The Immune System
After reading this chapter, the student will be able to:
• Describe the chemical composition and function of antigens and antibodies
• Name and explain the functions of the different classes of antibodies
• Describe the components and functions of the lymphatic and immune systems
• Discuss the lines of defense against microbes
• Describe the processes and steps of phagocytosis and inflammation
• Differentiate between innate (nonspecific) and acquired (specific) immunity
• Discuss active and passive immunity—both naturally and artificially acquired
• Identify and discuss autoimmune diseases
• Describe the four types of hypersensitivity reactions
• Explain how the aging process influences the immune system
Fundamentals of the Immune System
Immunology
Immunology is the study of the genetic, biological, chemical, and physical characteristics of the immune system. It describes the actions of cells, tissues, and organs that are specialized to protect the body from microorganisms and other foreign substances (Figure 20.1). In essence, immunology is concerned with how the body uses protective mechanisms to recognize self from nonself-molecules. Self and nonself are determined by specific receptors on the surface of cell membranes that recognize and differentiate among antigens. These receptors, specific to a cell or particle, are called self-antigens or cell surface markers. In response to foreign antigens, cells of the immune system produce specific antibodies that bind to specific antigens. An antigen–antibody complex is formed, which is then destroyed by the immune system. Another immune reaction to protect the body is cell-mediated immunity. This is an immune response that does not include antibodies but involves the activation of macrophages, natural killer (NK) cells, and cytotoxic T lymphocytes, and the release of various cytokines.
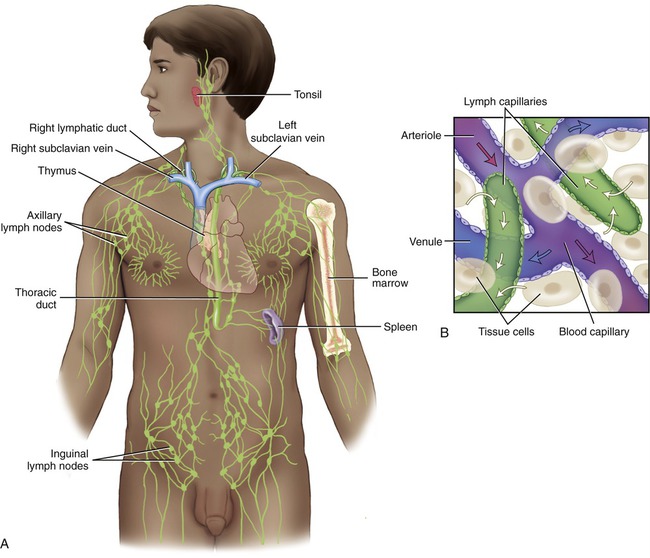
The lymphatic system consists of lymphatic vessels, lymphoid tissue and cells, and lymphatic organs.
Antigens
An antigen is any agent that is capable of binding specifically to components of the immune system such as lymphocytes, macrophages, and antibodies. Usually, only a part of an antigen triggers the immune response. The smallest part of an antigen molecule that can bind with an antibody is called an epitope or antigenic determinant. All antigens have one or more epitopes (Figure 20.2). Proteins, carbohydrates, lipids, and nucleic acids can all be antigens under certain circumstances.
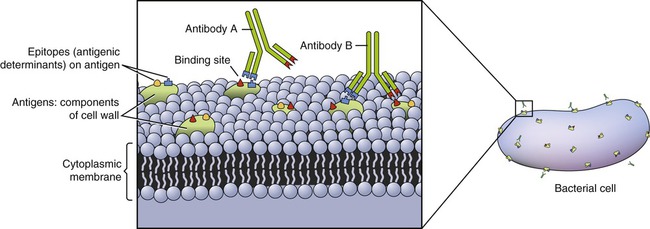
Antigens are a component of cell walls and/or plasma membranes and have one or more epitopes.
• Proteins are the most immunogenic molecules and often have several epitopes for antibody recognition.
• Carbohydrates are potentially immunogenic when bound to a protein, forming complex glycoprotein molecules. These are located in the cell membrane and can cause an immune response. Examples of the antigenicity of carbohydrates are the polysaccharides on the surface of red blood cells (see ABO blood typing discussed in the Antigens section, later in this chapter).
• Lipids also can cause an immune response if conjugated with a protein carrier.
• Nucleic acids are poor immunogens themselves, but when bound to a carrier protein they can become immunogenic. A clinical example is the presence of anti-DNA antibodies in patients with systemic lupus erythematosus (see the section Autoimmune Diseases later in this chapter).
• Immunogens are substances that stimulate the production of specific antibodies by the immune system. All immunogens are antigens but not all antigens are necessarily immunogens. Pathogenic organisms such as bacteria and viruses are immunogens.
• Tolerogens, like self-antigens, are tolerated by the immune system. However, if the molecular weight or form of a tolerogen changes, it can become an immunogen. For example, low molecular weight compounds, including some antibiotics and other drugs, are incapable of eliciting an immune response themselves, but become immunogens when conjugated with a high molecular weight molecule. The low molecular weight compound is called a hapten (Figure 20.3) and the high molecular weight compound is referred to as a carrier.
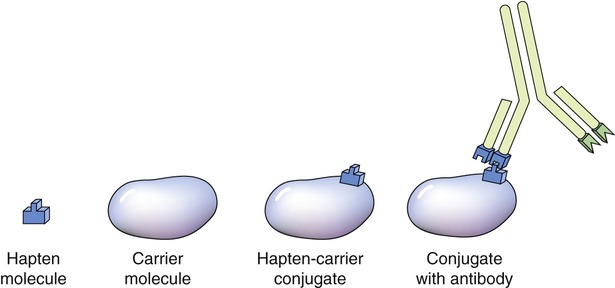
Haptens are small molecules and cannot stimulate the immune system. When a hapten combines with a carrier molecule it can result in an immune response and antibodies will bind to the hapten–carrier conjugate.
• Allergens are substances causing allergic reactions. They can be ingested, inhaled, injected, or come into contact with the skin to evoke an allergic response. Substances such as pollen, egg white, honey, and many others can cause such reactions in some individuals and therefore are referred to as allergens.
• Autoantigens are molecules, usually proteins, that are interpreted by the immune system as nonself-antigens, such as in the case of autoimmune diseases. Autoantigens are frequently components of multimolecular, subcellular particles that are involved in a variety of cell functions. Under normal circumstances these antigens would be accepted (tolerated) as self-antigens and not targeted by the immune system.
• Tumor antigens are always presented by tumor cells and never by normal cells of the body. In this case the antigens are tumor specific and typically result from tumor-specific mutations. Lymphocytes that recognize these antigens as nonself destroy these tumor cells before they can proliferate and metastasize. Examples of the origin of these tumor antigens include new genetic information introduced by a virus such as the human papillomavirus of cervical cancer, or the alteration of oncogenes by carcinogens.
• Autografts are tissues transplanted from one site in an individual to another site. An example is the removal of a portion of a blood vessel from one site and transplanted to another, in order to provide a healthy blood vessel for a dialysis stent.
• Isografts are tissues or organs transplanted between genetically identical entities such as identical twins, for whom tissue or organ rejection is not an issue. For example, a kidney or part of the liver can be transplanted from one identical twin to the other without resulting in major transplant rejection.
• Allografts are transplants between genetically different individuals from the same species (e.g., humans) but with different genomes (genetic information). In other words, the MHC molecules are different and rejection of the transplant can occur.
• Xenografts are transplants between individuals of different species such as pigs and humans. Although xenografts are often less successful than same-species grafts, pig heart valves have been used extensively and with great success for human cardiac valve replacement.
Blood typing, for compatibility before blood transfusion, is also based on genetically determined surface antigens on red blood cells (RBCs). Although more than 20 blood group systems are known today the ABO and Rh systems are used most often. The ABO system is based on the presence of type A or B antigens on the surface of RBCs. The RBCs of blood type A have A antigens, blood type B cells have B antigens, blood type AB cells have both antigens, and blood type O cells have neither A nor B antigens. The immune system does not normally produce antibodies against its own RBC antigens, but antibodies against another blood type are present in the blood plasma (Table 20.1). Type A blood contains antibodies against type B blood (anti-B) and type B blood contains antibodies against type A blood (anti-A). Type O blood contains both anti-A and anti-B, whereas type AB blood does not contain either.
TABLE 20.1
Blood Type | Antigen | Antibody |
A | A | Anti-B |
B | B | Anti-A |
AB | A, B | None |
O | None | Anti-A, anti-B |
In addition, many people have an additional antigen, the Rh factor (or D antigen), on the surface of their RBCs. This antigen was first discovered during experiments in rhesus monkeys and is therefore called the Rh factor. A person with the D antigen is called Rh positive (Rh+) and someone without D antigen is referred to as Rh negative (Rh−). Although a person with Rh− blood does not normally contain Rh antibodies in the serum, exposure to Rh+ blood will trigger the production of Rh antibodies. An example of this is erythroblastosis fetalis, or hemolytic disease, which occurs when an Rh− mother begins to develop antibodies because she is carrying an Rh+ fetus (for more information, please refer to the Medical Highlights box).
Antibodies
Structurally, antibodies are fork- or Y-shaped molecules composed of a pair of two identical long polypeptide chains (heavy chains) and a pair of identical short (light) polypeptide chains (Figure 20.4). At the end of the Y shape, formed by the heavy and light chains, are specific antigen-binding sites that differ from antibody to antibody. Because of the high variability of this region, it is referred to as the variable (V) region of the antibody. This variable region is composed of 110 to 130 amino acids, which gives the antibody its specificity for the antigen through its antigen-binding site. The remainder of the molecule is the constant (C) region that does not vary significantly from antibody to antibody. It is the variable region of antibodies that meets the enormous variety of antigenic challenges. The tips of the Y-shaped molecule consisting of the V and C regions are referred to as the Fab (fragment, antigen-binding) region, and the stem of the molecule is called the crystallized fragment or Fc fragment and plays a role in modulating immune cell activity. The Fc region can bind to various cell receptors including Fc receptors and complement proteins. This binding mediates different physiological effects, including opsonization (the action of making bacteric and other cells easier to phagocytize by marking or “tagging” them chemically), cell lysis, and degranulation of mast cells, basophils, and eosinophils.
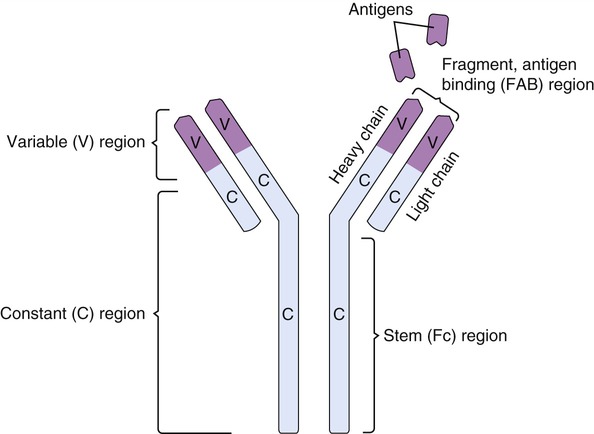
Antibodies are Y-shaped structures with two heavy chains and two light chains with specific antigen-binding sites.
Although all immunoglobulins share common features, different classes of antibodies trigger different responses when combining with the same epitope (antigenic determinant). Five classes of immunoglobulins have been identified (Table 20.2).
TABLE 20.2
Summary of Immunoglobulin Classes
Characteristic | IgG | IgD | IgE | IgA | IgM |
Structure | ![]() Monomer |
![]() Monomer |
![]() Monomer |
![]() Monomer, dimer |
![]() Pentamer |
Number of antigen-binding sites | 2 | 2 | 2 | 2 (monomer), 4 (dimer) | 10 |
Percentage of total serum antibody | 80% | 0.2% | 0.002% | 10–15% | 5–10% |
Average life span in serum | 23 d | 3 d | 2–2.5 d | 6 d | 5 d |
Function | Major antibody in circulation; long-term immunity | Receptor on B cells | Antibody in allergies and worm infections | Secretory antibody | First response to antigen; can serve as B-cell receptor |
1. IgG, a monomer produced by B cells, is the major antibody in the blood and lymphatic circulation. This class of antibody can cross the placenta and provide passive immunity to the newborn.
2. IgA, a monomer in blood and a dimer in secretions such as tears, saliva, and secretions of the respiratory and digestive tracts, provides local protection against bacteria and viruses. IgA is also found in colostrum and mother’s milk, providing additional passive immunity to the newborn.
3. IgM is a pentamer and the largest of the immunoglobulins. Because of its large molecular size, it does not diffuse readily from the bloodstream, where it remains and is efficient in reacting with bacteria and foreign cells. IgMs provide the first immunoglobulin activity in an immune response. These antibodies are also mainly responsible for the clumping of red blood cells in transfusion reactions.
4. IgD is a monomer bound to the surface of B cells and plays a role in B-cell activation. Although IgD is present in small amounts in serum its function in the circulation is unknown.
5. IgE is a monomer that binds to receptors on mast cells and basophils. It is the least abundant antibody type in serum. Functionally, it is implicated in allergic reactions and stimulates basophils to release histamines. Research indicates that IgE production can occur locally in the nasal mucosa of patients with allergic rhinitis. IgEs also provide protection against parasites and a 10- to 100-fold increase in serum IgE levels has been detected in patients with parasitic infections.
Components of the Immune System
Tissues and Organs of the Immune System
Lymphatic Vessels
Lymphatic vessels anatomically communicate with the vessels of the cardiovascular system. They originate as microscopic blind-ended vessels in the capillary beds of tissues (Figure 20.5). The function of lymph capillaries is to absorb excess extracellular (interstitial) fluid generated by the hydrostatic pressure of blood capillaries in these areas. As soon as the interstitial fluid enters the lymph capillaries it is called lymph. The plasma-like, watery lymph contains white blood cells, which are important in the immune response, and also transports dietary lipids from the small intestine to the liver.
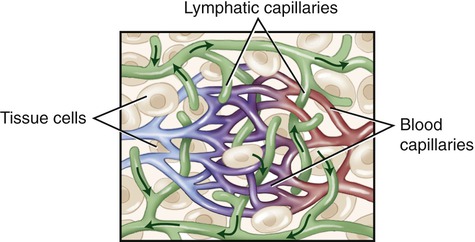
Lymph capillaries are microscopic blind-ended vessels originating in the capillary beds of tissues.
The lymph capillaries drain the lymph into larger lymphatic vessels, which resemble veins of the cardiovascular system in their structure but have thinner walls and more valves. At various intervals throughout the body’s lymph vessels, lymph passes through lymph nodes (where lymphocytes first interact with a specific antigen) and then is collected by lymphatic trunks, which carry the lymph to the largest lymphatic vessels, the lymphatic ducts. The thoracic or left lymphatic duct is the main collecting duct of the lymphatic system. It receives lymph from the left side of the head, neck, and chest, left upper limb, and the entire body inferior to the ribs. The thoracic duct then drains the lymph into the cardiovascular system via the left subclavian vein. The right lymphatic duct drains lymph from the upper right side of the body into the right subclavian vein (Figure 20.6).
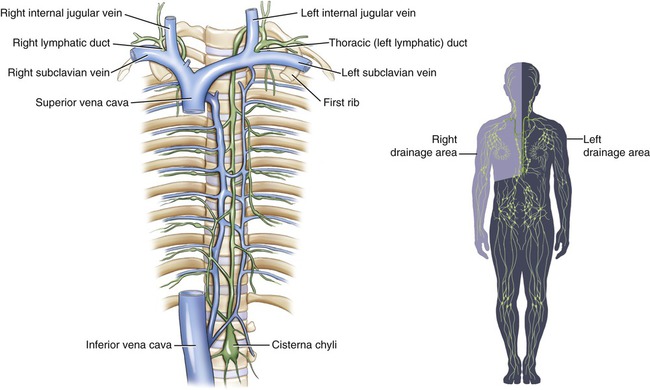
Lymph capillaries drain into larger lymphatic vessels that drain into lymphatic trunks and then into the thoracic and right lymphatic ducts. The thoracic and right lymphatic ducts deliver the lymph into the left and right subclavian veins.
Lymphoid (Lymphatic) Tissue
Lymphoid tissue consists of a framework of loose connective tissue with accumulations of lymphocytes in the interspaces. Lymphoid tissues are the secondary lymphoid organs and can be divided into two basic types: diffuse and nodular lymphatic tissue. Diffuse lymphatic tissue consists of any unorganized collections of lymphocytes, whereas nodular lymphatic tissue is much more organized (see the next section, Lymphatic Nodules). Lymphoid tissue is widely distributed in the body and is typically found at sites that provide possible routes of entry for pathogens. This type of tissue therefore plays a major role in the defense against microorganisms and is found in the connective tissues of mucous membranes in the gastrointestinal, respiratory, urinary, and reproductive tracts (Figure 20.7).
Tonsils
• A single pharyngeal tonsil, also called the adenoid, that is embedded in the posterior wall of the nasopharynx
• A pair of palatine tonsils located in the posterior region of the oral cavity
• A pair of lingual tonsils, located at the base of the tongue
HEALTHCARE APPLICATION
Tonsillitis
Symptoms | Cause | Treatment |
Red, swollen tonsils, sometimes white patches on the tonsils, severe sore throat, painful swallowing, headache, fever, enlarged tender glands, loss of voice | Viral or bacterial infection |
Lymph Nodes
Lymph nodes are small bean-shaped lymphatic organs, surrounded by a fibrous connective tissue capsule and ranging in diameter from 1 to 25 mm. The connective tissue capsule extends into the lymph node in the form of trabeculae, a meshwork of supporting reticular fibers. The parenchyma of the lymph nodes is divided into an outer cortex and an inner medulla. The cortex contains lymphoid nodules, some of which are referred to as primary nodules because they consist mainly of small lymphocytes. Secondary nodules contain a pale central region called the germinal center. A germinal center is formed when a B lymphocyte recognizes an antigen and proliferates into plasma cells for antibody production. The medulla consists of medullary cords that are separated by the medullary sinuses (Figure 20.8).
HEALTHCARE APPLICATION
Lymphoma
Term | Symptoms | Cause | Treatment |
Non-Hodgkin’s lymphoma | Enlarged lymph nodes, fever, excessive sweating with night sweats, unintentional weight loss, abdominal pain or swelling | Production of abnormal lymphocytes that continue to divide and grow uncontrollably; crowding of these lymphocytes into the lymph nodes, causing them to swell; exact cause is unknown | Chemotherapy, radiation, stem cell transplantation, observation for slow-growing lymphomas, biologic therapy, radioimmunotherapy |
Hodgkin’s lymphoma | Painless swelling of lymph nodes in neck, armpits, or groin; persistent fatigue; fever and chills; night sweats; unexplained weight loss; loss of appetite; itching | Exact cause unknown, commonly begins in the lymph nodes; development of abnormal B lymphocytes (Reed-Sternberg cells) |
Depends on disease stage; radiation, chemotherapy, bone marrow transplant |
Spleen
The spleen is the largest lymphatic organ of the body and is located in the upper left abdominal cavity between the stomach and diaphragm (Figure 20.9). The cellular components of the spleen consist of the red pulp, containing large quantities of blood, and the white pulp, which contains lymphoid tissue resembling lymphoid nodules. Approximately 25% of the body’s lymphocytes are located in the spleen. The spleen filters the blood, removing damaged blood cells and other cells with nonself-antigens. In response to circulating antigens, B cells, T cells, and macrophages are activated to eliminate any pathogens.
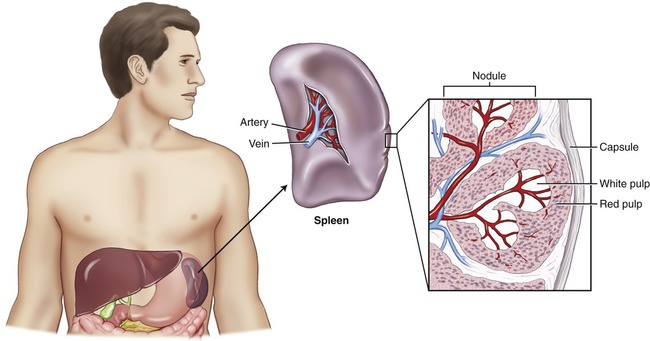
The spleen is the largest lymphatic organ, and is located in the upper left abdominal cavity. The spleen consists of red pulp, which contains a large quantity of blood, and white pulp, composed of lymphoid tissue.
HEALTHCARE APPLICATION
Spleen Disorders
Term | Symptoms | Cause | Treatment |
Trauma (damage or rupture) | Painful and tender abdomen, abdominal muscles contract and feel rigid, low blood pressure, light-headedness, blurred vision, confusion, loss of consciousness | Severe blow to the stomach, abdominal injury (e.g., car accidents), athletic mishaps | Immediate blood transfusions, followed by removal of the spleen (splenectomy), if required by the severity of tissue damage |
Splenomegaly | Abdominal pain, early satiety (fullness) due to splenic encroachment, anemia | Mononucleosis caused by the Epstein-Barr virus, other viral infections, bacterial infections, parasitic infections; diseases involving the liver, hemolytic anemias, cancer, sarcoidosis | No treatment for the disease; treatment of the underlying conditions; treatment of symptoms |
Thymus Gland
The thymus gland is a bilobate organ located in the mediastinum (Figure 20.10). The cells of the thymus produce thymosins, which are hormones essential for the maturation of the T lymphocytes. The thymus is large after birth and is essential in the development of the immune system in newborns. Although large in childhood, it gradually atrophies throughout adulthood. Functionally, after being active during the first years of life, its capacity slows down during puberty. By age 60 years the thymus gland is small and many of its cells have been replaced by adipose (fat) cells. As a result, the production of thymosin may have stopped, which negatively influences the maturation of T lymphocytes.
Red Bone Marrow (Myeloid Tissue)
Red bone marrow is found in flat and irregularly shaped bones and is considered the primary lymphoid organ. All blood cells, including the cells of the immune system, originate from hematopoietic stem cells, the hemocytoblasts located in the red bone marrow. The process of blood cell formation is called hemopoiesis or hematopoiesis and includes the following processes (Figure 20.11):
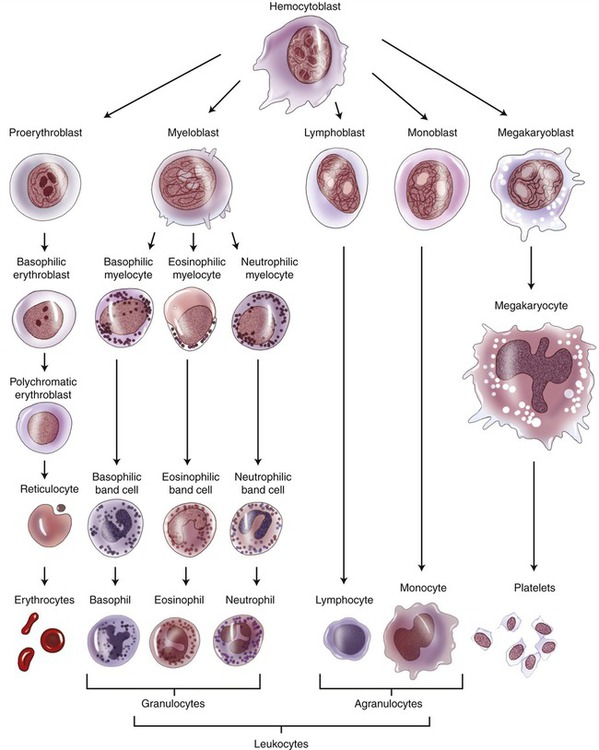
Blood cell production is called hemopoiesis and occurs in the red bone marrow. The stem cell of all blood cells is the hemocytoblast, which undergoes differentiation to form the cell lines for all blood cells.
• Erythropoiesis is the formation of erythrocytes (red blood cells) and is stimulated by the hormone erythropoietin, which is produced in the kidneys.
• Leukopoiesis is the formation of leukocytes (white blood cells) from the hematopoietic stem cells of the red bone marrow. Two pathways or processes are responsible for the production of leukocytes: lymphopoiesis and myelopoiesis.
• Lymphopoiesis is the production of lymphocytes. Immature B cells and natural killer (NK) cells are produced in red bone marrow and are transported by circulating blood to the secondary lymphoid organs, located throughout the body. Immature T cells are also produced in red bone marrow but migrate to the thymus for maturation.
• Myelopoiesis generally refers to the production of monocytes and granulocytes. This process also generates precursor cells for macrophages and dendritic cells. These cells are also transported by the circulating blood to the secondary lymphoid organs.
HEALTHCARE APPLICATION
Leukemia
Symptoms | Causes | Treatment |
Lymphocytosis; increased production of white blood cells; large atypical lymphocytes; cutaneous T-cell lymphoma; dermatitis | Genetic factors; mutagenic chemicals and viruses | Bone marrow transplants, various drug treatments, radiation |
Cells of the Immune System
Leukocytes: White Blood Cells
Leukocytes are divided into two main subdivisions: granulocytes and agranulocytes. Granulocytes are characterized by intracellular granules and on the basis of their special staining properties, differently shaped nuclei, and specific functions. They are further subdivided into basophils, eosinophils, and neutrophils (Figure 20.12). Agranulocytes are devoid of granules and are subdivided into lymphocytes and monocytes (Figure 20.13).
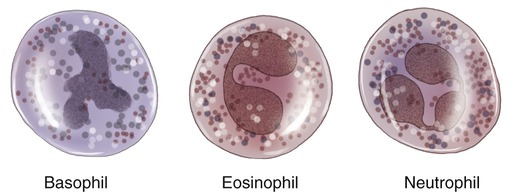
Granulocytes are identified by the specific staining properties of their granules: basophils (blue/purple granules), eosinophils (pink/reddish granules), and neutrophils, in which granules do not stain.
Agranulocytes
• Kupffer cells belong to the class of sinusoidal lining cells and are fixed macrophages located in the sinusoids of the liver (Figure 20.14, A).
• Alveolar macrophages are found in the alveoli of the lungs (Figure 20.14, B).
• Mesangial cells are macrophages located around blood vessels in the kidneys.
• Osteoclasts are macrophages in bone that break down the bone matrix when calcium is needed in the bloodstream.
• Microglial cells are the only phagocytotic cells in the brain, moving to areas where cell debris must be removed (Figure 20.14, C).
Like macrophages, dendritic cells (DCs) are antigen-presenting cells that arise from monocytes and then migrate through the blood and lymph to almost every tissue. Dendritic cells have class II MHC molecules as surface components and are characterized by long extensions that look like the dendrites of neurons, hence their name (Figure 20.15). In general, they are concentrated at sites where antigen-bearing microorganisms seek their portal of entry, such as the skin and mucous membranes. Dendritic cells check their environment for microorganisms, and when they encounter a pathogen they ingest the organism, break it down, and transport protein fragments to their cell membrane, where these cell fragments are presented to other immune system cells. The different types of DCs include thymic dendritic cells, Langerhans cells, interstitial dendritic cells, and interdigitating dendritic cells.
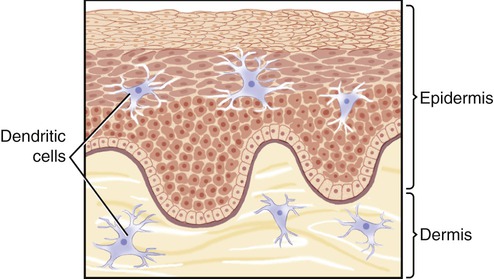
• Thymic dendritic cells produce thymosins, hormones that are believed to aid in the maturation process of T cells.
• Langerhans cells are located in the basal layer of the epidermis and engulf antigens by the process of pinocytosis. The antigens are broken down into polypeptides and moved to the cell surface. This process evokes the activation of T lymphocytes.
• Interstitial dendritic cells are part of a network in the gastrointestinal tract that monitors and interacts with the intestinal environment. These cells may induce the production of IgAs to protect the mucosal lining from pathogen attachment and invasion.
• Interdigitating dendritic cells are found in lymph nodes and play a major role in antigen presentation to the lymphocytes in these lymphatic organs.
Host Defense
Host defense mechanisms protect against foreign substances and microbes. Certain defense mechanisms are always present (innate) and often are sufficient to impede the replication and spreading of infectious agents. The function of the innate immune defense is to prevent the development of disease by mechanisms of the first and second lines of defense. Innate defense mechanisms are nonspecific because they challenge all invaders via general defense mechanisms. If the innate immune defense is insufficient to stop the invasion of the infectious agent, the adaptive immune system is activated. This process takes time to attain maximal strength. The adaptive immune system response is specific to a specific antigen and is therefore also referred to as specific defense. It represents the third line of defense (Figure 20.16).
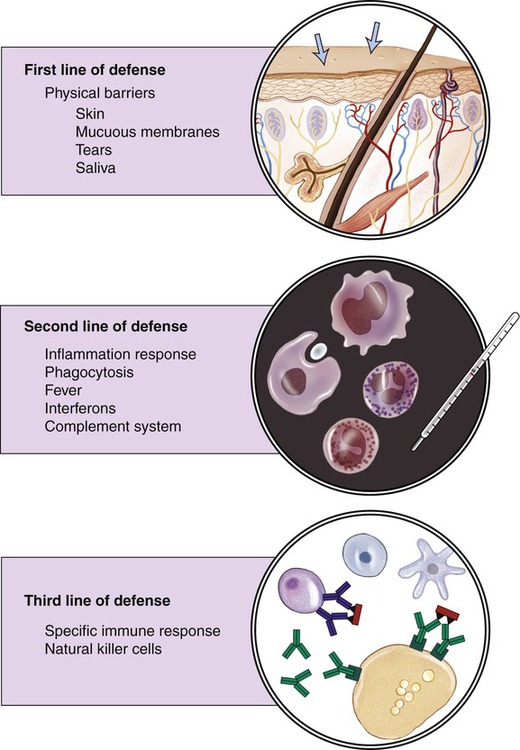
The human body has three lines of defense. The first line consists of a physical barrier (skin and mucous membranes); the second line of defense includes phagocytosis, inflammation, fever, production of interferons, and activation of the complement system; and the third line of defense is provided by specific or adaptive defense mechanisms.
First Line of Defense
Physical Barriers
The intact skin and mucous membranes lining the respiratory, digestive, gastrointestinal, urinary, and reproductive systems provide physical protection against microbial invasion. The smallest of injuries to these physical barriers can provide a portal of entry for pathogens. The mucus of mucous membranes traps particles, which are then removed by the mechanical actions of cilia, called the ciliary escalator (Figure 20.17). The term ciliary escalator describes the process by which the ciliary action moves the mucus from the apical surface of the mucous membrane of the trachea to the oral cavity. The mucus, together with the trapped particles, is then swallowed together with the trapped particles and moved to the stomach, where the particles and microbes are destroyed by the acidity (pH 2) of the stomach’s environment. Sneezing and coughing assist in the removal of mucus from the upper respiratory tract. In smokers, the cilia of the respiratory tract are damaged and the ciliary action is impaired or absent; therefore the mucus is not easily transported to the oral cavity and must be removed by coughing actions (smoker’s cough).
Second Line of Defense
Phagocytosis and Phagocytes
Steps in phagocytosis (Figure 20.18):
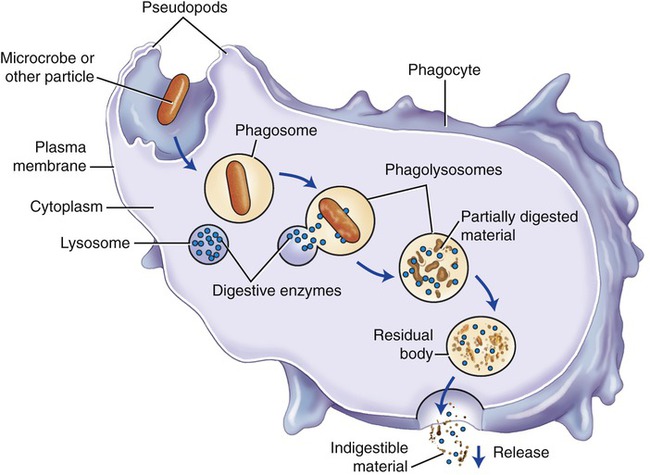
Phagocytosis is part of the second line of defense and involves chemotaxis, the movement of phagocytes to the invaded site; adhesion; ingestion; and digestion.
• Chemotaxis is the guided movement of phagocytes to the invaded site. It is the result of a chemical attraction caused by chemotactic agents. In general, this is achieved through the action of chemokines produced during the complement cascade (see Complement System later in this chapter).
• Adhesion is the process by which phagocytes attach to foreign substances before their ingestion.
• Ingestion is the process by which the membrane of the phagocyte extends pseudopods, surrounding the particle to be ingested.
• Digestion occurs when the phagosome merges with lysosomes within the phagocytotic cell to produce a phagolysosome. The digestive enzymes of lysosomes break down and dissolve the invaders, followed by the release of these digested materials from the phagocyte into the extracellular fluid compartment.
Inflammatory Response
The inflammatory reaction is a protective mechanism, which under certain circumstances may be harmful. Initiating events of an inflammation can be either injury or the presence of antigens, but also extremes of temperature, chemicals, and radiation. If the inflammatory reaction begins when the skin is broken due to a minor injury, it is often strong enough to prevent disease by stopping pathogenic microbes from entering other tissues (Figure 20.19).
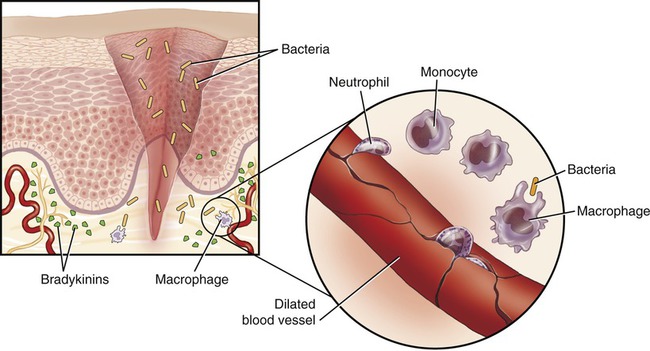
Inflammation occurs when microbes enter damaged tissue. In response to tissue damage blood vessels also dilate, bradykinin is released by the damaged endothelial cells, and monocytes/macrophages and neutrophils leave the blood vessels in an effort to destroy the invaders.
Steps of inflammation include the following:
1. An injury to capillaries and tissue cells is followed by the release of bradykinin from the injured cells.
2. Bradykinin activates sensory nerve impulses, which initiate pain.
3. The sensation of pain stimulates mast cells and basophils to release histamine.
4. Both bradykinin and histamine cause capillary dilation, increase blood flow, and increase capillary permeability in the infected area.
5. The break in the skin allows bacteria to enter the tissue, which results in the migration of neutrophils and monocytes to the site of injury.
6. Neutrophils phagocytize bacteria and destroy them via the hydrolytic enzymes of their lysosomes.
7. Monocytes that matured into macrophages leave the bloodstream and quickly phagocytize microbes.
The symptoms of inflammation (Box 20.1) include redness (rubor), increased temperature (calor), swelling (tumor), and pain (dolor) in the inflamed area. The redness and increased temperature are caused by the dilation of capillaries and increased blood flow in the region. The increased temperature increases the metabolic rate of the cells to increase the speed of healing. Heat also increases the activities of phagocytotic and other defensive cells. The sensation of pain results from stimulation of nociceptors (sensory receptors) caused by edema (excessive tissue fluid), and the release of prostaglandins by injured cells or bacterial toxins. Swelling (edema) is due to increased permeability of the capillaries and results in seeping of blood plasma components into the tissues. This capillary leakage provides a path to the site of infection for rapid action of infection-fighting cells and clotting factors.
Fever
Fever or pyrexia is a systemic response to extensive inflammation or microbial invasion (Box 20.2). A body temperature above 37.8° C (100° F) is generally considered to be a slight (mild) fever and helps the body to eliminate pathogenic organisms. Although high fever is dangerous, within limits it can also be beneficial if it reduces or eliminates the growth and reproduction of the invading microorganisms. The increase in body temperature is regulated by the hypothalamus in response to pyrogens, which are fever-producing substances released from white blood cells in response to microbial invasion. Also, microbial toxins released into the bloodstream can act as pyrogens. Pyrogens are transported by the bloodstream to the hypothalamus, where they stimulate specific neurons that release prostaglandins. Prostaglandins raise the physiological set point of the body temperature, which results in shivering (chills), a physiological response that initiates a rise in body temperature. Shivering involves increased muscle contraction, generating the heat necessary to increase body temperature. When the pathogens are eliminated the set point of the body temperature is lowered to pre-fever levels by evoking the physiological response of perspiration to cool off the body (the fever breaks).
Cytokines
Cytokines are small proteins considered to be chemical mediators of the immune response and are secreted by cells of the immune system. They are chemical messengers that allow cells within the immune system to communicate with each other and organize the attack on an invader. The same cytokine may be produced by several different cells and the same cytokine might have a different effect in different circumstances. This phenomenon is called pleiotropy. In addition, different cytokines may mediate the same activity depending on the situation; this is called redundancy. Many cytokines are referred to as interleukins (ILs) and are numbered according to when they were discovered, similar to the numbering of blood-clotting factors. The term interleukin describes the chemicals that function as mediators between the white blood cells (inter + leukocytes). Three groups of cytokines have been described according to their functional properties: cytokines that play a role in the regulation of hematopoiesis, cytokines that affect the inflammatory response, and cytokines that regulate the adaptive immune response (Table 20.3).
TABLE 20.3
Selected Cytokines and Their Activities
Cytokine | Source | Function |
IL-1 | Macrophage, monocytes | T- and B-cell activation; fever; inflammation |
IL-2 | T cells | T-cell proliferation; activation of B cells |
IL-3 | T cells | Stimulates growth of stem cells and mast cells |
IL-4 | T cells | Stimulates growth of B cells and some T cells |
IL-5 | T cells | B cells; eosinophil differentiation |
IL-6 | Macrophages, B cells and T cells, other cells | Inflammation, hematopoiesis, differentiation of B cells |
IL-7 | Fibroblasts, endothelial cells | Early B- and T-cell differentiation |
IL-8 | Monocytes | Attracts granulocytes |
IL-11 | Bone marrow | Hematopoiesis |
IL-14 | Dendritic cells, T cells | B-cell memory |
Complement System
1. The classical complement pathway, triggered when an antigen–antibody complex (IgG or IgM) binds to the C1 component
2. The alternative complement pathway, activated by C3 component hydrolysis on the surface of a pathogen
3. The lectin pathway, activated when mannose-binding lectin bound to carbohydrate of a pathogen interacts with an enzyme similar to C1
Third Line of Defense
Cell-mediated Immunity
The cell-mediated immune system is controlled by T cells, which are highly specialized lymphocytes circulating in the blood and lymph to fight bacteria, viruses, fungi, protozoans, and any other substances or cells that are foreign to the body. Neither cytotoxic T cells nor helper T cells can recognize an antigen floating in the blood or lymph and must be activated by an antigen-presenting cell, such as a macrophage (Figure 20.20).
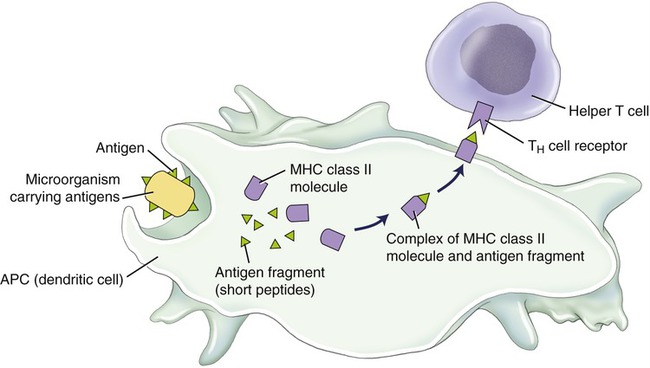
The categories of T cells (T lymphocytes) are as follows:
• Cytotoxic/killer T cells (CD8) recognize antigens presented within class I MHC molecules and will attack and destroy antigen-bearing cells such as virus-infected cells and cancer cells by releasing perforin and granzymes. Perforin molecules form a hole in the infected cells, allowing entry of the granzymes, which initiate apoptosis.
• Helper T cells (CD4) recognize antigens presented within class II MHC molecules and will aid the immune response by releasing cytokines that stimulate growth and division of cytotoxic T cells and B cells. In addition, they attract macrophages and further enhance the capabilities of macrophages to phagocytose microbes.
• Suppressor T cells (a subpopulation of CD8 T cells) release chemicals to regulate the immune response by suppressing both the further development of helper T cells and the antibody production by plasma B cells.
• Memory T cells represent the population of T cells that persists after suppression of the immune response. These cells recognize and respond to pathogens that previously invaded the body. They secrete cytokines to stimulate macrophages and B cells in response to repeated invasions.
Antibody-mediated Immunity (Humoral Immunity)
B cells produce specific antibodies and regulate the antibody-mediated immune response to viral and bacterial antigens. Small, naive B cells are mature immunocompetent cells that are stimulated to proliferate and differentiate by antigens that bind to their surface receptors. Each B cell carries a specific surface antibody that recognizes a specific antigen. After an antigen has been recognized, B cells become activated (sensitized) and start dividing into plasma cells and memory cells. This process is called clonal selection (Figure 20.21) and is enhanced by helper T cells (CD4). The majority of the clones become short-lived plasma cells that produce the specific antibodies and the others become long-lasting memory cells. Once enough antibodies have been produced by the B cells to eliminate the antigens, the development of plasma cells ceases under the direction of suppressor T cells. Memory B cells remain and they are responsible for long-term immunity. They will recognize the same antigen when it enters the system again and will divide quickly to produce new plasma and memory cells.
Active Immunity
• Naturally acquired active immunity results when an individual becomes ill from a particular pathogen and recovers by producing specific antibodies (immunoglobulins) via the immune system. This type of immunity provides long-term protection against this particular pathogen. The extent of time for protection is dependent on the nature of the pathogen.
• Artificially acquired active immunity is a result of a controlled, intentional exposure to weakened, fragmented, killed pathogens or bacterial toxins. By administering a safe amount and form of an antigen, the body’s immune system will produce its own antibodies and memory cells. This type of antigen is called a vaccine. A successful vaccine should have the ability to elicit the appropriate immune response, provide long-term protection, and be safe, stable, and inexpensive. Several different types of vaccines are currently used in the practice of immunization.
• Attenuated microbes are living but nonvirulent strains of a microorganism. This type of live vaccine provides long-lived immunity by administration of a single dose. Although not likely, a reversion to virulence of the attenuated microbes is possible and especially dangerous for immunocompromised people.
• Vaccines from killed or fragmented microbes do not elicit as strong an immune response, the immunity is short-lived, and multiple doses are needed. It is expensive to prepare and the efficacy of the vaccine does not rely on the viability of the organisms.
• DNA immunization through recombinant DNA technology uses genes for viral antigens and not the antigen itself as the immunogen source. The host cells in the individual to be immunized take up the DNA and produce viral antigens by the usual cellular mechanisms. The newly produced antigen is then presented on the cell surface with host MHC class I and class II molecules. Subsequent contact with immunocompetent cells evokes an immune response.
• Toxoids are bacterial or viral toxins that have been altered to become nontoxic. Injections of toxoids provide protection against the toxin, but not against the pathogen itself.
The quantity of antibodies produced and ultimately found in serum after vaccination or an actual infection is the antibody titer. The initial vaccination triggers a rise in the antibody titer that gradually weakens, and booster shots (second injections) are often administered to keep the antibody titer high enough to prevent infection. The secondary response is more effective than the primary response. The memory B cells quickly divide to form more memory B cells and a large number of plasma cells produce a great number of antibodies at a moment’s notice. When an immunized individual is later exposed to the particular pathogen, the immune response is even more intense, thus preventing the infection. For more information about vaccination, see the Life Application box.
Passive Immunity
• Oligosaccharides and mucins, which interfere with the adherence of microbes to host cells
• The iron-binding substance lactoferrin, which makes iron unavailable to most bacteria
• Fibronectin, which increases the activity of the infant’s macrophages
• Cytokines, which enhance the activity of certain immune cells
• Lysozymes, which break down the peptidoglycan layer in bacterial cell walls
Diseases Caused by the Immune System
Allergy/Hypersensitivity Reactions
An allergy is a strong immune response to an allergen, a substance that usually is not harmful to the body but causes hypersensitivity reactions. Substances such as pollen, mold, dust (most likely dust mites), cat dander, foods, insect stings or bites, certain foods, and medicines can cause these reactions (Table 20.4). Allergies are caused by an overactive immune system producing antibodies against substances that are nonpathogenic and do not cause problems for the human body.
TABLE 20.4
Examples of Allergic Responses, Symptoms, and the Causative Allergens
Allergic Response | Symptoms | Allergen |
Allergic rhinitis (hay fever), asthma | Sneezing, nasal congestion, difficulty breathing | Mold, pollen, animal dander, dust mites |
Food allergy | Nausea, vomiting, abdominal cramps, diarrhea | Eggs (mostly egg white), fish (especially shellfish), chicken, milk and milk products, nuts, wheat, soybeans |
Hives | Reddening and swelling of specific skin areas | Selected foods and food additives, insect bites, drugs (e.g., penicillin), cosmetics |
Anaphylactic shock | Dilation of blood vessels, dizziness, nausea, diarrhea, unconsciousness, possibly death | Insect stings, medications, certain foods |
Immediate Hypersensitivity (Type I)
HEALTHCARE APPLICATION
Examples of Hypersensitivity Reactions
Allergy | Symptoms | Cause | Treatment |
Type I Hypersensitivity Reactions: Immediate or Anaphylactic | |||
Dermatitis (eczema) | Itchy, inflamed skin caused by a variety of antigens with different ports of entry | Contact with an antigen found in gloves, new clothes, plants, foods; stress or neurological conditions | Frequent washing and shampooing with medicated soap; topical corticosteroids, and antibiotics |
Allergic rhinitis (hay fever) | Nasal congestion, sneezing, coughing, extensive mucous secretion, itchy and teary eyes occurring seasonally or year round | Pollens (tree, grass, and weed), molds, house dust mites, pets, cockroaches, rodents | Environmental control measures; allergen avoidance; antihistamines, decongestants, or both; immunotherapy (desensitization) |
Asthma | Impaired breathing, bronchoconstriction, wheezing, shortness of breath, coughing, abnormal breathing sounds, chest tightness | Dust mites, pet dander, pollen, molds, etc. | Antiinflammatory agents, bronchodilators |
Food allergies | Tingling sensation in the mouth, swelling of the tongue and throat, difficulty breathing, hives, vomiting, abdominal cramps, diarrhea, drop in blood pressure, loss of consciousness and death | Products in food, food additives, and/or preservatives | Avoidance of the allergy-causing food, treatment of symptoms; no medications are currently available to cure food allergies |
Drug allergies | Hives, skin rash, itching of skin or eyes, wheezing, swelling of the lips, tongue, or face; anaphylaxis | A side effect to chemotherapy; antibiotics (most often penicillin) | Avoidance of offending medication; treatment of symptoms, desensitization |
Anaphylactic reactions | Dizziness, nausea, difficulty breathing, profound fall in blood pressure, unconsciousness, and sometimes death. Response usually after first exposure | Insect bites, certain foods, medications | Adrenalin, antihistamine, corticosteroids, oxygen when needed. Usual treatment for shock |
Type II Hypersensitivity Reactions: Antibody Dependent or Cytotoxic | |||
Hemolytic disease of the newborn | Jaundice, pallor, enlarged liver and/or spleen, generalized swelling, respiratory distress, death | Sensitized mother against fetal blood (see earlier in this chapter) | Before birth: intrauterine transfusion or early induction of labor; plasma exchange in the motherAfter birth: transfusion with compatible packed red blood; exchange transfusion with a compatible blood type; sodium bicarbonate for correction of acidosis, assisted ventilation |
Goodpasture’s syndrome |
Lung disease: dry cough, minor breathlessness, symptoms may last for many years before severe symptoms such as impairment of oxygenation and coughing up blood develop Kidney disease: affects the glomeruli causing a form of nephritis; acute renal failure can occur Lung and kidney disease may occur alone or together depending on the patient |
Autoimmune Diseases
Autoimmunity occurs when the immune system is unable to distinguish between self-antigens and nonself-antigens and attacks cells, tissues, or organs of the body. Autoimmune diseases can be subdivided into organ-specific diseases, such as Graves’ disease, and nonorgan-specific diseases, such as myasthenia gravis. Autoimmune diseases often occur after an individual has experienced an infection or traumatic injury that activates cytotoxic T cells and antibody production against the body’s own cells and/or organs. Exact causes of autoimmune diseases are still unknown, but susceptibility based on genetics and gender is a possibility. This chapter addresses a few of the most common autoimmune diseases (Table 20.5).
TABLE 20.5
Disease | System | Symptoms | Tests |
Myasthenia gravis | Skeletal muscles | Progressive muscle weakness, paralysis, death | Autoantibodies against acetylcholine receptors |
Multiple sclerosis | Central nervous system | Problems with coordination and balance, difficulty in speaking and walking; paralysis, tremors, numbness and tingling sensation in extremities | Neurological examinations, MRI, MRS, testing of the cerebrospinal fluid |
Graves’ disease | Thyroid gland | Insomnia, irritability, weight loss, sweating, muscle weakness, bulging eyes, shaky hands | Blood test for TSH |
Hashimoto’s thyroiditis | Thyroid gland | Tiredness, depression, cold sensitivity, weight gain, dry hair, muscle weakness, constipation; sometimes asymptomatic | Blood test for TSH |
Systemic lupus erythematosus | Affects many organ systems | Swelling and damage to joints, skin, kidneys, heart, lungs, blood vessels, and brain; rash across nose and cheeks (“butterfly rash”) | Blood and urine tests |
Rheumatoid arthritis | Systemic, mostly affecting joints, but also the nervous system, lungs, and skin | Inflammation of joints, muscle pain, weakness, fatigue, loss of appetite, weight loss | Blood tests |
Summary
• Immunology is the study of the events in the immune system, whereas immunity describes the immune response to invading microorganisms.
• The circulatory system (lymphatic and cardiovascular) helps to facilitate the immune response, by distributing immune system cells and antibodies throughout the body. Antigens and antibodies interact to form antigen–antibody complexes during an immune reaction.
• Tissues and organs of the immune system include the lymphatic vessels, lymphoid tissue, lymphatic nodules, tonsils, adenoids, lymph nodes, spleen, thymus, and red bone marrow. The cells of the immune system are leukocytes, including basophils, eosinophils, neutrophils, monocytes, macrophages, lymphocytes (B and T cells), and dendritic cells. Each of these components fulfills a particular function in the immune response.
• Antigens stimulate phagocytosis, provided by a variety of phagocytes, and the production of antibodies by B cells. The immune response can be nonspecific (innate) and/or specific (adaptive).
• The first line of defense is a nonspecific defense mechanism and consists of physical and chemical barriers. Physical barriers include the skin, mucous membranes, sweat, tears, and urine flow. Chemical barriers include the pH of the skin, fatty acids and lactic acids from sebum, mucus containing lysozymes, and the pH of the stomach.
• The second line of defense is also part of the innate immune system, involving phagocytes (phagocytosis), inflammation, fever, interferons, cytokines, and the complement system.
• The third line of defense is composed of acquired/adaptive defense mechanisms, consisting of cell-mediated (T and B cells) and humoral (antibody-mediated) immune responses.
• Active and passive immunity require the presence of antibodies. In active immunity, B cells produce antibodies, whereas in passive immunity the body receives antibody from an external source. Both active and passive immunity can be naturally or artificially acquired.
• An overactive immune system can cause autoimmune diseases and hypersensitivity reactions. Autoimmune diseases occur when the immune system is unable to distinguish between self and nonself. They are classified as organ specific and nonorgan specific.
• Hypersensitivity reactions are classified as types I, II, III, and IV.
• During the aging process, people become more susceptible to microbial infections and autoimmune diseases because of deficiencies in their lymphatic and immune systems.
Review Questions
1. The antibody found in body secretions is:
a. Substance initiating an allergic response
b. Marker on the cell surface of macrophages
3. Which of the following cell types secretes antibodies?
4. Which of the following provide defense against viral infections?
5. Which of the following cells is a granulocyte?
6. Immunity that is a result of an actual infection is called:
a. Artificially acquired passive immunity
b. Artificially acquired active immunity
7. A substance capable of raising the body temperature is:
8. When an organ or tissue is transplanted between genetically different individuals from the same species it is called a(n):
9. Which of the following is a systemic autoimmune disease?
10. Which of the following is not part of the second line of defense?
11. Enzymes that attack the peptidoglycan layer of bacteria and are present in perspiration, nasal secretion, saliva, and tears are __________.
12. Delayed hypersensitivity is a result of __________.
13. B cells are responsible for __________-mediated immunity.
14. Substances that stimulate the production of antibodies are called __________.
15. The body’s decreased ability to fight infections is called __________.
16. Describe and explain the three lines of host immune defense.
17. Name and describe the steps of inflammation.
18. Describe the structure and function of the various antibodies.