with intramuscular administration.
IVIG has been used to treat immunodeficiencies, human immunodeficiency virus (HIV) infection in infants, lymphoproliferative malignancies, and infections in low-birth-weight infants. It is also used to treat a wide range of autoimmune diseases such as myasthenia gravis, systemic lupus erythematosus, and autoimmune neuropathies (Box 13-1).
IVIG is particularly useful in the treatment of diseases such as idiopathic thrombocytopenic purpura (ITP) and Kawasaki disease and for the prevention of erythroblastosis fetalis.
Idiopathic Thrombocytopenic Purpura
ITP is a pediatric disease that is associated with low platelet counts. Children often present with bruises all over the body, bleeding gums, and, occasionally, a bleeding nose. Patients with persistently low platelet counts are also at high risk of intracranial hemorrhage.
ITP is an autoimmune disease characterized by antibody-coated platelets. In most cases, coated platelets bind to Fc receptors on macrophages in the spleen and liver. Following phagocytosis, platelets are destroyed by the oxidative killing mechanism. In a small proportion of patients, the liver fails to remove the platelets, and circulating antibody–platelet immune complexes are created. Complexes localize in arterioles and capillaries, occluding vessels and causing tissue ischemia. Vessels in the heart, kidney, brain, pancreas, and spleen are common targets for immune complex deposition.
Treatment of Idiopathic Thrombocytopenic Purpura
Administration of IVIG reduces autoantibody levels and disease symptoms. The mechanism is both simple and elegant. Pooled IVIG saturates specialized neonatal Fc receptors (FcRn) on endothelial cells. When the receptor is engaged, IgG is internalized by pinocytosis and recycled to the cell surface, thus preventing lysosomal degradation (Figure 13-1).
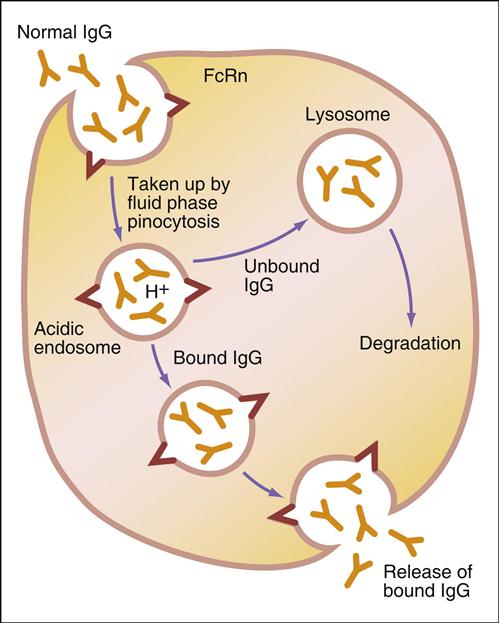
In contrast, serum autoantibodies binding to other Fc receptors on the same cells are internalized and destroyed, thereby arresting the disease process but not curing the disease.
Kawasaki Disease
Kawasaki disease is a self-limiting, febrile disease that is characterized by vasculitis of peripheral and coronary vessels. Patients present with a persistent rash, conjunctivitis, and lymphadenopathy. Individuals with Kawasaki disease have a high risk of heart failure, pericarditis, mitral or aortic valve insufficiency, and rupture of the coronary artery. In the last 5 years, Kawasaki disease has emerged as the leading cause of heart disease in the United States. Although the etiology of the disease is unclear, an immune response to a viral (parvovirus B19 or cytomegalovirus) or bacterial (Yersinia) infection is believed to activate an autoimmune response to vascular endothelial cells and cardiac myosin.
Treatment of Kawasaki Disease
Infusion of IVIG reduces the symptoms by inhibiting the formation of a C3 convertase. Microaggregates within the IVIG displace C3b from tissue-bound immune complexes to the fluid phase, which downregulates the inflammatory response. IVIG also downregulates an inflammatory response by scavenging the anaphylatoxins (C3a and C5b) via low-affinity F(ab′)2 receptors in microaggregated immunoglobulin. In children with early disease, one regimen of IVIG may reduce the symptoms and progression of the disease for several years.
Erythroblastosis Fetalis
Pooled immunoglobulin is also used to prevent erythroblastosis fetalis in neonates caused by Rh factor incompatibility between mother and child (see Chapter 3). Generally, the mother is Rh negative (Rh–) and the fetus is Rh positive (Rh+). Spillage of fetal blood into the maternal circulation elicits an immune response that destroys the infant’s blood cells. When the fetal bone marrow cannot compensate for the destruction of red blood cells, severe anemia occurs. Administration of RhoGAM (anti-Rh) is sufficient to prevent the stimulation of the maternal immune response to Rh+ red blood cells from the fetus.
Intravenous Immunoglobulin and T Cell–Mediated Autoimmune Diseases
Although Fc saturation and complement inhibition explain the IVIG immunomodulation of antibody-mediated autoimmune diseases, it does not account for the downregulation of autoimmune diseases mediated by auto-reactive T cells. In animal models of diseases mediated by T cells, infusion of immunoglobulin increases the number and function of CD4 regulatory cells or downregulates the function of T cells by inhibiting the expression of B7-1 by dendritic cells. Engagement of B7-1 and CD28 on T cells provides the second signal required for T cell activation (see Chapter 6).
Limitations of Pooled Human Immunoglobulin
Available IVIG products differ widely in immunoglobulin content, the affinity and avidity of antibodies, and IgG subclass distribution. This may reduce the efficacy of the product when used for immunomodulation. Moreover, pooled immunoglobulin is a finite reagent and new lots must be prepared every few years. Variation in efficacy between different batches of the same IVIG lot also occurs. Variations may be caused by differences in the concentrations or the sizes of microaggregates, which reduce the bioavailable antibody concentration.
Safety concerns about the use IVIG do exist. A small percentage of individuals manifest infusion-related adverse effects, including nausea, headache, backache, and muscle pain. Individuals with high levels of anti-IgA antibodies have a high risk of life-threatening anaphylaxis.
Disease transmission is always a concern when blood products are used for therapy. Transmission of HIV, hepatitis B, or Creutzfeldt-Jakob syndrome prions has not been reported. However, several cases of IVIG-transmitted hepatitis C were reported before 1994. Additional antiviral measures included in the manufacturing process have reduced the possibility of hepatitis C transmission.
Monoclonal Antibodies Produced in Tissue Culture
Monoclonal antibodies are a product of recombinant DNA technology and can be produced in the laboratory. The name is derived from the fact that antibodies are produced from a population of cells that originated from a single B cell clone (monoclonal). Monoclonal antibodies have the same isotype and affinity constants for an antigen. In essence, monoclonal antibodies are a homogeneous antibody population that can be produced in the laboratory for decades.
Köhler and Milstein created the tissue culture–based system that produces homogeneous antibodies that bind to only one antigen with a high affinity. The methodology is based on the fusion of antigen-stimulated murine splenic B cells with a tissue culture–adapted, antibody-producing murine myeloma cell line. The spleen cells carry the information for antibody specificity, while the myeloma cells act as factories for the production of a specific immunoglobulin isotype. The fusion of the two types of cells creates a hybrid cell that can produce homogeneous antibodies directed at a specific antigen.
In the experimental protocol, polyethylene glycol (PEG) is used to fuse cell membranes. At best, the fusion is nonspecific and creates different products that include B cell–B cell fusions; B cell–myeloma cell fusions; and myeloma cell–myeloma cell fusions. The next step selects the B cell–myeloma cell fusions for expansion and study. Selection is necessary to prevent overgrowth by tissue culture–adapted myeloma cells and myeloma cell–myeloma cell fusion products.
HAT (Hypoxanthine–Aminopterin–Thymidine) Selection
Selection takes advantage of different nucleic acid synthesis pathways. The classic de novo pathway is a complex pathway that produces folic acid. In turn, folic acid serves as a single carbon methyl donor in the synthesis of nucleotides. The nucleic acid salvage pathway provides a second conduit for DNA synthesis. In this pathway, thymidine and hypoxanthine are recycled into new nucleotides. Recycling requires the presence of an enzyme called hypoxanthine-guanine phosphorylribosyl transferase (HPRT+).
Selection of B cell–myeloma cell hybrids is facilitated by the use of medium that contains hypoxanthine–aminopterin, and thymidine (HAT). Murine B cells have both classic and salvage pathways. As a consequence of several mutational events in the myeloma cells, the HPRT gene is inactivated, and they can only synthesize DNA by the classic pathway. In the HAT selection process, aminopterin (a folic acid inhibitor) blocks the production of DNA by the classic de novo pathway and kills the myeloma cells.
Since B cell–myeloma cell hybrids have both classic and de novo synthesis pathways, they survive the selection process. The surviving cells are known as hybridomas.
Hybridomas are placed in 96-well microtiter plates and are allowed to grow for 1 to 3 weeks. Each well is tested for the presence of antibodies. Cultures producing antibodies are cloned in agar to isolate single-cell hybridomas. Each hybridoma is expanded from the single B cell clone and retested for antibody production. The original protocol for the production of monoclonal antibodies is shown in (Figure 13-2).
Production of murine monoclonal antibodies solved the problem of limited reagents, but the immunogenicity problem remained unsolved. When administered to humans, murine monoclonal antibodies are considered foreign and generate an antibody response that neutralizes the monoclonal antibodies before they can reach their target. In addition, a risk of developing serum sickness exists.
Monoclonal Antibodies with Reduced Immunogenicity
The immunogenicity of murine monoclonal antibodies has been reduced by additional genetic engineering. For example, chimeric antibodies are composed of murine VH and VL domains and constant domains from human IgG. These monoclonal antibodies contain only 33% murine protein. Humanized monoclonal antibodies contain only 5% murine or rat protein, which is located in the hypervariable regions or the complementarity-determining regions (CDRs) of heavy and light chains (Figure 13-3).
CDRs are short amino acid sequences found in the antibody hypervariable region that actually come in contact with antigens. Despite the reduction in murine proteins, monoclonal antibodies often elicit an immune response. The immunogenicity problem was solved by the introduction of human monoclonal antibodies.
Chimeric Antibodies
To produce chimeric antibodies, murine hybridomas are produced by the classic method described above. The genes coding for the murine variable region of heavy and light chains are isolated from the hybridoma and amplified by polymerase chain reaction (PCR). Constant-region genes of human heavy and light chains also are amplified. After insertion of human and murine genes into a plasmid and transfection into bacteria, chimeric antibodies are produced as inclusion bodies. Following lysis of the cells, the chimeric antibodies are purified for in vivo use. Antibody aggregation and limited growth of antibody-producing bacteria restrict the usefulness of bacterial expression systems.
Humanized Antibodies
Humanized monoclonal antibodies are derived from murine hybridomas. However, genes coding for the six murine CDRs (three each on heavy and light chains) are amplified by PCR and inserted into a plasmid vector. Genes for human variable and constant regions also are inserted into the same vector. Transfected bacteria or transformed human B cells produce humanized monoclonal antibodies.
Human Monoclonal Antibodies
It has now become possible to produce human monoclonal antibodies in transgenic mice. In these animals, the murine immune system has been inactivated and replaced by megabase-sized yeast artificial chromosome (YAC) carrying the genes for IgG1–IgG4. Repeated stimulation of the mice produces a robust response of antibodies with high affinity for antigens. B cells isolated from the draining lymph nodes or spleen are fused with myeloma cells. Following HAT selection and hybridoma expansion in 96-well microtiter plates, supernatant fluids are tested for antibody production. These laboratory-created human monoclonal antibodies have high affinity for antigens and have elimination kinetics that are identical to natural antibodies.
Nomenclature for Monoclonal Antibodies
Generic names of monoclonal antibodies provide information about the nature of the antibody, the animal source, and the antibody target. The World Health Organization Committee on Nonproprietary Names and the Adopted Name Council have published guidelines for monoclonal antibody nomenclature (Table 13-1 and Table 13-2).
Table 13-1
Nomenclature for Designating Monoclonal Antibody Source
Source | Name |
Human | u |
Mouse | o |
Rat | a |
Hamster | e |
Primate | i |
Chimeric | xi |
Humanized | zu |
Rat/mouse | axo |
Modified from Smith BT: Concepts in immunology and immunotherapeutics, Bethesda, MD, 2003, American Society of Health-System Pharmacists.
Table 13-2
Nomenclature for Designating Monoclonal Antibody Target
Source | Name |
Bacterial | Bac |
Infection | Les |
Musculoskeletal | Mol |
Colonic tumor | Col |
Mammary tumor | Man |
Ovarian | Gov |
Tumor | Tu |
Toxin | Tox |
Immune system | Lim |
Cardiovascular | Cir |
Interleukin | Kin |
Melanoma | Mel |
Testicular tumor | Got |
Prostate | Pro |
Nervous system | Neur |
Fungal | Fug |
Modified from Smith BT: Concepts in immunology and immunotherapeutics, Bethesda, MD, 2003, American Society of Health-System Pharmacists.
According to these guidelines, the suffix designates the product as a monoclonal antibody (mab), and the prefixes show the monoclonal origin and the biologic target. For example, Humira, a monoclonal antibody used to treat autoimmune rheumatoid arthritis, carries the generic name adalimumab. The name explains that the agent is a monoclonal antibody (mab) of human origin (u), which targets a component of the immune system (lim). Another example is Omnitarg, or pertuzumab, which is a monoclonal antibody (mab) that is humanized (zu) and targets tumor cells (tu). Pertuzumab is highly effective in blocking HER-2 receptors expressed on breast cancer cells, thus preventing estrogen-induced cell proliferation. The choice of the prefix has no rules, but it is usually a distinct syllable compatible with the other designations. For ease of pronunciation, the final consonant of the target designation is dropped when the source designation also begins with a consonant (e.g., basiliximab). A representative list of therapeutic monoclonal antibodies is provided in Table 13-3.
Table 13-3
Selected Monoclonal Antibodies Currently Used for Immunotherapy
< ?comst?>
Generic Name | Nature of Monoclonal | Target | Indication |
Abciximab | Chimeric | GPIIb/IIIa | Anti-platelet, post-angioplasty |
Adalimumab | Human | Tumor necrosis factor (TNF) | Rheumatoid arthritis |
Alemtuzumab | Humanized | CD52 | B cell chronic lymphocytic leukemia |
Basiliximab | Chimeric | CD25 | Organ rejection |
Bevacizumab | Humanized | Vascular endothelial growth factor (VEGF) | Metastatic colon cancer |
Cetuximab | Chimeric | Epidermal growth factor (EGF) receptor | Solid tumors |
Daclizumab | Humanized | CD25 | Acute renal graft rejection |
Eculizumab | Humanized | C5 | Paroxysmal nocturnal hemoglobulinuria (PNH) |
Efalizumab | Humanized | CD11a | Severe plaque psoriasis |
Gemtuzumab ozogamicin | Humanized | CD33 | Acute myelogenous leukemia |
Ibritumomab tiuxetan | Murine | CD20 | B cell lymphoma |
Infliximab | Chimeric | TNF | Rheumatoid arthritis, Crohn’s disease |
Muromonab-CD3 | Murine | CD3 | Acute organ rejection |
Natalizumab | Humanized | Alpha 4-integrin | Relapsing multiple sclerosis |
Omalizumab | Humanized | Immunoglobulin E (IgE) | Asthma, allergies |
Palivizumab | Humanized | F-glycoprotein | Respiratory syncytial virus (RSV) |
Panitumumab | Human | EGF receptor | EGF-R–expressing metastatic colorectal cancer |
Ranibizumab | Humanized | VEGF | Macular degeneration |
Rituximab | Chimeric | CD20 | B cell leukemia |
Tositumomab | Murine | CD20 | B cell lymphoma |
Trastuzumab | Humanized | Herceptin receptor | Breast cancer, non–small cell lung cancer |
< ?comen?>< ?comst1?>
< ?comst1?>
< ?comen1?>
Novel Antibody Configurations
Fab Fragments
Using molecular biology techniques, it is possible to construct monoclonal antibody fragments that consist solely of Fab or F(ab′)2Abciximab, a chimeric Fab fragment, is commonly used to inhibit platelet clumping after angioplasty. Afelimomab, which is undergoing clinical trials for the treatment of sepsis or septic shock, is composed of F(ab′)2 antibody fragments.
Bi-Specific Antibodies
Bi-specific antibodies can be generated by genetic engineering and the fusion of two antibody-producing hybridomas to form a quadroma. Antibodies produced by the quadroma have a normal Y-shaped IgG configuration. Bi-specific antibodies combine heavy and light chains of one monoclonal antibody with one specificity with the heavy and light chains of another monoclonal antibody with a different specificity. Therefore, a single antibody can react with two different antigens because bi-specific antibodies have two different sets of CDRs.
Bi-Functional Antibodies
Antibodies or antibody fragments can be constructed in a number of different formats by using recombinant DNA technology. Tetravalent antibodies are the most common format used in cancer immunotherapy. Two antibodies react with target molecules on the cancer cell surface, while the other antibodies react with cytotoxic T cells or natural killer (NK) cells. Antibody-mediated cross-linkage of cytotoxic cell receptors activates cytotoxic T and NK cells that lyse tumor cells.
Flex antibodies are a unique subset of tetravalent antibodies that have linker segments between the antigen-combining sites. Because of their increased flexibility, they can react with antigens that are distant from one another on the same cell.
Single-Chain Fab Variable Fragments and Phage Display
Genetic information coding for antibody-variable regions can be gleaned from naïve B cells and used to construct genetic libraries. Library construction uses common molecular biology techniques such as reverse transcription of messenger ribonucleic acid (mRNA) to create complementary DNA (cDNA) and PCR, which amplify the variable regions in heavy and light chains. These antibody-variable regions (antigen-combining sites) called single-chain variable fragments (scFv) can be expressed in the protein coat of bacterial viruses, or bacteriophages (Figure 13-4).
Libraries from tonsillar B cells have created 107 to 1010 different antibody fragments with micromolar-binding affinities.
ScFv are generated by a multiple-step protocol. In the first step, mRNA coding for VH and VL are isolated from naïve B cells, hybridomas, or B cells from persons immunized against a specific pathogen. Using reverse transcription, cDNA is created for the VH and VL chains. To link variable heavy and light chain regions, nucleotides coding for repeating units of glycine and serine (Gly4Ser)3 are added to the DNA.
ScFv are expressed in a nonlytic M13 filamentous phage. DNA coding for the scFv are inserted into phage DNA at a site coding for the phage protein coat. Escherichia coli, a gram-negative bacterium, is a receptive host for phage replication. The secreted M13 phage displays scFv in the phage coat. Soluble phage is recovered and tested for antigen binding. Only a small number of expressed scFV may react with an antigen of interest because expression is a reflection of the amino acids in the variable region. Phage expressing scFv and high antigen-binding affinity for the antigen of interest is eluted and used to infect new E. coli. Using the M13 phage display expression system, large amounts of antigen-specific scFv (20–400 mg/L) can be produced by E. coli within a short period.
Many advantages as well as disadvantages to the use of scFv exist. One of the advantages is the excellent pharmacokinetics for tissue penetration. The disadvantages are that scFv are monovalent and have a high off-rate and poor target retention time. To increase retention time, multimeric scFv have been genetically engineered. Reductions in the glycine and serine (Gly4Ser)3 linker create scFv which are bivalent (diabodies), trivalent (triabodies), or tetravalent (tetrabodies).
ScFv are now being introduced into clinical trials. Pexelizumab, a humanized scFv directed at C5, has been shown to decrease tissue damage following an acute myocardial infarction and coronary artery bypass graft (CABG) surgery.
Radioimmunotherapy
Radioisotopes with moderately high energy can be linked to monoclonal antibodies. In reagent design, the overriding issues are affinity of the antibody for the target, isotope energy level, and half-life. Radioisotopes are coupled directly with the antibody via a tyrosine residue or bound directly to the antibody Fc by a chelator. Only five radioisotopes are used in radioimmunotherapy. These isotopes include (1) rhenium 186, (2) yttrium-90, (3) indium-111, (4) iodine-131, and (5) technetium-99. All have a half-life between 6 hours and 8 days and energy levels between 100 Kev and 375 Kev.
Two radiolabeled antibodies have been used extensively in treating patients with relapsed, refractory low-grade, and transformed non-Hodgkin’s lymphoma (NHL). 131-I tositumomab and 90-Y ibritumomab are murine monoclonal antibodies directed at CD20 expressed on 95% of B cell lymphomas.
Inherent toxicities and risks are associated with the use of these radiolabeled antibodies, which include prolonged myelosuppression, myelodysplastic syndrome, and acute myelogenous leukemia. Toxicity is reduced when the anti-CD20 is combined with fludarabine or with fludarabine and cyclophosphamide.
A preloading delivery system, which reduces toxicity, also is used to deliver radioactivity to tumor cells. The protocol takes advantage of the high-binding affinities between biotin (vitamin H or B7) and an egg protein called avidin. In the laboratory, biotin is coupled with a tumor-specific antibody. After the biotinylated antibodies are administered and bind to tumor cells, free avidin is administered in low concentrations. Avidin binds with high affinity to both bound and unbound biotinylated antibodies without saturating all of the biotin receptor sites on the antibodies. Non–tumor-cell-bound, soluble antibodies with biotin-avidin localize in the liver and are ingested by Kupper cells. After clearance of non-bound antibodies, radiolabeled avidin is added, and it binds almost irreversibly to the biotinylated tumor cell–bound antibodies. Radiolabeled antibodies are internalized, and the tumor cell is destroyed.
Summary