Chapter 74 The Human Genome
Fundamentals of Molecular Genetics
DNA consists of a pair of chains of a sugar-phosphate backbone linked by pyrimidine and purine bases to form a double helix (Fig. 74-1). The sugar in DNA is deoxyribose. The pyrimidines are cytosine (C) and thymine (T); the purines are guanine (G) and adenine (A). The bases are linked by hydrogen bonds such that A always pairs with T and G with C. Each strand of the double helix has polarity, with a free phosphate at one end (5′) and an unbonded hydroxyl on the sugar at the other end (3′). The two strands are oriented in opposite polarity in the double helix.
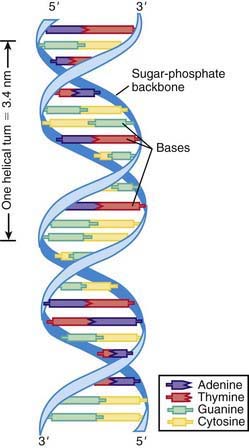
Figure 74-1 DNA double helix, with sugar-phosphate backbone and nitrogenous bases.
(From Jorde LB, Carey JC, Bamshad MJ, et al, editors: Medical genetics, ed 2, St Louis, 1999, Mosby, p 8.)
A prototypical gene consists of a regulatory region, segments called exons that encode the amino acid sequence of a protein, and intervening segments called introns (Fig. 74-2). Transcription starts at the promoter region and continues through the entire length of the gene to form mRNA. The introns are removed and the exons spliced together to form a mature message, which is exported to the cytoplasm. There the mRNA is bound to ribosomes and translated into protein.
Genetic Variation
The process of producing protein from a gene is subject to disruption at multiple levels owing to alterations in the coding sequence (Fig. 74-3). Changes in the regulatory region can lead to altered gene expression, including increased or decreased rates of transcription, failure of gene activation, or activation of the gene at inappropriate times or in inappropriate cells. Changes in the coding sequence can lead to substitution of one amino acid for another (missense mutation or nonsynonymous) or creation of a stop codon in the place of an amino acid codon. Some single-base changes do not affect the amino acid (silent or wobble mutation or synonymous), because there may be several codons that correspond with a single amino acid. Amino acid substitutions can have a profound effect on protein function if the chemical properties of the substituted amino acid are markedly different from the usual one. Other substitutions can have a subtle or no effect on protein function, particularly if the substituted amino acid is chemically similar to the original one.