Diabetes Mellitus
Natural History
II. DR is a leading cause of blindness in the United States.
A. More than three-fourths of the blind are women.
C. The most important factor in the occurrence of DR is how long the patient has been diabetic.
1. Although approximately 60% of patients develop retinopathy after 15 years of diabetes, and almost 100% after 30 years, the risk of legal blindness in a given diabetic person is only 7–9% even after 20–30 years of DM.
3. Over a 25-year follow-up period, the mortality in diabetic blind individuals is 61% compared to 41% for those who are not blind. Moreover, there is significant racial difference in the quality-adjusted life-years for individuals with diabetes and visual impairment, with whites having a higher quality-adjusted life expectancy compared to black individuals.
Factors associated with mortality are glycemic regulation, dyslipidemia, and creatinine level.
III. In juvenile DM, PDR is uncommon in patients younger than 20 years of age and almost unheard of in patients younger than 16 years of age.
IV. Most diabetic patients never acquire PDR, and in those who do, it develops only after at least 15 years of DM.
Rarely, a patient presents with BDR, or even with PDR, before any systemic evidence of DM (e.g., hyperglycemia) is discovered.
A. Primary open- and closed-angle glaucoma occurs more often in diabetic patients than in nondiabetic individuals.
D. Other risk factors for the development of DR include hypertension and abdominal obesity.
VI. Diabetic peripheral neuropathy affects approximately 50% of diabetic patients.
Retinal Vasculature in Normal Subjects and Diabetic Patients
Figure 15.1 shows examples of retinal vasculature in normal subjects and diabetic patients (see also section Neural Retina, later in this chapter).
Conjunctiva and Cornea
I. Conjunctiva
B. Transmural lipid imbibition may occur in conjunctival capillaries in diabetic lipemia retinalis (Fig. 15.2). Histologically, lipid-laden cells, either endothelial cells or subintimal macrophages, are present projecting into and encroaching on conjunctival capillary lumens.
II. Cornea
A. Epithelium
B. Endothelium
2. The abnormalities are more pronounced in patients with PDR. Sub-basal nerve abnormalities correlate with reduced corneal epithelial basal cell density.
a. Corneal nerve tortuosity may relate to the severity of the neuropathy in diabetic patients.
Lens
I. “Snowflake” cataract of juvenile diabetic patient
A. The cataract consists of subcapsular opacities with vacuoles and chalky-white flake deposits.
C. The histopathology has not been defined.
II. Adult-onset diabetic cataract (Fig. 15.3)
A. The cataract (cortical and nuclear) is indistinguishable clinically and histopathologically from the “usual” age-related cataracts. Diabetic patients, however, are at an increased risk for cataracts compared with nondiabetic subjects. Nevertheless, diabetes is not universally accepted as a risk factor for nuclear cataracts.
1. Diabetes is a strong risk factor for the development of posterior subcapsular cataract.
2. Apoptosis plays an important role in the development of cataracts in DR compared to senile cataract.
B. Patients with diabetes may have transient lens opacities and induced myopia during hyperglycemia.
Ciliary Body and Choroid
I. Basement membrane of ciliary pigment epithelium (external basement membrane of ciliary epithelium; Fig. 15.6)
III. Fibrovascular core of ciliary processes (see Fig. 15.6)
A. Fibrosis results in obliteration of capillaries in the “core” of the ciliary processes.
B. The capillary basement membrane is often significantly thickened.
IV. Choriocapillaris, Bruch’s membrane, and retinal pigment epithelium (Figs. 15.7 and 15.8)
B. The cuticular portion of Bruch’s membrane (basement membrane of the retinal pigment epithelium; basal laminar-like deposits) may become thickened, and the lumen of the choriocapillaris may become narrowed by endothelial cell proliferation and basement membrane elaboration.
V. Arteries and arterioles of choroid (see Figs. 15.7 and 15.8)
Arteriosclerosis occurs at a younger age in diabetic patients than in the general population.
A. The incidence increases sharply beyond the 15th year of the disease.
B. The change is reflected in atherosclerosis and arteriolosclerosis of the choroidal vessels.
Neural Retina
I. The cause(s) of DR (Table 15.1; see also discussion of PDR later in this section)
A. Although DR is usually discussed relative to the characteristic and clinically apparent vascular changes, recent evidence suggests that DR involves alterations in all of the retinal cellular elements, including vascular endothelial cells and pericytes; glial cells, including macroglia (Müller cells and astrocytes) and microglia; and neurons, including photoreceptors, bipolar cells, amacrine cells, and ganglion cells (Table 15.2). Each of these elements makes unique contributions to visual function and participates in multiple homeostatic relationships to the other cellular elements.
D. Inflammation appears to play a significant role in the pathogenesis of diabetic retinopathy.
TABLE 15.1
Proposed Pathogenic Mechanisms for Diabetic Retinopathy
Proposed Mechanism* | Putative Mode of Action | Proposed Therapy |
Aldose reductase | Increases production of sorbitol (sugar alcohol produced by reduction of glucose) and may cause osmotic or other cellular damage | Aldose reductase inhibitors (clinical trials in retinopathy and neuropathy thus far have been unsuccessful) |
Inflammation | Increases adherence of leukocytes to capillary endothelium, which may decrease flow and increase hypoxia; may also increase breakdown of blood–retinal barrier and enhance macular edema | Aspirin (ineffective in the Early Treatment Diabetic Retinopathy Study but did not increase vitreous hemorrhage; therefore not contraindicated in patients with diabetes who require it for other reasons); corticosteroids (intravitreal injection or slow-release implants for macular edema now being tested) |
Protein kinase C | Protein kinase C upregulates VEGF and is also active in “downstream” actions of VEGF following binding of the cytokine to its cellular receptor. Protein kinase C activity is increased by diacylglycerol, which is accelerated by hyperglycemia. | Clinical trials of a protein kinase Cβ isoform inhibitor in retinopathy have thus far been unsuccessful. |
Reactive oxygen species | Oxidative damage to enzymes and to other key cellular components | Antioxidants (limited evaluation in clinical trials) |
Nonenzymatic glycation of proteins; advanced glycation end products | Inactivation of critical enzymes; alteration of key structural proteins | Aminoguanidine (clinical trial for nephropathy halted by sponsor) |
Inducible form of nitric oxide synthase | Enhances free radical production; may upregulate VEGF | Aminoguanidine |
Altered expression of critical gene or genes | May be caused by hyperglycemia in several poorly understood ways; may cause long-lived alteration of one or more critical cellular pathways | None at present |
Apoptotic death of retinal capillary pericytes, endothelial cells | Reduces blood flow to retina, which reduces function and increases hypoxia | None at present |
VEGF | Increased by retinal hypoxia and possibly other mechanisms; induces breakdown of blood–retinal barrier, leading to macular edema; induces proliferation of retinal capillary cells and neovascularization | Reduction of VEGF by extensive (panretinal) laser photocoagulation; several experimental medical therapies being tested (specific VEGF inhibitors are used to treat neovascularization and macular edema) |
PEDF | Protein normally released in retina inhibits neovascularization; reduction in diabetes may eliminate this infection. | PEDF gene in nonreplicating adenovirus introduced into eye to induce PEDF formation in retina (phase I clinical trial ongoing) |
Growth hormone and IGF-1 | Permissive role allows pathologic actions of VEGF; reduction in growth hormone or IGF-1 prevents neovascularization. | Hypophysectomy (now abandoned); pegvisomant (growth hormone receptor blocker; brief clinical trial failed); octreotide (somatostatin analogue, clinical trial now in progress) |
* For all the proposed mechanisms, hyperglycemia accelerates the progression to diabetic retinopathy.
VEGF, vascular endothelial growth factor; PEDF, pigment epithelium-derived factor; IGF-1, insulin-like growth factor-1.
(Modified from Frank RN: Diabetic retinopathy. N Engl J Med 350:48, 2004.)
TABLE 15.2
Diabetic Alterations in Retinal Cellular Elements
Cell Type | Changes |
Vascular | Altered tight junctions |
Endothelial cell and pericyte death | |
Glial | Altered contacts with vessels |
Release inflammatory mediators | |
Impaired glutamate metabolism | |
Microglial | Increased numbers |
Release inflammatory mediators | |
Neuronal | Death of ganglion cells, inner nuclear layer |
Axonal atrophy |
(Modified from Gardner TW, Antonetti DA, Barber AJ et al.: Diabetic retinopathy: More than meets the eye. Surv Ophthalmol 47(Suppl 2):S253. © Elsevier, 2002.)
III. Specific constellation of vascular findings—clinical BDR
A. Loss of capillary pericytes (see Fig. 15.1)
1. In the normal retinal capillary, the pericyte-to-endothelial cell ratio is 1 : 1.
3. Pericyte death is accompanied by morphologic nuclear changes and lack of inflammation characteristic of apoptosis (see Chapter 1). Activation of nuclear factor-κB, induced by high glucose in diabetes, may regulate a proapoptotic program in retinal pericytes.
B. Capillary microaneurysms (Figs. 15.9 and 15.10)
2. An increase in the number of RCMs can be directly correlated with the loss of pericytes.
3. RCMs are formed in response to a hypoxic environment in which abortive attempts at neovascularization or regressed changes or both have been made in a previously proliferating vessel.
b. The retinal capillary endothelial cells proliferate and lay down increased amounts of basement membrane (Figs. 15.10 and 15.11).
c. Ultimately, all of the endothelial cells may disappear; ghost retinal capillaries result.
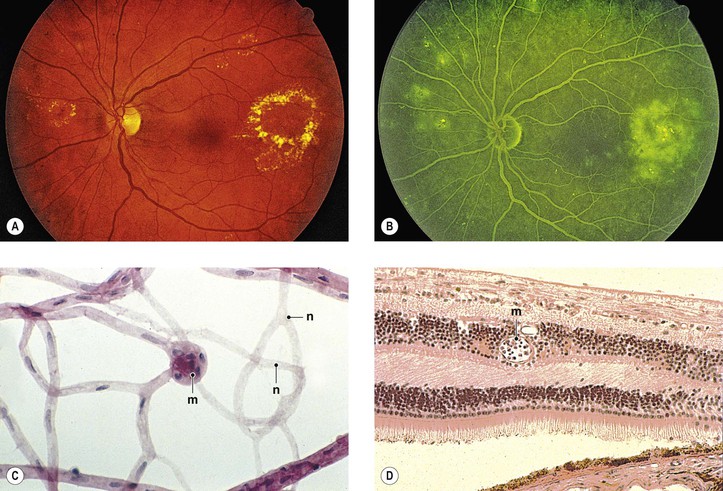
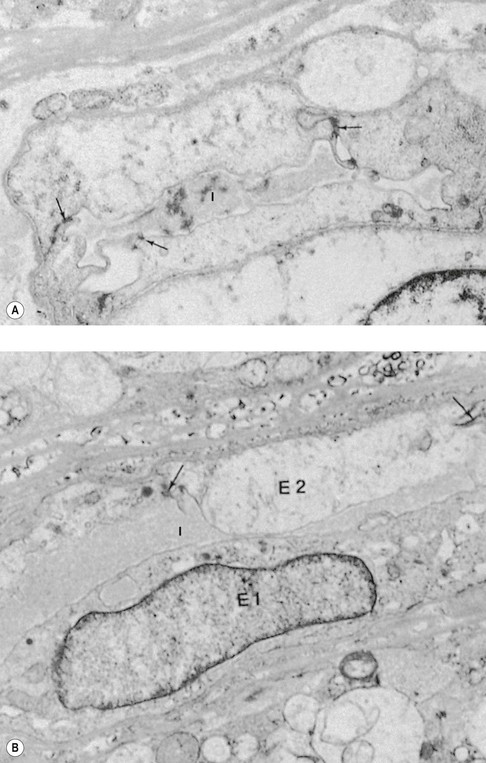