Surgical and Nonsurgical Trauma
Causes of Enucleation
I. Figure 5.1 shows that trauma (surgical and nonsurgical) is the number-one cause of enucleations (35%).
Complications of Intraocular Surgery1
Adult Cataract Surgery
Immediate
Complications occurring from the time the decision is made to perform surgery until the patient leaves the operating room are considered immediate.
A. Misdiagnosis: Not all cataracts are primary, but they may be secondary to such things as trauma, inflammation, neoplasm (Fig. 5.2), or metabolic disease.
B. Faulty technique may result in and/or from:
3. Increased intraocular pressure because of a retrobulbar hemorrhage or poorly placed lid speculum
4. Misalignment of the entering incision
5. Splitting off (stripping) of Descemet’s membrane from the posterior cornea (can lead to postoperative corneal edema; Fig. 5.3)
7. Endothelial cell loss can accompany intraocular surgery, particularly that directly on the anterior segment such as cataract surgery. Endothelial cells loss is greater in diabetic patients even if under good glycemic control compared to nondiabetic individuals.
10. Calcification within the optic material of hydrophilic intraocular lenses has been reported.
A. May occur if the iris is inadvertently cut.
B. Bleeding invariably stops in a short time if patience and continuous saline irrigation are used.
III. Radial tear of the anterior capsulorhexis, rupture of the posterior lens capsule, or a zonular dialysis
B. They also predispose to malposition of the lens implant and irregular pupil.
IV. Loss of vitreous, which occurs in approximately 3–9% of cataract cases, leads to an increased incidence of iris prolapse, bullous keratopathy, epithelial downgrowth, stromal ingrowth, wound infection, endophthalmitis, updrawn or misshapen pupil, vitreous bands, postoperative flat chamber, secondary glaucoma, poor wound healing, neural retinal detachment, cystoid macular and optic disc edema, vitreous opacities and hemorrhage, expulsive choroidal hemorrhage, and “chronic ocular irritability.”
A. Risk factors for decreased vision following cataract surgery include older age, short axial length, any ocular comorbidity, age-related macular degeneration, diabetic retinopathy, amblyopia, corneal pathology, previous vitrectomy, and posterior capsule rupture during surgery. Importantly, only intraoperative posterior capsular rupture is not intrinsic to the patient.
B. There may be delayed prolapse of vitreous into the anterior chamber following cataract surgery.
V. Expulsive choroidal hemorrhage (Fig. 5.4; see also Figs. 16.27 and 16.28)
VI. Intraoperative floppy iris syndrome
A. It is associated with a history of preoperative use of alpha antagonists, such as tamsulosin, usually for benign prostatic decrease in urinary flow.
Postoperative
Postoperative complications may arise from the time the patient leaves the operating room until approximately two or three months after surgery.
I. Atonic pupil
B. The site of the lesion appears to be in the iris sphincter.
II. Flat anterior chamber (extremely rare)2—most chambers refill within 4–8 hours after surgery.
A. Most postoperative flat chambers are secondary to a complicated cataract surgery.
1. Faulty wound closure (Fig. 5.5): Faulty apposition of the wound edges can lead to poor wound healing and a “leaky” wound. Hypotony and a flat anterior chamber result.
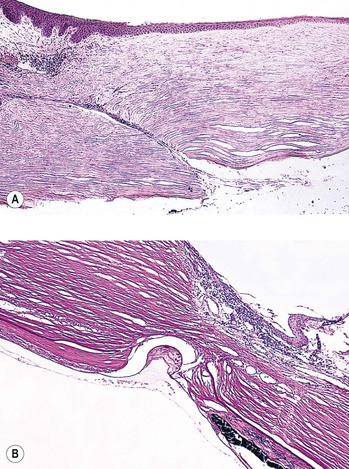
2. Choroidal detachment (“combined” choroidal detachment) is not a true detachment but, rather, an effusion or edema of the choroid (hydrops), and it is always associated with a similar process in the ciliary body. This complication occurs much more commonly following glaucoma surgery than following cataract surgery with hypotony as the common predisposing feature.
Frequently, the edema fluid is “washed out” of tissue sections and the spaces appear empty.
3. Iris incarceration (Fig. 5.6; iris within the surgical wound) or iris prolapse (iris through the wound into the subconjunctival area) acts as a wick through which aqueous can escape, resulting in a flat chamber.
5. Fistulization of the wound (Fig. 5.7) is usually of no clinical significance, but occasionally, it may be marked and lead to a large inadvertent filtering bleb, hypotony, flat chamber, corneal astigmatism, and epiphora.
6. Vitreous wick syndrome consists of microscopic-scale wound breakdown leading to subsequent vitreous prolapse, thus creating a tiny wick draining to the external surface of the eye.
a. In some cases, severe intraocular inflammation develops and resembles a bacterial endophthalmitis.
b. Infection can gain entrance into the eye through a vitreous wick.
1. Pseudophakic, pupillary-block glaucoma may occur from an intraocular lens. The prevalence varies with different types of intraocular lenses and from surgeon to surgeon.
a. Most cases occur in eyes that have anterior chamber intraocular lenses placed but do not have a peripheral iridectomy performed (Fig. 5.8). The glaucoma in the postoperative period is usually caused by a pupillary-block mechanism.
III. Striate keratopathy (“keratitis”)
B. Striate keratopathy is usually completely reversible and disappears within a week.
A. Most postoperative hyphemas occur within 24–72 hours after surgery.
A. Causes
1. “Traumatic” extracapsular cataract extraction
2. Glaucoma, usually pupillary-block glaucoma (pseudophakic glaucoma)
3. Vitreous (Fig. 5.10) or iris adherent to the surgical wound or within it, or adherent to the corneal endothelium
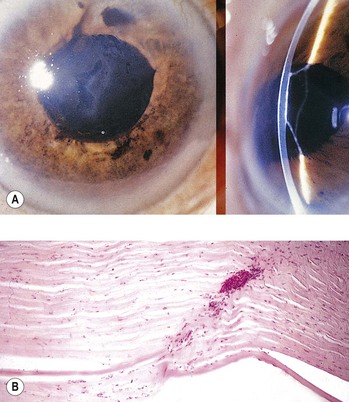
4. Splitting of Descemet’s membrane from the posterior cornea (Descemet’s membrane detachment) (see Fig. 5.3)
B. Histologically (see Figs. 8.50, 8.55, 16.26, and 16.27), the basal layer of epithelium is edematous early.
1. In time, subepithelial collections of fluid (bullae or vesicles) may occur.
A. It is usually secondary to extension of a choroidal hemorrhage.
IX. Inflammation
A. Endophthalmitis (see Fig. 3.1)
4. An increased prevalence of endophthalmitis is seen in diabetic patients.
B. Uveitis
C. Toxic anterior segment syndrome (TASS) and toxic endothelial destruction syndrome (TEDS)
2. Possible causes that have been cited include intraocular solutions with inappropriate chemical composition, concentration, pH, or osmolality; preservatives; denatured ophthalmic viscosurgical devices; enzymatic detergents; bacterial endotoxin; oxidized metal deposits and residues; and factors related to intraocular lenses, such as residues from polishing or sterilizing compounds.
4. Impurities in an autoclave steam mixture have also been cited as causing one outbreak of TASS.
5. An iris-supported phakic intraocular lens has been associated with TASS.
X. Intraocular lens implantation
A. Lens implant subluxation and dislocation (Fig. 5.11)
1. The posterior chamber lens implant may subluxate nasally, temporally, superiorly (sunrise syndrome), or inferiorly (sunset syndrome).
a. A recent study of dislocation of in-the-bag intraocular lenses (IOLs) found a higher association with a history of prior vitrectomy and a decreased association with pseudoexfoliation than previous studies. Nevertheless, in-the-bag IOL dislocation was associated with pseudoexfoliation in 7/19 patients in another study. The pseudoexfoliation specimens displayed capsular contraction, shrinkage in diameter of the capsular bag, and dehiscence of the zonular fibers. Only slight capsular contraction was present in the other specimens; however, they exhibited capsular delamination at the equatorial region of the capsule where the zonular fibers had completely disappeared.
1) Bilateral in-the-bag IOL dislocation has been found in association with retinitis pigmentosa in an elderly man.
b. Blunt trauma may result in the expulsion of an IOL through a clear corneal wound.
5. Nuclear fragments of a surgically removed cataractous lens may be retained in the anterior chamber and contribute to corneal endothelial decompensation requiring return to the operating room for removal of the nuclear fragment. They may be associated with recurrent anterior uveitis.
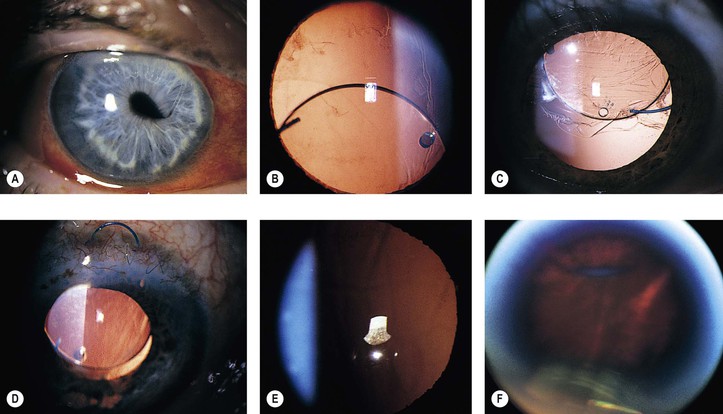
XII. Acute vitreomacular traction syndrome
C. Spontaneous resolution of the traction occurs within 10 days.
E. Permanent metamorphopsia and slightly decreased visual acuity also may be sequelae.
Congenital Cataract Surgery
Delayed
Delayed complications are those that occur after the second or third month after surgery.
I. Corneal edema secondary to:
A. The five entities listed under Corneal edema in the preceding subsection, Postoperative.
B. Intraocular lenses, especially iris-clip lenses (almost never seen anymore), may cause delayed corneal edema (Fig. 5.12).
C. Peripheral corneal edema (Brown–McLean syndrome), onset of edema, often delayed six years after surgery, is bilateral when the surgery is bilateral, mainly occurs in women, and is of historical interest only.
E. Lens opacities may be the sequelae of posterior chamber phakic intraocular lens implantation.
F. Secondary (“after”) cataract
1. Posterior capsule opacification (Fig. 5.13)
2. Elschnig’s pearls (Fig. 5.15) result from aberrant attempts by remaining lens cells attached to the capsule to form new lens “fibers.”
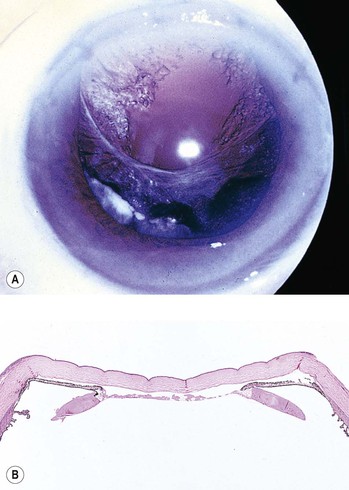
3. Soemmerring’s ring cataract (Detmar Wilhelm Soemmerring, 1793–1871; see Fig. 5.15) results from loss of anterior and posterior cortex and also loss of the nucleus but with retention of equatorial cortex.
4.