Chapter 48 Proliferative Diabetic Retinopathy
The earliest diabetes-induced changes in the retina are biochemical, hemodynamic, and cellular in nature. Often these are initially imperceptible clinically and may have no or minimal effect on vision. In contrast, proliferative diabetic retinopathy (PDR) represents an advanced stage of diabetic eye disease characterized by the growth of newly formed retinal vessels on the retina or optic disc that extend along the retinal surface or into the vitreous cavity, significantly increasing the risk for vision loss.1,2 Among patients with diabetes, nearly 25% with type 1 and 16% with type 2 will develop PDR after 15 years of diabetes.3,4 The rate of progression to PDR is highest among type 1 patients, with a 42% cumulative risk over 25 years.5 Furthermore, there is a strong association between PDR and uncontrolled systemic disease.6,7 The publication of landmark clinical trials establishing the importance of intensive glycemic control in preventing the onset and progression of retinopathy and other diabetic complications in both type 1 and type 2 diabetes has led to marked improvement in the medical care of patients with diabetes over the past two decades. With these improvements there has been a corresponding decline in the incidence of PDR.8–11 Nevertheless, the ocular complications arising from development of PDR remain a leading cause of severe vision loss in developed countries worldwide.10
Pathogenesis of proliferative diabetic retinopathy
Hyperglycemia and metabolic changes from diabetes lead to alterations in the retinal vasculature that result in reduced perfusion of the retinal tissue.12 This state of relative retinal ischemia is thought to be the primary angiogenic stimulus that plays a central role in the pathogenesis of PDR. Various angiogenic factors such as angiopoietin, erythropoietin, basic fibroblast growth factor (bFGF), insulin-like growth factor (IGF), protein kinase C (PKC), tumor growth factor (TGF), and platelet-derived growth factor (PDGF) have stimulatory or modulating activities during the development of PDR. However, based on in vivo and in vitro studies, the protein called vascular endothelial growth factor (VEGF) appears to be primarily responsible for the ischemia-driven angiogenic pathology in PDR.13–15 The role of VEGF in PDR is well supported by studies demonstrating high concentrations of VEGF in the vitreous of patients with PDR which are closely correlated with extent of disease activity.13,16 Following successful laser treatment and in patients with naturally quiescent PDR, VEGF vitreous concentrations were low or undetectable.13 Furthermore, the direct role for VEGF mediation of the neovascular response in PDR was demonstrated by showing that vitreous fluids from patients with active PDR were angiogenic in vitro, and this angiogenic stimulus could be blocked using a VEGF-specific inhibitor. Intraocular vessels from PDR and diabetes-induced iris neovascularization are exquisitely sensitive to VEGF inhibitors, often showing initial regression within one day.17
Although VEGF appears to be the primary direct causative angiogenic factor in PDR, the complex mechanisms regulating in vivo angiogenesis likely involve factors other than VEGF as well. Angiogenic pathways such as the angiopoietin/Tie-2 system modulate the effect of VEGF and directly affect retinal pericytes and endothelial cells, which are the principal cell types thought to be involved in the pathological processes of PDR.18,19 In addition, hyperglycemia reduces PDGF survival-promoting activity, thus leading to pericyte apoptosis and diabetic vasculopathy. This mechanism is driven by hyperglycemia-induced activation of protein kinase C-δ (PKC-δ) which leads to increased expression of a protein tyrosine phosphatase called Src homology-2 domain–containing phosphatase-1 (SHP-1). SHP-1 activation in turn mediates resistance to PDGF, resulting in loss of cellular survival mechanisms and increased pericyte apoptosis.20 Inhibition of SHP-1 is being investigated as a possible protective mechanism against initial retinal changes that underlie subsequent development of PDR.20 VEGF independent pathways such as that mediated by erythropoietin (EPO) have also been implicated in the development of PDR.21 Single nucleotide polymorphisms (SNPs) that increase EPO expression have been associated with development of PDR and severe renal disease in a small genetic study of three independent patient populations.22,23 Antiangiogenic mediators such as pigment epithelium-derived factor (PEDF) are reportedly lower in patients with diabetes and in patients with active PDR compared to other retinopathies.24 An interplay between both angiogenic and antiangiogenic pathways may be important in the eye at various stages of retinopathy.25 A more detailed discussion regarding the pathological angiogenesis of PDR is presented in Chapter 26.
Origin and early recognition of preretinal new vessels
The risk of PDR is greatest in eyes with severe nonproliferative diabetic retinopathy (NPDR), characterized by the presence and severity of soft exudates (cotton-wool spots), intraretinal microvascular abnormalities (IRMA, a term chosen so as to be neutral about whether these abnormal vessels represent intraretinal new vessels or dilated pre-existing vessels), venous beading, and extensive retinal hemorrhages or microaneurysms (H/MA) (Fig. 48.1). In the Diabetic Retinopathy Study (DRS), severe NPDR was defined as the presence of at least three of the above four characteristics, each generally involving at least two quadrants of the fundus. Approximately 50% of such eyes assigned to the untreated control group developed PDR within 15 months.26 Today, a quick assessment of severe or very severe NPDR can be derived from determining the extent and severity of H/MA (moderately severe in 4 quadrants), VB (definitely present in 2 or more quadrants), and IRMA (obvious in 1 or more quadrant) – the 4–2–1 rule (see Box 48.1). Any one of these findings indicates severe NPDR and two or more represent very severe NPDR. As noted above, these levels of NPDR are associated with a high likelihood of developing PDR.
The lesions characterizing severe NPDR are related to retinal capillary closure, and their frequent presence in eyes that are about to develop preretinal new vessels is one important observation linking these processes. Further evidence has been provided by the fluorescein angiographic montages of Shimizu and coworkers,27 who found that the extent of capillary closure observed using angiography increased as the severity of new vessels increased on the following four-step scale: (1) none, (2) new vessels involving the retina but sparing the disc, (3) new vessels involving the disc, and (4) neovascularization of the anterior chamber angle with neovascular glaucoma. Muraoka and Shimizu28 have provided serial fluorescein angiographic observations supporting the view that some lesions designated as IRMA or reduplication of small venules are in fact intraretinal new vessels revascularizing areas of capillary loss.
Although there is little doubt that the presence of severe NPDR is predictive of subsequent neovascularization, the characteristic intraretinal lesions are not always present when preretinal new vessels are first recognized. A possible explanation for this absence is the relatively transient nature of some of these lesions. Soft exudates usually disappear within 6–12 months.29 H/MA have a half-life of approximately 3 months.30 Blot hemorrhages and IRMA tend to disappear after extensive capillary closure, when the number of small vascular branches decreases and some small arterioles become sclerosed with a white thread-like appearance. This condition is sometimes described as “featureless retina” (Fig. 48.2B). However, in some eyes intraretinal lesions are mild, and signs of extensive capillary closure are absent when new vessels are first recognized.
New vessels may arise anywhere in the retina; however, they are most frequently seen posteriorly, within about 45 degrees of the optic disc. They are particularly common on the disc itself (Davis reported 69% of 155 eyes with PDR31; Taylor and Dobree, 73% of 86 eyes32). In the DRS, among 1377 control-group eyes with new vessels present in baseline photographs 15% had new vessels only on or within 1 disc diameter (DD) of the disc or in the vitreous cavity anterior to this area (new vessels on disc, or NVD), 40% had new vessels only outside this zone (new vessels elsewhere, or NVEs), and 45% had new vessels in both zones.33 Also from the DRS, NVE had been shown to occur most frequently in the superotemporal quadrant (field 4, 27%), followed in frequency by the inferonasal (field 7, 21%) quadrant.34 Although rare, the appearance of neovascularization arising from the perifoveal capillaries has been reported,35 and possibly may provide insight on the association of DR and perifoveal telangiectasia.36
NVD (defined as NV at or within 1 disc diameter of the diskc37) begins as fine loops or networks of vessels lying on the surface of the disc or bridging across the physiologic cup. They are usually easily identified once established, but in their earliest stages they may be overlooked, especially with the low magnification of binocular indirect ophthalmoscopy. They also may be difficult to distinguish from normal vessels in nonstereoscopic photographs or with monocular direct ophthalmoscopy. The most satisfactory examination methods are those that provide a magnified stereoscopic view, either biomicroscopy with contact or precorneal lens or stereoscopic 30-degree photography. If necessary, new vessels can be identified using fluorescein angiography where they will leak profusely, unlike normal physiologic vasculature (Fig. 48.2).
Evaluation of early NVE requires identification of the lesion and differentiation from IRMA. Binocular indirect ophthalmoscopy of the retina combined with a biomicroscopic or direct ophthalmoscopic examination of any suspicious lesions and careful review within 5 or 6 DD of the disc is a useful approach. Indirect ophthalmoscopy alone is not adequate. When new vessels or fibrous proliferation are extensive, wide-angle (45-degree or, preferably, 60-degree) photographs have the advantage of providing in one or two fields an integrated view of all or most of these lesions. For detection of early NVE, stereophotographs with a 30-degree camera are superior, and in most patients an adequate stereoscopic effect can be obtained in all the seven standard fields of the modified Airlie House classification33,37 (Fig. 48.3), even with a maximum pupillary dilation of only 4 or 5 mm. The fundus should be scanned for definite or questionable NVE outside the standard fields, particularly between and above fields 4 and 6, between and below fields 5 and 7, and temporal to fields 4 and 5. Subsequent follow-up visits are greatly facilitated by a set of 30-degree stereophotographs from the baseline examination.
Ultrawide field imaging is a newer modality allowing visualization of retinal areas previously difficult or impossible to image. A single 200-degree field taken with ultrawide field scanning laser ophthalmoscopes resulted in detection of lesions that were beyond the field of routine standard 30- and 45-degree photography.38 This capability of ultrawide field retinal imaging may enhance the detection of areas of peripheral vessel leakage and non-perfusion by flourescein angiography.39 Potentially, when validated in a broader patient population, imaging of the retinal periphery may provide prognostic and early risk stratification for visual loss in patients with diabetic retinopathy.
Natural course of proliferative diabetic retinopathy
Development and proliferation of new vessels
Initially, new vessels may be barely visible. Later, their caliber is commonly one-eighth to one-quarter that of a major retinal vein at the disc margin, and occasionally they are as large as such veins (Fig. 48.4). New vessels frequently form networks that often resemble part or all of a carriage wheel. The vessels radiate like spokes from the center of the complex to a circumferential vessel bounding its periphery (see Figs 48.2A and 48.4). New vessel networks may also be irregular in shape, without a distinct radial pattern. New vessel patches often lie over retinal veins and appear to drain into them. The superotemporal vein is involved somewhat more frequently than others.31,32 In the 1158 DRS control-group eyes that had NVE in at least one of the five photographic fields in which they were graded (fields 3 to 7 in Fig. 48.3), the number of times each field was involved was assessed. In each eye a count of one was divided equally among all fields containing NVE, and the counts for each field were totaled for all eyes. Field 4, which usually includes a major portion of the superotemporal vein, had a score of 308 (27% of 1158), whereas other scores ranged from 194 (17%) for field 5 to 242 (21%) for field 7.2
At times new vessels grow for several DD across the retina without forming prominent networks. The new vessels appear much like normal retinal vessels but are easily recognized as new vessels because of their unique capability of crossing both arterioles and veins in the underlying retina (Figs 48.5. 48.6). New vessels of this type commonly arise on the disc and are often accompanied during their actively growing phase by mild-to-moderate thickening (presumably edema) of the disc and surrounding retina (Fig. 48.7). This appearance is similar to typical cases of diabetic papillopathy,40 in which all or most of the dilated small vessels on and adjacent to the disc are intraretinal and characteristically do not leak on fluorescein angiography.
The growth rate of new vessels is extremely variable. In some patients a patch of vessels may show little change over many months, whereas in others a definite increase may be seen in 1–2 weeks. Early in their evolution new vessels appear bare, but later, delicate white fibrous tissue usually becomes visible adjacent to them. The common clinical convention of referring to such tissue as “fibrous” is adhered to in this chapter, even though it has been shown to contain both fibrocytes and glial cells.41,42 New vessels characteristically follow a cycle of proliferation followed by partial or complete regression.31,43 Regression of a wheel-shaped net of new vessels typically begins with a decrease in the number and caliber of the vessels at the center of the patch, followed by their partial replacement with fibrous tissue. Simultaneously, the peripheral vessels tend to become more narrow, although they may still be growing in length and the patch may still be enlarging (Fig. 48.4). At times, regressing new vessels appear to become sheathed. The width of the sheath, which presumably represents opacification and thickening of the vessel wall, increases until only a network of white lines without visible blood columns remains (Fig. 48.8). At times certain new vessels seem to become preferential channels, enlarging while adjacent vessels regress and disappear. Fresh, active new vessels are commonly seen emerging from the edges of partially regressed patches, and new vessels are frequently seen at different stages of development in different areas of the same eye. Early in their evolution, the fibrous components of fibrovascular proliferations tend to be translucent and are easily underestimated. Subsequently, with increasing growth, contraction, or separation from the retina, they become more prominent. If contraction of the vitreous and fibrovascular proliferations does not occur, new vessels may pass through all the stages described here without causing any visual symptoms. Concurrently, a decrease in intraretinal lesions and in the caliber of major retinal vessels may occur as retinopathy enters the quiescent stage. Occasionally, new vessels appear to regress completely, leaving no trace of their previous presence.44
Based on the findings of the DRS, the development of PDR with high-risk characteristics places the patient at an increased risk for visual loss and generally requires prompt laser treatment. PDR with high-risk characteristics is defined by one or more of the following lesions: (1) NVD that is approximately one-quarter to one-third disc area or more in size (i.e., greater than or equal to NVD in standard photograph 10A); (2) any amount of NVD if fresh vitreous or preretinal hemorrhage is present; or (3) NVE greater than or equal to one-half disc area in size if fresh vitreous or preretinal hemorrhage is present Therefore, attention must be paid to the presence, location, and severity of new vessels, as well as the presence or absence of preretinal or vitreous hemorrhages.2
Contraction of the vitreous and fibrovascular proliferation
Before the beginning of posterior vitreous detachment, new vessels are usually asymptomatic.31,43 Small hemorrhages in the posterior vitreous are occasionally seen near the growing ends of the new vessels, but they usually remain subhyaloid or hang suspended in the most posterior portion of the vitreous without becoming apparent to the patient. When symptomatic vitreous hemorrhages occur, some evidence of localized posterior vitreous detachment can usually be found. When only a small area of the posterior vitreous surface is detached, it appears flat and very close to the retina, but as detachment becomes more extensive, this surface moves forward and assumes a curved contour more or less parallel to the retina and about 0.5–2 DD anterior to it. This otherwise smoothly curved surface is held posteriorly by vitreoretinal adhesions at the sites of new vessels. The new vessels in turn tend to be pulled forward in these same areas. Vitreous strands and opacities can usually be seen anterior to the posterior vitreous surface, whereas posteriorly the vitreous cavity is optically empty or contains red blood cells.31,45 The principal force pulling the posterior vitreous surface forward usually appears to be the forward vector resulting from contraction of this surface and the fibrovascular proliferation growing along it. In explaining this process to students and patients, it helps to use the analogy of a bowl lined with a piece of cloth attached to the rim of the bowl: if the cloth shrinks, it eventually becomes tightly stretched across the top of the bowl.
The thickness of the posterior vitreous surface varies, as indicated by three different appearances. Immediately adjacent to the site of new vessels, the surface is often thick enough to be seen easily with the ophthalmoscope. Presumably this increased opacity is due to proliferation of fibrous tissue along the posterior vitreous surface. In other areas some distance from any visible new vessels, the surface is also sometimes thick enough to be detected ophthalmoscopically or in stereoscopic fundus photographs. In these areas the surface is usually somewhat shiny, with thinner and thicker areas alternating to give a “Swiss cheese” effect but without actual holes; presumably a thin layer of fibrous tissue is also present here. In still other areas the posterior vitreous surface is so thin that it can be appreciated only by mentally integrating many separate slit-lamp sections. Only the portion directly illuminated by the slit beam is visible, and the impression of a continuous surface is gained by watching the slit beam glide along smoothly over the surface as the slit lamp is moved. Frequently in the same eye all these various appearances can be seen in different areas of the same surface, the general course of the surface continuing without change as its thickness varies (Fig. 48.9).
Posterior vitreous detachment usually begins near the posterior pole, the most common locations being the region of the superotemporal vessels, temporal to the macula, and above or below the disc.31 Detachment often spreads fairly rapidly (within hours, days, or weeks) to the periphery of the quadrant in which it begins, unless such spread is impeded by vitreoretinal adhesions associated with patches of new vessels. Extension circumferentially into other quadrants of the fundus tends to be slower, sometimes requiring months or years to reach completion. Detachment of the vitreous from the disc is usually prevented by adhesions between the vitreous and fibrovascular proliferations arising there. Vitreous detachment is not a smoothly progressive process. It occurs in abrupt steps, usually halting whenever its advancing edge meets a patch of active or regressed new vessels. If contraction continues, the patch is pulled forward, with or without the underlying retina, and vitreous detachment spreads beyond it. At times the peripheral spread of posterior vitreous detachment is halted temporarily by invisible adhesions to the retina in areas where no new vessels are present. These adhesions are indicated by a subtle linear elevation of the inner surface of the retina at the junction of posteriorly detached and anteriorly attached vitreous. After several weeks or months, vitreous detachment usually spreads farther peripherally, and the subtle retinal fold flattens.
Traction exerted on the new vessels appears to be a factor contributing to the recurrent vitreous hemorrhages that often coincide with extension of vitreous detachment. Hemorrhages also occur independently, sometimes apparently in relation to bouts of severe coughing or vomiting and occasionally at the time of insulin reactions. More often they occur during sleep and are unrelated to any obvious factor.46,47 Blood in the fluid vitreous posterior to the detached vitreous framework usually absorbs within weeks or several months, retaining its red color until absorbed. Hemorrhage in the formed vitreous tends to lose its red color and become white before absorption is complete. Absorption of a large hemorrhage from the formed vitreous is usually slow, requiring many months.
The arrangement and movement of blood in the posterior fluid vitreous often make it possible to define the limits of posterior vitreous detachment ophthalmoscopically.31,48 In areas of vitreous detachment, the presence of fresh blood in the posterior fluid vitreous obscures fundus details, distinguishing these areas from adjacent areas in which the vitreous remains attached and details of the retina are clear. In the upper quadrants of the fundus, blood tends to become deposited in thin meridional streaks on the detached posterior vitreous surface, identifying its position. Inferiorly, blood pools between the detached vitreous and attached retina, outlining the inferior extent of vitreous detachment and often forming a fluid-level or “boat-shaped” hemorrhage. At times, even when posterior vitreous detachment cannot definitely be identified with slit lamp and contact lens, a thin, curving line of subhyaloid hemorrhage parallel to and behind the inferior equator can be seen, presumably marking the lower edge of an area of vitreous detachment. Occasionally the posterior vitreous surface can be traced across the macula on slit-lamp examination, but usually its continuity is lost in this region. In some of these cases a round or oval hole with sharp edges can be detected in the posterior vitreous surface, occupying an area 2–4 DD wide in the posterior pole. The posterior vitreous surface in this area appears broken, with solid vitreous protruding back through the hole and coming into contact with the retina. At times the surface of a bulging mushroom of vitreous can be seen extending posteriorly through such a hole, occasionally with hemorrhage suspended within its lower part (Fig. 48.10).
Retinal distortion and tractional detachment
With contraction of an extensive sheet of fibrovascular proliferations, distortion or displacement (“dragging”) of the macula may occur.49 In some cases the central, more intensely pigmented area of the retinal pigment epithelium (RPE) appears to be dragged with the neurosensory macula toward the major focus of contracted tissue, whereas in other cases only the neurosensory macula appears displaced. Since the most common site of extensive fibrovascular proliferations is on and near the disc, the macula is usually dragged nasally and often also somewhat vertically (Figs 48.11, 48.12).
Contraction of vitreous or areas of fibrovascular proliferation may also lead to retinal detachment. This retinal detachment may be limited to avulsion of a retinal vessel, usually a vein, sometimes accompanied by vitreous hemorrhage. Alternatively, a relatively thin fold of retina may become elevated, with only a narrow zone of retinal detachment adjacent to its base, sometimes outlined by a pigmented demarcation line. In other cases retinal detachment may be more extensive, but the concave shape that is typical of traction detachment is generally maintained. At times, small, apparently full-thickness retinal holes may be seen near the proliferation; these sometimes, but not always, lead to rhegmatogenous detachment. When such detachment does occur, it tends to have a flat or convex anterior surface and be more extensive, often reaching the ora serrata. The occurrence and severity of retinal detachment are influenced by the timing and degree of shrinkage of the vitreous and fibrovascular proliferations and by the type, extent, and location of the new vessels responsible for vitreoretinal adhesions. Extensive nets of large-caliber new vessels accompanied by heavy fibrous tissue produce broad, tight vitreoretinal adhesions. Contraction of such proliferations is often followed by extensive retinal detachment (Fig. 48.12). New vessels with little accompanying fibrous tissue tend to produce less extensive vitreoretinal adhesions and less risk of retinal detachment, particularly when posterior vitreous detachment begins soon after the onset of neovascularization (Fig. 48.13). At times, new vessels that extend for a considerable distance along the surface of the retina appear to be adherent to the retina only at their sites of origin and to the vitreous only near their distal ends. In this case, the posterior vitreous surface can pull away from the retina by a distance equal to the length of the vessels before exerting traction on the retina. When new vessels are confined to the surface of the disc, vitreous detachment can reach completion without producing traction on the retina, since there are no vitreoretinal adhesions. Retinal detachment does not occur in such eyes, but recurrent vitreous hemorrhage from the new vessels is common.
Involutional or “Quiescent” Proliferative Diabetic Retinopathy
DR ultimately reaches an involutional stage wherein the retinopathy has “burned-out” and is termed “quiescent.” At this stage vitreous contraction has reached completion and the vitreous is detached from all areas of the retina except where vitreoretinal adhesions associated with new vessels prevent such detachment.31,43,50,51 Vitreous hemorrhages decrease in frequency and severity and may stop entirely, although many months may elapse before substantial vitreous clearing occurs. Some degree of retinal detachment may be present at this stage. If the detachment is localized and the macula remains intact, visual acuity may be good. However, dragging or distortion of the macula or long-standing macular edema can lead to substantial reduction in vision. In some cases, retinal detachment involves the entire posterior pole, with resultant severe loss of vision. Although spontaneous partial reattachment occasionally occurs, if the macula has been detached for months or years, usually little significant return of vision occurs.
Relationship of proliferative diabetic retinopathy to type and duration of diabetes
In a population-based stereophotographic study carried out by Klein and coworkers,52 the prevalence of PDR in insulin-taking patients younger than 30 at diagnosis (exclusively or mainly type 1) was near zero when duration of diabetes was less than 10 years and then rose rapidly to about 50% in persons with 20 years or more of diabetes. In an older-onset (30 years or more) insulin-taking group, which included both diabetes types, prevalence of PDR rose fairly steadily, from 2% in persons with less than 5 years of diabetes to about 25% in those with 20 years or more. In the older-onset, noninsulin-taking (type 2) group, prevalence of PDR increased only slightly with duration, from less than 5% before 20 years to about 5% thereafter (Fig. 48.14).53 Among patients with PDR, its severity did not appear to differ between the younger-onset and the combined older-onset groups. In each case, in the worse eye about 25% of patients had DRS high-risk characteristics and 15% had retinopathy severity ungradable because of extensive vitreous hemorrhage, phthisis bulbi, or enucleation secondary to complications of DR.7 In patients with PDR, macular edema was more common in the combined older-onset group with retinal thickening or scars of previous focal photocoagulation present in at least one eye in about 45% (versus 30% in the younger-onset group).54
The Diabetic Retinopathy Vitrectomy Study (DRVS) found a substantial variation in severity of PDR by diabetes type among persons with vitreous hemorrhage severe enough to reduce visual acuity to 5/200 or less for a period of at least 1 month.55–58 In this study the severity of new vessels, fibrous proliferation, and vitreoretinal adhesions decreased significantly as diabetes type shifted from type 1 to type 2 (Table 48.1).
Table 48.1 Percentage of Diabetic Retinopathy Vitrectomy Study (DRVS) group H eyes assigned to early vitrectomy with specified severity level of new vessels, fibrous proliferations, and vitreoretinal adhesions, by diabetes type
Diabetes with onset after age 30 is more common than the younger-onset type, and in clinical practice PDR is seen with about equal frequency in the younger- and older-onset groups. Klein et al.52,53 estimated that in the population they surveyed, 43% of patients with PDR were in the younger-onset group, 42% were in the older-onset insulin-taking group, and 15% were in the noninsulin-taking group. In the DRS, in which more than 90% of the 1742 patients examined had PDR in at least one eye, 44% were classified as juvenile-onset (younger than 20 years at diagnosis and taking insulin at entry into the study); 28% as adult-onset, possibly insulin-dependent (age 20 years or older at diagnosis, not overweight, and taking insulin); and 26% as classic adult-onset (mild symptomatic or asymptomatic onset at age 20 years or older and either overweight or not taking insulin at study entry). The remaining 2% were not classifiable.33 Aiello and coworkers59 described the distribution of age at diabetes diagnosis among 244 patients with PDR at the Joslin Clinic during a 5-month period: diagnosis age less than 20 years, 53%; 20–39 years, 25%; and 40 years or older, 22%.
In a comprehensive meta-analysis including 28 prospective interventional or observational studies comprising 27 120 diabetic patients with at least 10 years of follow-up, lower rates of progression to PDR and severe visual loss were observed in those more recently diagnosed with diabetes.10 The 4-year risk of progression to PDR and severe visual loss was substantially lower among participants in 1986–2008 (2.6% and 3.2%) than in 1975–1985 (19.5% and 9.7%). At 10 years, similar patterns were observed with participants in 1986–2008 studies having lower proportions of PDR and NPDR at all time points than participants in 1975–1985 studies. National population-based estimates have reflected this trend of a reduction in the prevalence of PDR, presumably reflecting improved glycemic and systemic control as well as earlier detection of retinal complications.60 These trends in the reduction of PDR and visual loss are discussed in greater detail in Chapter 45 (The epidemiology of diabetic retinopathy).
Proliferative diabetic retinopathy and blood glucose control
The results from the Diabetes Control and Complications Trial (DCCT)/Epidemiology of Diabetes Interventions and Complications (EDIC) and the United Kingdom Prospective Diabetes Study (UKPDS) have established the benefit of intensive blood glucose control in reducing the risk for DR in both type 1 and type 2 patients.61–70 These large multicenter trials demonstrated conclusively that the long-term risks for the development and progression of DR can be reduced dramatically by improving blood glucose control with intensive treatment.61–70
Additional evidence that better glycemic control in patients with severe NPDR or early PDR reduces their risk of further progression is provided by Early Treatment Diabetic Retinopathy Study (ETDRS) multivariable analyses of risk factors for progression to high-risk PDR. HbA1c at baseline was a strong risk factor. Patients with HbA1c >12% had the highest risk of progression and patients with HbA1c <8.3% had the lowest risk of progression (OR 1.59 vs 1.00, P <0.0001).71 Even in the lowest A1c category, the 5-year rate of developing high-risk PDR from severe NPDR was high (50%). These data suggest that the benefits of better control continue to be manifest even once severe NPDR or PDR has developed; however, they do address the effects, beneficial or harmful (see below), of improving control at this stage.
Early worsening of retinopathy with improved glycemic control
Soon after HbA1c assays, home blood glucose monitoring and continuous subcutaneous insulin infusion became widely available, several small clinical trials and case series reported the frequent occurrence of unexpected worsening of DR72–75 in the first 3–12 months following the initiation of intensive insulin therapy (termed “early worsening”).73,76–78 In most of these early trials the patients enrolled had no more than mild-to-moderate NPDR at baseline and the early worsening, when it occurred, was usually mild (development of cotton-wool spots and/or IRMA) and transient. In some reports, however, when glycemic control was very poor and/or retinopathy more severe at baseline, some eyes developed severe PDR and/or macular edema and substantial visual loss.63,72–75
In the DCCT, cotton-wool spots or IRMA, or both, developed in only 1% of 348 patients entering the trial with no retinopathy. This proportion increased to 48% in the 60 patients with mild nonproliferative retinopathy, defined as the presence of microaneurysms plus mild retinal hemorrhages and/or hard exudates. Recovery was frequent and at the 4-year follow-up visit progression from baseline on the ETDRS scale was about the same in intensive treatment group eyes that had experienced early worsening as in conventional treatment group eyes that had not (1.3 vs 1.0 steps). Clinically important early worsening (defined as development of PDR, severe NPDR, or clinically significant macular edema) was not observed in patients with no retinopathy or with microaneurysms involving only one eye, but it occurred in 6 of the 32 patients with moderate NPDR. DCCT patients were followed closely, and early worsening did not lead to serious visual loss, but DCCT findings support the conclusion that early worsening may be more common and more sight-threatening in patients with more severe retinopathy and/or very poor glycemic control. For this reason, patients with advanced nonproliferative or active proliferative DR should be monitored closely before and for several months after initiation of intensive insulin treatment.70,72,75,79 Panretinal photocoagulation prior to initiation of such treatment may be considered when factors suggest a particular need to protect against advancing severe retinopathy. Such considerations include very severe NPDR or active PDR, long-standing very poor glycemic control and high likelihood of suboptimal follow-up.72 The most important risk factors for early worsening were higher baseline HbA1c and greater reduction of HbA1c after enrollment. Possible mechanisms include72,75 alterations in retinal blood flow, decreased autoregulation of the retinal circulation, transient ischemia owing to a decrease in nutrient substrate, and insulin-induced changes in retinal homeostasis that lead to an increase in growth factors such as VEGF.80–82 Because the short- and long-term benefits of improved glycemic control in reducing the risk of retinopathy progression are remarkable67 and because treatments for sight-threatening retinopathy are highly effective in preventing visual loss, intensive glycemic control should not be discouraged for fear of retinopathy progression.69
Absence of proliferative diabetic retinopathy in individuals with diabetes of extreme duration
Despite the nearly universal development of some degree of retinopathy in people with diabetes given sufficient time, the development of PDR plateaus at approximately 60%. This observation has generated significant research interest, as it suggests that there may be protective mechanisms that may delay or prevent the progression to PDR. There are published reports on two unique cohorts of type 1 patients with more than 50 years of type 1 diabetes. The Golden Years cohort from the United Kingdom was noted to have characteristic normal body mass, low insulin dose, a favorable lipid profile, and a positive family history of longevity consistent with possibly genetically determined favorable outcomes.83 The Joslin 50-year Medalist cohort has been characterized for all four major diabetic vascular complications of retinopathy, nephropathy, neuropathy, and cardiovascular disease. The 50-Year Medalist Study84,85 has demonstrated that substantial proportions of individuals may survive diabetes duration of 50 years or more and remain free of advanced diabetic vasculopathy including PDR (49.4%). Longitudinal data from a subgroup of 97 Medalists followed for an average of 20.6 years and 39.4 visits suggests that retinopathy worsening occurs almost entirely within the first two decades of follow-up and eyes that do not develop PDR have a slower rate of retinopathy progression. These findings strongly suggest the existence of a subgroup of individuals who develop early protection against the long-term adverse effects of hyperglycemia. Furthermore, despite multiple studies that have strongly associated worse glycemic control, hypertension, and hyperlipidemia with more severe diabetic retinopathy or diabetic macular edema in patients with shorter duration diabetes, no relationship has been found between these factors and PDR status in the Medalists. Instead, initial findings suggest that specific combinations of advanced glycation endproducts may be associated with increased risk for (carboxyethyl-lysine and pentosidine) or protection from (carboxymethyl-lysine and fructose-lysine) PDR in this unique cohort. Ongoing studies in the Medalists and other populations with extremely long duration of diabetes may yield additional insights into protective mechanisms against PDR development, including novel genetic, biochemical, and physiologic factors.
Systemic medications and proliferative diabetic retinopathy
Systemic medications are often used in the setting of diabetes mellitus to attain optimal glycemic control and treat coexisting conditions. These drugs can have beneficial or deleterious effects on the onset or progression of diabetic eye disease. There is mounting evidence that oral systemic medications can reduce microvascular complications possibly though mechanisms other than their effect on glycemic control, blood pressure and lipid lowering. The results of clinical trials on glycemic control (DCCT,61,65 EDIC,69,70 UKPDS,62,67,68 ACCORD,86 ADVANCE87), lipid-lowering medications (ACCORD-EYE,88 FIELD89) and angiotensin-converting enzyme inhibitors (EURODIAB,90 EUCLID,90 ADVANCE86) angiotensin II type 1-receptor blockers (DIRECT91,92, RASS93) on retinopathy progression are discussed in detail in Chapter 45 (The epidemiology of diabetic retinopathy) and Chapter 47 (Nonproliferative diabetic retinopathy and diabetic macular edema).
Evidence to support the rationale of using systemically active therapeutic agents to prevent or limit local microvascular complications such as PDR is also growing. Thiazolidinediones are a class of oral hypoglycemic agents used in the treatment of type 2 diabetes that activate the peroxisome proliferator-activated receptor (PPAR) γ – a transcription factor known to regulate the expression of genes primarily located in adipose tissue, but also present in other tissues such as the retina.94 The thiazolidinedione rosiglitazone has been reported to delay the onset of PDR, possibly because of antiangiogenic effects mediated by PPARγ agonist activity.95,96 Shen and colleagues95 performed a longitudinal medical record review of 124 patients treated with rosiglitazone and 158 patients not receiving rosiglitazone as controls, who were matched by baseline characteristics including level of HbA1c. Among patients with severe NPDR receiving rosiglitazone, the relative risk of progression to PDR at 3 years was reduced by 59% (P = 0.045), and this effect continued over 5 years of follow-up. Furthermore, at 5 years of follow-up, a significantly smaller proportion of patients in the rosiglitazone group experienced a decline of 3 or more lines in visual acuity (0.5% vs 38.0%; P = 0.03). No difference was found in the incidence of DME or CSME between the groups (P = 0.28). Initial case series and a prospective cohort-based electronic medical record-based review have reported the association of thiazolidinedione use and diabetic macular edema (OR 2.6; 95% CI 2.4–3.0). However, data from the largest clinical trial study to date to evaluate an association between thiazolidinedione exposure and DME in patients with type 2 diabetes demonstrated no such association. Thus, it appears that DME can occur at least sporadically with thiazolidinedione use, although this is relatively rare.
Other risk factors for proliferative diabetic retinopathy
Most studies seeking to identify risk factors for the development of PDR begin with patients who have various levels of NPDR or no visible retinopathy at all, and make comparisons of baseline factors between those who do and do not develop PDR. As expected, the significant risk factors for the development of PDR include increasing NPDR severity, decreased visual acuity, and elevated HbAlc. Additional risk factors included the presence of diabetic neuropathy, decreased hematocrit, increased serum triglyceride, and decreased plasma albumin.71 However, it should be noted that if the risk factors for progression from severe NPDR to PDR differ substantially from those mediating onset or progression of earlier NPDR previous studies may not have readily identified these differences.
The association of elevated serum lipids with increased risk of progression to high-risk PDR, as well as their association with increased hard exudates and decreased visual acuity,97 provide additional motivation for lowering the frequently elevated lipid levels observed in diabetic patients. Data from the ACCORD-EYE study show that DR progression rates were reduced from 10.2% with placebo to 6.5% with fenofibrate therapy for dyslipidemia (adjusted OR 0.60; 95% CI 0.42–0.87; P = 0.006).88 Severe anemia is a less frequently encountered problem in diabetic patients, but its association with increased risk of severe retinopathy has been suggested by ETDRS analyses and three other reports.71 Hypertension was not identified as a risk factor for development of high-risk PDR in the ETDRS, while findings in previous studies have been variable.7,71 In the UKPDS, patients with hypertension were randomized between more- and less-intensive regimens of blood pressure control, and retinopathy progression was significantly less common in the former, as was the incidence of photocoagulation and of a 3 or more line decrease in visual acuity. Risk reductions after 7.5 years ranged from 35% to 45% for these outcomes. Progression to PDR was too infrequent for meaningful analysis. A more detailed discussion of the epidemiology and risk factors for DR is presented in Chapter 45.
Management of proliferative diabetic retinopathy
Familiarity with the natural course of PDR suggests two principal therapeutic approaches: first, to discourage the proliferation of new vessels, and, second, to prevent or relieve the effects of contraction of the posterior vitreous surface and fibrovascular proliferation. This section deals mainly with the first aim as the second is discussed in Chapter 111 (Surgery for proliferative diabetic retinopathy). The cornerstone of diabetes eye care is the maintenance of intensive glycemic control which is remarkably effective in reducing the risk of onset and progression of DR and the development of PDR.61–70 Once active proliferative changes have begun, glycemic control alone is usually insufficient. A variety of other approaches have been attempted including pituitary ablation, which was supplanted by laser photocoagulation as the treatment of choice for more than four decades now. In addition, new therapeutic interventions are now on the horizon. The following section discusses these past, present, and future approaches.
Pituitary ablation
Building on the fundamental discovery of Biasotti and Houssay98 and that hypophysectomy reduced the severity of diabetes in pancreatectomized dogs, Luft and corworkers99 carried out hypophysectomy in the hope of ameliorating the vascular complications of diabetes. Further impetus was provided by Poulsen’s report100,101 of remission of DR in a woman with postpartum anterior pituitary insufficiency (Sheehan syndrome). Over the next 25 years, various types of pituitary suppression were used, ranging from external irradiation to transfrontal hypophysectomy, and consensus developed among advocates of these procedures that complete or nearly complete suppression of anterior pituitary function (pituitary ablation) produced rapid improvement in eyes with the intraretinal lesions characteristic of severe NPDR and actively growing new vessels not yet accompanied by extensive fibrous proliferations. Although only two randomized trials have been reported,102 both small and neither in itself compelling, the weight of evidence supports the strongly held opinion of those most experienced with this procedure that it was beneficial. Particularly persuasive are comparisons between patients in whom transsphenoidal implantation of radioactive yttrium was followed by complete or nearly complete anterior pituitary suppression and similar patients in whom little or no suppression was achieved. Substantially better outcome was observed in the former group.103 Additional support is provided by a nonrandomized comparison of eyes with very extensive new vessels and IRMA, in which outcome was better in the eyes of patients undergoing pituitary ablation than in similar eyes receiving photocoagulation or no treatment.104 Pituitary ablation is now primarily of historical interest because photocoagulation is more effective and is free of the many substantial disadvantages of inducing and living in the hypopituitary state with concomitant diabetes (e.g., operative and immediate postoperative risks, increased susceptibility to severe insulin reactions, need for continuing replacement of adrenal corticosteroids, sterility).
The favorable effect of pituitary ablation on retinopathy is thought to be mediated by suppression of growth hormone activity and effects on insulin-like growth factor 1 (IGF-1).105 Daily subcutaneous injections of a genetically engineered growth hormone receptor antagonist, pegvisomant, have been given for 3 months in 25 patients with non-high-risk PDR. Regression of new vessels did not occur in any patient, although the serum level of insulin-like growth factor 1 (IGF-1), a growth factor whose secretion is stimulated by growth hormone, did decrease an average of 55% compared to baseline levels.106 In a small randomized clinical trial, multiple daily subcutaneous injections of octreotide, a somatostatin analog that inhibits both growth hormone and insulin-like growth factor, were given to 11 patients with severe NPDR or non-high-risk PDR. During 15 months’ follow-up, 1 out of 22 of these patients’ eyes required scatter laser photocoagulation compared to 9 out of 24 eyes of 12 patients randomly assigned to an untreated control group.107 Unfortunately, larger clinical trials of somatostatin analogs were not found to be effective.
Early laser trials
Early observations noted that certain ocular conditions seemed to prevent severe diabetic retinopathy. In addition, as many as 10% of patients experienced spontaneous resolution of PDR.51 In these eyes the retinopathy becomes stable, the hemorrhages resolve, the retinal vasculature becomes quiescent, the proliferating tissue thins, the retinal veins lose their distended appearance, the retinal arteries become small and attenuated and many obliterated vessel branches are observed. This appearance of the retina is strikingly similar to eyes that have undergone chorioretinal scarring, optic atrophy, high myopia, retinitis pigmentosa, and the end stage of the desired outcome following pituitary ablation.108,109 The initial use of the xenon arc photocoagulator developed by Meyer–Schwickerath110 in the treatment of PDR involved direct treatment of new vessels on the surface of the retina, particularly those that appeared to be the source of vitreous hemorrhage.111–113 Large, slow, moderately intense burns were used, turning the retina white adjacent to the new vessels and sometimes causing them to narrow and the flow within them to slow. These effects were the result of heat generated when light was absorbed by the retinal pigment epithelium (RPE) or by hemorrhage within the retina or on its surface. Direct destruction of new vessels required heavy burns, which usually involved the full thickness of the retina and often led to nerve fiber bundle field defects, particularly if hemorrhages were present in or on the retina. When new vessels were located some distance from the RPE, either in the vitreous or on the optic disc, they could not be treated directly with the xenon arc photocoagulator because it was not possible to concentrate enough energy in a short enough time to coagulate the rapidly flowing blood within them. The hope that this effect would be possible with the narrow, intense, blue–green beam of the argon laser was part of the rationale for its development.
Before the argon laser became widely available, and before recognition of the tendency for NVD and elevated NVE to regrow after apparently successful direct treatment, the novel concept of panretinal treatment described above began to evolve. Based on observations of remarkable asymmetry of retinopathy favoring the involved eye in diabetic patients who had unilateral disseminated chorioretinal scarring, high myopia, or optic atrophy, Beetham and Aiello began a study in which ruby laser burns were scattered across the retina from the posterior pole to the midperiphery.109,114,115 The hope was that the induced scarring might have a distant effect across the retina and promote regression of new vessels and diminution of retinal edema and vascular congestion.109,116,117 The long wavelength and very brief exposure time of the ruby laser limited burns mainly to the outer layers of the retina, without immediately visible effects in new vessels on its surface.
The mechanisms by which panretinal photocoagulation mediates its remarkable benefit are not fully understood. Several mechanisms have been proposed and all may contribute toward the beneficial effect. Ischemic retina, which produces growth factors, is destroyed, thus reducing the angiogenic stimulus. Another possibility is that retinal cells may produce growth-inhibiting factors or reduce production of growth-promoting factors in response to photocoagulation injury. Such factors have not been shown to be of importance in vivo to date.118,119 It is thought that the primary mediating factor is an increase in oxygenation from the choroid to the inner retina that occurs through the laser scars due to the thinning of the retina in the treated area.120–124 Indeed, retinal blood flow decreases and the autoregulatory response to breathing pure oxygen improves following scatter photocoagulation, as might be expected if more oxygen reached the inner retina from the choroid.125,126 Furthermore, direct measurements of increased vitreous oxygen have been made using intraocular microelectrodes.127The oxygen concentration of the vitreous is much higher overlying the areas of laser burns than over the untreated retina. Regardless of the relative contributions of these mechanisms, the remarkable efficacy of panretinal photocoagulation in the treatment of PDR has been thoroughly documented in multiple randomized clinical trials.
Panretinal photocoagulation
The initial reports concerning photocoagulation suffered from small numbers of patients, brief periods of follow-up, or lack of a randomly selected control group.119 Randomized clinical trials were needed to evaluate the possible benefits and risks of this treatment objectively. Two collaborative studies were initiated in the early 1970s: the British multicenter trial using xenon arc photocoagulation128 and the National Eye Institute’s Diabetic Retinopathy Study (DRS), which compared xenon arc and argon laser photocoagulation to no photocoagulation in patients with PDR.129 The DRS provided the initial evidence to establish the safety and efficacy of modern panretinal (scatter) photocoagulation (PRP).
The DRS conclusively demonstrated that PRP significantly reduces the risk of severe visual loss (SVL) from PDR, particularly when high-risk PDR is present.1,26,130 Patients entering the DRS had PDR in at least one eye or severe NPDR in both eyes. Visual acuity of 20/100 or better was present in each eye. Each patient was randomly assigned to either the argon or xenon treatment group. One eye was randomly assigned to photocoagulation treatment and the other to indefinite deferral of treatment (i.e., no treatment ever), unless evidence that treatment was beneficial resulted in a change of study protocol. Patients were followed at 4-month intervals according to a protocol that provided for measurement of best-corrected visual acuity under standard lighting conditions, with separate charts for each eye. The visual acuity examiners did not know the identity of the treated eye or type of treatment and attempted to reduce patient bias by urging the patient to read as far down the chart as possible with each eye, guessing at letters until more than one in a line was missed.1
DRS treatment techniques are summarized in Table 48.2. Both techniques included scatter treatment with burns spaced about one-half to one burn-width apart, extending from the posterior pole to the equator and often completed in a single sitting. The argon treatment technique specified 800–1600 500 µm scatter burns of 0.1 second duration and direct treatment of new vessels on the disc and elsewhere, whether flat or elevated. Direct treatment was also applied to microaneurysms or other lesions thought to be causing macular edema. Follow-up treatment was applied as needed at 4-month intervals. The xenon technique was similar, but burns were fewer, of longer duration, and stronger. Direct treatment was not applied to elevated new vessels or those on the surface of the disc in the xenon treated group.
Table 48.2 Diabetic retinopathy study photocoagulation techniques
Argon laser | Xenon arc | |
---|---|---|
Scatter treatment | ||
No. of burns | 800–1600 (500 µm) | 400–800 (3 degrees) |
or 500–1000 | or 200–400 | |
(1000 µm) | (4.5 degrees) | |
Exposure time | 0.1 s | Not specified |
Direct treatment* | ||
Surface NVE | + | + |
Elevated NVE | + | − |
NVD | + | − |
Macular edema | + | + |
Follow-up treatment | + | + |
* NVE, new vessels elsewhere (more than 1 disc diameter [DD] from the disc); NVD, new vessels on or within 1 DD of the disc.
(Reproduced with permission from Diabetic Retinopathy Study Research Group. Photocoagulation treatment of proliferative diabetic retinopathy: clinical application of Diabetic Retinopathy Study (DRS) findings. DRS report number 8. Ophthalmology 1981; 88:583–600. Copyright © 1981 American Academy of Ophthalmology.)
The principal outcome of the DRS was visual acuity of <5/200 at each of two consecutively completed follow-up visits, scheduled at least 4-months apart (termed severe visual loss). Visual acuity of <5/200 was chosen as the level at which vision becomes too poor to be useful for walking about or for other self-care activities. The requirement of two consecutive visits was included because the rate of recovery to better visual acuity after a single visit at the <5/200 level was 29% in the control group and 49% in the treated group whereas after two visits it was 12% and 29%, respectively, and after three visits, 8% and 21%.1 Because recovery was somewhat more common in treated eyes, the chosen endpoint tends to underestimate the treatment benefit.
Table 48.3 presents 2-year cumulative rates of severe visual loss in eyes grouped by baseline retinopathy severity and treatment assignment.2 For severe visual loss to be present at the 2-year visit, visual acuity had to be <5/200 no later than the 20-month visit. For all eyes in the untreated control group, the risk of severe visual loss within 2 years was 15.9%, and this risk was reduced to 6.4% by treatment. The risk was greatest in group J (36.9% in the control group). These eyes had preretinal or vitreous hemorrhage and NVD exceeding those in standard photograph 10A of the modified Airlie House classification (Fig. 48.15). The risk appeared somewhat lower for eyes with NVD of this severity without hemorrhage (group I, 26.2% in the control group). Similar risks (25.6 and 29.7%, respectively) were observed for untreated eyes in groups H and F, eyes with vitreous or preretinal hemorrhage, and less severe new vessels. Eyes in these four groups were referred to in the DRS as eyes with high-risk characteristics or, alternatively, eyes with three or four new vessel-vitreous hemorrhage (NV-VH) risk factors, these factors being: (1) new vessels present; (2) new vessels located on or within 1 DD of the disc (NVD); (3) new vessels moderate to severe (NVD equaling or exceeding those in standard photograph 10A or, for eyes without NVD, NVE equaling or exceeding one-half disc area in at least one photographic field); and (4) vitreous or preretinal hemorrhage (or both) present. In counting risk factors, the presence and severity of NVE were considered only in eyes without NVD because a subgroup analysis indicated that in eyes with NVD the presence of moderate or severe NVE did not further increase the risk of severe visual loss.2 In the remaining groups (A through E and G), the risk without treatment varied from 3.6 to 10.5%. Treatment reduced the rate of severe visual loss in each group, most impressively in groups F through J. Since it appeared that a small permanent reduction in visual acuity might occur in 10–20% of treated eyes, the DRS investigators concluded in 1976 that prompt photocoagulation treatment was usually desirable for eyes with high-risk characteristics. The protocol was therefore modified to allow treatment of eyes originally assigned to the untreated control group, if they had high-risk characteristics at the time or developed them subsequently.1
Table 48.3 Cumulative 2-year rates of severe visual loss in eyes grouped by baseline retinopathy severity and treatment assignment
In Table 48.4 the retinopathy severity groups presented in Table 48.3 have been combined, and observations from follow-up visits completed after the 1976 protocol change have been included.1 Forty-three percent of the 2-year visits and all the 4-year visits included in this analysis were carried out after the 1976 protocol change. At the 2-year visit, 12% of control-group eyes had been treated, and by the 4-year visit 35% had been treated. All eyes were classified in the group to which they were originally randomly assigned, without reference to treatment of control-group eyes. In the control group the 2-year risk of severe visual loss increased from 3.2% in eyes with NPDR to 7% in eyes with PDR without high-risk characteristics, and to 26.2% in eyes with high-risk characteristics. The 4-year rates in these groups were, respectively, 12.8, 20.9, and 44.0%. Treatment reduced the risk of severe visual loss by 50% to 65% in all three groups at both 2 and 4 years, except for the NPDR group at 2 years.
Table 48.4 Cumulative 2- and 4-year rates of severe visual loss in eyes grouped by baseline retinopathy severity and treatment assignment*
Figure 48.16 depicts cumulative rates of severe visual loss by treatment assignment (argon and xenon groups combined) for up to 6 years. Two separate analyses are summarized, one excluding and the other including visits made after the 1976 protocol change. The curves for control-group eyes are very similar over the first 20 months of follow-up, and those for treated eyes are similar over at least the first 28 months. The difference between the two control-group curves is probably due, at least in part, to the beneficial effect of treatment experienced by some of these eyes after the protocol change, and the long-term analysis probably underestimates treatment effect. In each of these analyses, treatment reduced the risk of severe visual loss by 50% or more at and after the 16-month visit.130 In Fig. 48.17, the plots from Fig. 48.16, including all visits, are presented separately for the argon and xenon groups. The treatment effect (i.e., the difference between treatment and control groups) appeared somewhat greater in the xenon group, but this difference was small, its statistical significance was borderline, and its clinical importance was outweighed by the greater harmful effects of DRS xenon treatment.
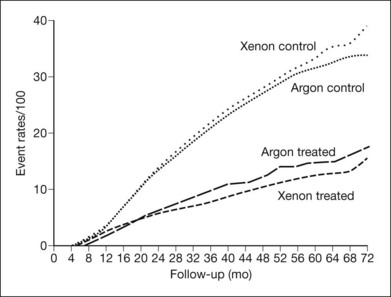
Fig. 48.17 Cumulative rates of severe visual loss by treatment group.
(Reproduced with permission from Diabetic Retinopathy Study Research Group. Photocoagulation treatment of proliferative diabetic retinopathy: clinical application of Diabetic Retinopathy Study (DRS) findings. DRS report number 8. Ophthalmology 1981;88:583–600. Copyright © 1981 American Academy of Ophthalmology.)
A temporary decrease in visual acuity is frequently noted after extensive scatter photocoagulation, with recovery to the pretreatment level in most cases within several weeks. In the DRS, visual acuity decreases of one or more lines from which recovery did not occur were attributed to treatment in 14% of argon-treated and 30% of xenon-treated eyes. Visual field losses were also more common in the xenon group130,131 (Table 48.4). In a small subgroup of eyes with severe fibrous proliferations or localized traction retinal detachment, or both, visual acuity decreases of 5 lines or more were attributed to xenon treatment in 18% of eyes but were not significantly more frequent in argon-treated than in control eyes.131
In Fig. 48.18, the Fig. 48.16 plots including all visits are presented separately for the three subgroups shown in Table 48.4. In each subgroup treatment reduced the risk of severe visual loss to about one-half of that observed in control-group eyes, but this effect became apparent later, and the percentage of eyes treated that benefited (the arithmetic difference between treated and control groups) was smaller as retinopathy severity decreased. On the basis of this analysis and the estimates of the harmful effects of treatment summarized in Table 48.5, the DRS confirmed its previous conclusion that, for eyes with high-risk characteristics, the chance of benefit from treatment clearly outweighed its risk and recommended prompt photocoagulation for most such eyes.130
Table 48.5 Estimated percentages of eyes with harmful effects attributable to diabetic retinopathy study treatment
Argon (%) | Xenon (%) | |
---|---|---|
Constriction of visual field | ||
(Goldmann IVe4 test object) | ||
to an average of | ||
≤ 45 degree, > 30 degree per meridian | 5 | 25 |
≤ 30 degree per meridian | 0 | 25 |
Decrease in visual acuity | ||
1 line | 11 | 19 |
≥ 2 lines | 3 | 11 |
(Reproduced with permission from Diabetic Retinopathy Study Research Group. Photocoagulation treatment of proliferative diabetic retinopathy: clinical application of Diabetic Retinopathy Study (DRS) findings. DRS report number 8. Ophthalmology 1981;88:583–600. Copyright © 1981 American Academy of Ophthalmology.)
For eyes with severe NPDR or PDR without high-risk characteristics, the DRS concluded that either prompt treatment or careful follow-up with prompt treatment if high-risk characteristics developed was satisfactory and that DRS results were not helpful in choosing between these strategies. In unadjusted analyses of DRS control-group eyes that had PDR without high-risk characteristics, the severity of each of three retinopathy characteristics was associated with risk of visual loss: retinal hemorrhages or microaneurysms, arteriolar abnormalities, and venous caliber abnormalities. These lesions – and soft exudates and IRMA – were also risk factors for visual loss in control-group eyes with NPDR.33 A multivariable analysis that included all DRS control-group eyes found baseline visual acuity, extent of NVD, elevation of NVD (a measure of contraction of vitreous and fibrous proliferations), and severity of hemorrhages or microaneurysms, arteriolar abnormalities, venous caliber abnormalities, and vitreous or preretinal hemorrhage all to be risk factors for visual loss. Neither in this analysis, nor in a similar one confined to DRS control-group eyes that were free of NVD, was the extent of NVE found to be a risk factor.132 These findings support clinical impressions that NVE on the surface of the retina often proliferate and regress over a period of years, remaining asymptomatic unless contraction of vitreous and fibrous proliferations begins, and that the severity of intraretinal lesions may be of greater prognostic importance than the extent of NVE.
When the DRS first reported evidence of a beneficial treatment effect and modified its protocol to encourage treatment of control-group eyes with high-risk characteristics, it also modified its treatment protocol. Because the harmful effects of the DRS argon treatment were less than those observed with the xenon treatment used in the DRS, argon was given preference and, in the hope of further reducing harmful side-effects, scatter treatment was more often divided between two or more episodes several days apart. However, because the beneficial treatment effect in the xenon group, in which no focal treatment had been applied to NVD or elevated NVE, had been at least as great as that in the argon group, these technically difficult parts of the argon protocol were dropped. Two large case series133,134 and two smaller randomized trials reported beneficial treatment effects similar to those found in the DRS.135,136
Early treatment diabetic retinopathy study and the timing of treatment
As mentioned previously for eyes with severe NPDR or early (not high-risk) PDR, DRS results were not helpful in determining which of two treatment strategies would be attended by a more favorable visual outcome: (1) immediate photocoagulation or (2) frequent follow-up and prompt initiation of photocoagulation only if high-risk PDR developed. One of the goals of the Early Treatment Diabetic Retinopathy Study (ETDRS), a randomized clinical trial sponsored by the National Eye Institute, was to compare these alternatives (designated “early photocoagulation” and “deferral of photocoagulation,” respectively) in patients with mild to severe NPDR or early PDR, with or without macular edema.137 Other goals were to evaluate photocoagulation for diabetic macular edema138 and to determine the possible effects of aspirin on DR.139 Between 1980 and 1985, 3711 patients were enrolled and assigned randomly to aspirin 650 mg/day or placebo. One eye of each patient was randomly assigned to early photocoagulation and the other to deferral. Follow-up ranged from 3 to 8 years. Eyes assigned to early photocoagulation were randomly assigned to either of two scatter treatment protocols, full or mild. The full scatter protocol called for 500 µm, 0.1 sec argon blue–green or green laser burns of moderate intensity, placed one-half burn apart, extending from the posterior pole to the equator. Between 1200 and 1600 burns were applied, divided between two or more sittings. The mild scatter protocol was the same, except that 400–650 more widely spaced burns were applied to the same area in a single sitting. Direct (local) treatment was specified for patches of flat surface NVE that were two disc areas or less in extent (the area of a circle about 1.4 times the diameter of the disc), using confluent, moderately intense burns that extended 500 µm beyond the edges of the patch. For larger patches or several small ones close together, full scatter alone to this area was an acceptable alternative. No direct treatment was carried out for NVD.140
One important outcome measure used in the ETDRS was the first occurrence of either severe visual loss, as defined in the DRS, or vitrectomy.137 These events were combined because progression to a stage requiring vitrectomy may rightly be considered an undesirable outcome for ETDRS-eligible eyes and since presumably most eyes selected for vitrectomy before the occurrence of severe visual loss (68% of the 243 ETDRS eyes undergoing vitrectomy) would have developed severe visual loss within several months if vitrectomy had not been performed. Five-year life-table rates of severe visual loss or vitrectomy, and relative risks for early photocoagulation compared to deferral over the entire follow-up period, are shown in Table 48.6. The first two rows include eyes with macular edema, subdivided by retinopathy severity. As anticipated, the poor outcome was more frequent in eyes with more severe retinopathy (in the deferral group, 10% in eyes with severe NPDR or early PDR versus 4% in eyes with mild to moderate NPDR). In both of these retinopathy subgroups, early treatment reduced the event rate to about one-half that of the deferral group, but the percentage of eyes treated that benefited was only 2–4%. The third row of the table includes all eyes without macular edema regardless of retinopathy severity (eyes with mild NPDR were not eligible unless macular edema was present), and outcome here was intermediate between that in rows 1 and 2. Some harmful effects of scatter photocoagulation were also observed in the ETDRS, including an early decrease in visual acuity (a doubling or more of the visual angle at the 4-month visit in about 10% of eyes assigned to early full scatter, compared to about 5% of eyes assigned to deferral) and some decrease in visual field. Both beneficial and harmful effects were somewhat greater with full than with mild scatter.
Table 48.6 Cumulative 5-year rates of severe visual loss or vitrectomy, and relative risks for the entire period of follow-up, by baseline retinopathy status and treatment group*
Figure 48.19 presents cumulative incidence rates of high-risk PDR in ETDRS eyes assigned to deferral of photocoagulation, by severity of retinopathy at baseline. It is of interest that high-risk PDR developed at about equal rates in eyes with moderate PDR or very severe NPDR, with rates of about 50% after 18 months. Figures 48.15, 48.20, and 48.21 present the standard photographs used in the definitions of high-risk PDR and very severe NPDR, the most important severity levels for application to clinical practice. It is convenient to express the approximate definitions of severe and very severe NPDR with the 4–2–1 rule described earlier (see Box 48.1).
These initial ETDRS recommendations were made without regard to patient age or type of diabetes. Subsequent analyses of ETDRS data suggest that, among patients whose retinopathy is in the severe NPDR to non-high-risk PDR range, the benefit of prompt treatment is greater in those who have type 2 diabetes or are older than 40 years of age (these characteristics are highly correlated, and analyses using either gave almost identical results)141 (Fig. 48.22). In the type 2 group, the 5-year rate of severe visual loss or vitrectomy was about 5% in eyes assigned to early photocoagulation versus 13% in eyes assigned to deferral, whereas in the type 1 group the rates were about 8% in both treatment groups (Fig. 48.19). In eyes assigned to deferral, severe visual loss or vitrectomy developed over the first 3 years at about the same rate in both diabetes types; apparently the greater treatment effect in type 2 diabetes resulted mainly from greater responsiveness to early treatment. The DRS also found greater photocoagulation treatment benefit in patients with type 2 diabetes.141 Greater responsiveness to photocoagulation in older versus younger patients has also been observed in other studies.142,143 These studies are consistent with the clinical impression that, in patients with type 2 diabetes, high-risk PDR is often first detected on the basis of a symptomatic vitreous hemorrhage in an eye in which new vessels had not been observed on previous visits, whereas in patients with type 1 diabetes, NVD is more often the first sign of high-risk PDR, an occurrence more easily managed with photocoagulation.
Thus, in older patients with type 2 diabetes who have very severe NPDR or early PDR, ETDRS results and clinical impression suggest that prompt photocoagulation is probably safer than deferral. In younger patients with type 1 diabetes, ETDRS results suggest that there is little to lose from deferring scatter photocoagulation until high-risk PDR develops assuming appropriate compliance with follow-up. However, even in younger patients, when early NVD (less than shown in Fig. 48.15) is accompanied by the intraretinal signs of severe or very severe NPDR (see Fig. 48.2), prompt treatment is generally recommended. On the other hand, when younger patients have only mild intraretinal lesions and NVE (only) that appear stable, an initial period of observation is generally recommended. If new vessels are demonstrated to be growing, photocoagulation is usually recommended. However, these eyes often remain asymptomatic for many years, with little new vessel growth and often demonstrate spontaneous regression of the new vessels. In such eyes vitreoretinal adhesions tend to be delicate, and when posterior vitreous detachment occurs, there is less tendency for traction retinal detachment. When the process of posterior vitreous detachment has reached completion in such an eye, new vessels are likely to be few, narrow, elevated, and partially replaced by fibrous proliferations, and there may be little to be gained from photocoagulation at this stage unless vitreous hemorrhages are occurring. Presumably the reduced aggressiveness of new vessels in the setting of posterior vitreous detachment results from the lack of the posterior vitreous face scaffold for proliferation and less possibility for retinal traction. These findings support studies regarding the therapeutic usefulness of pharmacologic vitreolysis described later in this chapter.
Systemic factors should also be considered when deciding whether to initiate treatment in patients with very severe NPDR or moderate PDR. The progression of retinopathy may accelerate during pregnancy,144,145 development of renal failure,146 extreme illness, and poor glycemic control. If photocoagulation is deferred until high-risk characteristics develop in these situations, these more pressing problems may make it difficult to provide prompt and complete photocoagulation.
Scatter photocoagulation and macular edema
Macular edema sometimes increases, at least temporarily, after scatter photocoagulation, and this edema may be followed by transient or persistent reduction of visual acuity.147–149 In the ETDRS, 18% of eyes had center-involved DME and less severe retinopathy without center involvement at baseline at 4 months.137 The DRS also found early harmful effects, which were greater in the xenon group.131 At the 6-week post-treatment visit, 21% of argon-treated and 46% of xenon-treated eyes that had DME and were free of high-risk characteristics at baseline had a decrease in visual acuity of two or more lines, compared with 9% of untreated eyes. Comparable percentages for eyes with neither DME nor high-risk characteristics were 9% argon, 18% xenon, and 3% untreated. After one year of follow-up in the group with DME at baseline, the greater progression of retinopathy in untreated eyes had led them to catch up with treated eyes; the percentages with a decrease in visual acuity of 2 or more lines were 32% argon, 33% xenon, and 34% untreated.130,150 Both the ETDRS and the DRS support the clinical impression that eyes with DME requiring scatter treatment are at less risk of visual acuity loss when focal or grid treatment to reduce the DME precedes scatter photocoagulation. If a delay of scatter treatment seems undesirable, the ETDRS protocol can be used, combining focal/grid treatment for macular edema with scatter treatment during the first photocoagulation sitting.140,151 VEGF inhibitors combined with either immediate or deferred macular laser have been shown to be more effective at reducing visual loss than laser alone.152–154 In eyes with DME and PDR, VEGF inhibitors used to treat the edema may have a temporary beneficial effect on the retinal neovascularization until PRP can be performed. Certainly, scatter treatment should not be delayed when the risks of vitreous hemorrhage or neovascular glaucoma seem high, regardless of the status of the macula.
The Diabetic Retinopathy Clinical Research Network (DRCR.net) evaluated 364 eyes with center-involved ME to evaluate the short-term effects of intravitreal ranibizumab or intravitreal triamcinolone on preexisting ME in eyes receiving both PRP for severe NPDR (or non-high risk PDR) and focal/grid laser for concurrent ME.155 Mean change in visual acuity from baseline was significantly better in the ranibizumab (+1 letters; P <0.001) and triamcinolone (+2 letters; P < 0.001) groups compared with the sham group (−4 letters) at the 14-week visit. The effect on retinal thickness mirrored these results. These differences were not maintained when study participants were followed for 56 weeks for safety outcomes. These data suggested that at least in the short term, exacerbation of DME and visual acuity loss following PRP in eyes also receiving focal/grid laser for ME can be reduced by intravitreal triamcinolone or ranibizumab. Whether continued long-term intravitreal treatment is beneficial cannot be determined from this study.
As noted above, the ETDRS divided PRP into two or more sittings in an effort to reduce side effects, including exacerbation of ME. Utilizing optical coherence tomography (OCT), studies have evaluated the effect of PRP on macular thickness. Alterations in OCT measured macular thickening during and after PRP in patients with severe DR and good vision were evaluated in a study comparing weekly versus biweekly spacing between episodes of PRP.156 Thirty-six patients with severe nonproliferative or early proliferative retinopathy and 20/20 vision in each eye at baseline received scatter photocoagulation in the following manner: in one eye, one quadrant was treated every week for 4 weeks and in the other eye, one quadrant was treated every other week for 8 weeks. OCT assessment of macular thickness was performed at baseline, before each session of photocoagulation, and at 16 weeks, the end of the follow-up period. Four of 36 eyes in the weekly treatment group and 3 of 36 in the biweekly group developed center-involved DME with decreased visual acuity (from 20/40 to 20/200), which was treated with photocoagulation. Additional photocoagulation for residual neovascularization was carried out in 35–40% of eyes in each group. The response of the remaining eyes is shown in Fig. 48.23. At baseline, mean retinal thickness was 191 µm in the central zone of the macular grid (1000 µm in diameter) in both groups. Thickness increased progressively after each weekly treatment to a maximum of 275 µm at week 4 and then decreased to 225 µm at 16 weeks. The increase was less after the biweekly treatments and at 16 weeks was closer to, but had not reached, the baseline value. There was little change in retinal thickness outside the central zone with either treatment technique.
The DRCR.net conducted an observational study to compare the effects of 1-sitting vs 4-sitting PRP on DME in subjects with severe nonproliferative or early proliferative diabetic retinopathy with relatively good visual acuity and no or mild center-involved macular edema.157 Patients enrolled in the study were treated with 1 sitting or 4 sittings of PRP in a nonrandomized, prospective, multicenter clinical trial. The median change in central subfield thickness was slightly greater in the 1-sitting group (n = 84) than in the 4-sitting group (n = 71) at the 3-day (+9 µm vs +5 µm, P = 0.01) and 4-week visits (+ 13 µm vs +5 µm, P = 0.003). At the 34-week primary outcome visit, the slight differences had reversed, with the thickness being slightly greater in the 4-sitting group than in the 1-sitting group (+14 µm vs +22 µm, P = 0.06). Visual acuity changes paralleled OCT changes: 1 vs 4 sittings at 3 days (−3 vs −1 letters, P = 0.005), 4 weeks (−1 vs −1 letters, P = 0.37) and 34 weeks (0 vs −2 letters, P = 0.006). The results of the study suggest that clinically meaningful differences are unlikely in OCT thickness or visual acuity following application of PRP in a single sitting compared with 4 sittings in patients without center-involved macular edema and good vision.
Panretinal photocoagulation and advanced proliferative diabetic retinopathy
There is widespread agreement that PRP should be performed promptly in most eyes with PDR and high-risk characteristics. However, progressive contraction of fibrous proliferations leading to displacement or detachment of the macula sometimes follows PRP in eyes with extensive fibrous proliferations (see Fig. 48.11). Such cases have led to some reluctance to advise photocoagulation in this situation. Few such eyes were included in the DRS, but analyses of such cases indicated that outcome was better with photocoagulation than without it and suggested that it is only excessively heavy treatment that should be avoided. The adverse treatment effect was only evident in the xenon group, and even there the benefit of treatment outweighed its risks.131 When high-risk characteristics are definitely present, PRP should usually be carried out, despite the presence of fibrous proliferation or localized traction retinal detachment. Treatment directly over areas of fibrous proliferation and retinal detachment should be avoided, and treatment strength should be mild to moderate.
Extensive neovascularization in the anterior chamber angle is a strong indication for PRP regardless of the presence of high-risk characteristics as regression of these new vessels before extensive closure of the angle has occurred can prevent neovascular glaucoma.133,158
Current techniques of panretinal photocoagulation
The DRS and ETDRS validated the effectiveness of PRP and established the indications and parameters for the treatment of PDR several decades ago.130,140 These concepts persist mostly unchanged to this day as a result of their remarkable efficacy. A summary of the current protocol for PRP is presented in Table 48.7.
Table 48.7 Current treatment protocol for panretinal (PRP) laser photocoagulation
Burn characteristic | Recommendations |
---|---|
Conventional slit-lamp laser delivery systems | |
Size (on retina) | 500 µm (e.g., argon laser using 200 µm spot size with Rodenstock lens (or equivalent), 400 µm (e.g., 200 µm spot size with Mainster 165, Volk Quadraspheric or SuperQuad 160) or 500 µm spot size with 3-mirror contact lens) |
Exposure | 0.1 seconds recommended, 0.05–0.2 allowed |
Intensity | Mild white retinal burns |
Distribution | Edges 1 burn width apart |
No. of Sessions/Sittings | Unrestricted (generally should be completed in <6) |
Nasal proximity to disk | No closer than 500 µm |
Temp. proximity to center | No closer than 3000 µm |
Superior/inferior limit | No further posterior than 1 burn within the temporal arcades |
Extent | Arcades (~3000 µm from the macular center) to at least the equator |
Number of Final Burns: | 1200 to 1600 |
Wavelength | Green or yellow (red can be used if vitreous hemorrhage is present precluding use of green or yellow) |
Automated pattern scanning laser delivery systems | |
Size (on retina) | 400 µm (e.g. 200 µm spot size with Mainster 165, Volk Quadraspheric or SuperQuad 160) |
Exposure | 0.020 seconds |
Intensity | Mild white retinal burns |
Distribution | 0.5 spot diameter spacing |
No. of sessions/sittings | 1 to 3 |
Nasal proximity to disc | No closer than 500 µm |
Temp. proximity to center | No closer than 3000 µm |
Superior/inferior limit | No further posterior than 1 burn within the temporal arcades |
Extent | Arcades (~3000 µm from the macular center) to at least the equator |
Number of final burns | 1800 to 2400 |
Wavelength | Green (532 nm) only |
Patterns | 2×2, 3×3, 4×4, 5×5 as needed for uniform focus/uptake |
Indirect laser delivery systems | |
Size (on retina) | 400 to 500 µm spot size with 20D, 28D, or 30D indirect lens (depending on diopter of Indirect lens) |
Exposure | 0.050–0.10 seconds |
Intensity | Mild white retinal burns |
Distribution | 500 µm spot diameter spacing |
No. of Sessions/Sittings | 1 to 3 |
Nasal proximity to disk | No closer than 500 µm |
Temp. proximity to center | No closer than 3000 µm |
Superior/inferior limit | No further posterior than 1 burn within the temporal arcades |
Extent | Arcades (~3000 µm from the macular center) to at least the equator |
Number of final burns: | 1200 to 2000 |
Wavelength | Green (532 nm) only |
(Adapted from the Diabetic Clinical Research Network Procedure Manuals.)
Direct (local) treatment of NVE
In the ETDRS, investigators had the option of applying direct photocoagulation (referred to as “local treatment”) to flat new vessels on the retina. Confluent argon laser burns of 200–1000 µm spot size and a 0.1–0.5 second duration were specified with a resulting appearance (Fig. 48.24A) very similar to that obtained with mild xenon arc photocoagulation. Local treatment was limited to patches of NVE <2 disc areas in size in order to avoid large scotomas or nerve fiber bundle field defects. Although most retina specialists today do not perform local treatment, the efficacy of PRP without it, although clearly good, is not precisely known.
Distribution and strength of panretinal photocoagulation
A common feature of most scatter laser treatment protocols is the location of burns beginning about 2–3 DD from the center of the macula and extending peripherally to the equator. It is important to realize that the size of the burn produced depends not only on the spot-size setting used, but also on power and duration, so it is difficult to compare techniques precisely, even those using the same wavelength and spot-size setting, on the basis of number and theoretical size of burns. It is also difficult to describe burn strength. Reporting power level is not particularly helpful, since the required power for a burn of given strength, even if spot-size setting and duration are kept constant, depends on the clarity of the media and the pigmentation of the fundus. The ETDRS protocol for full scatter treatment provides useful guidelines for initial treatment,140 calling for a total of 1200–1600 500 µm 0.1 s argon laser burns of moderate intensity placed one-half to one burn apart and divided between two or more sittings (at least 2 weeks apart if two sittings and at least 4 days apart if three or more sittings). Burns appear to enlarge slightly within several minutes after their application, resulting in the closer spacing of the scatter burns shown in Fig. 48.24A.
Pattern scanning laser delivery systems
Preclinical animal experiments on the effects of various pulse durations demonstrated that laser burns of pulse durations greater than 20 ms resulted in significant diffusion of heat with a less homogeneous lesion on histological examination.159 Pulse durations of approximately 20 ms have been shown to represent an optimal compromise between the favorable impact of speed, spatial localization, and reduced collateral damage with a sufficient therapeutic window.160 The 10–30 ms pulse of the pattern laser systems is associated clinically with a more uniform and predictable burn dimension with minimal thermal spread compared with conventional photocoagulation.159,161,162 Clinically, pattern scanning laser burns are typically lighter in intensity and more uniform, as confirmed by clinical retinal morphologic studies that demonstrated pattern scanning laser burns to be nearly identical in size and sharply delineated from the surrounding untreated retina.163 Furthermore, the area of tissue destruction was confined to the outer retinal layers, from the outer nuclear layer to the RPE, reflecting less energy spread and less damage to the inner retinal layers and choriod163 (Fig. 48.24).
Number of episodes used for scatter treatment
The effect of single and multiple laser sittings on DME has been discussed earlier in this chapter, noting that complete application within a single sitting did not appear to be detrimental as compared with dividing treatment over multiple sittings. Among the techniques in use for PDR alone, the number of sittings in which initial scatter treatment is carried out varies from one to four or more. Those techniques using a smaller number of larger burns tend toward a single sitting, sometimes with retrobulbar anesthesia, whereas those using a larger number of smaller burns have generally been divided into two or more sittings. In general, multiple sittings may reduce discomfort, but may cause delays and inconvenience for patients. Angle-closure glaucoma secondary to serous detachment of the peripheral choroid and ciliary body is reportedly less common when treatment is carried out in two or more sessions over a period of 1 or 2 weeks.164
Doft and Blankenship165 addressed this question in a small randomized trial. They randomly assigned one eye from each of 50 patients with DRS high-risk characteristics to scatter photocoagulation with 1200, 500 µm, 0.1 s argon laser burns of moderate intensity applied in either of two ways: (1) at a single sitting or (2) in three sittings at 1-week intervals. Retrobulbar anesthesia was used in the single-sitting group and for the first of the multiple-sitting group. In this latter group at the first episode, several rows of burns were placed surrounding the posterior pole, and treatment was extended temporally and nasally to the periphery, leaving the remainder of the upper quadrants (from the 10 o’clock to the 2 o’clock position) for the second episode and the remainder of the lower quadrants (from the 4 o’clock to the 8 o’clock position) for the third. Surrounding the posterior pole with treatment at the initial sitting is different from most protocols, where posterior burns are usually divided between multiple sittings.
Wavelength
The Krypton-Argon Regression of Neovascularization Study (KARNS) randomly assigned 907 eyes (of 696 patients) that had NVD equaling or exceeding those in Fig. 48.15 to scatter photocoagulation (1600–2000 moderate-intensity 500 mm burns) with either blue–green argon or red krypton wavelengths.142 If, after the initial treatment, NVD increased by more than 0.5 disc area, retreatment was recommended (and could also be applied for other reasons, such as increasing NVE or vitreous hemorrhage). Retreatment was carried out in 36% and 33%, respectively, of the argon and krypton groups. Worsening NVD was the reason for the retreatment in ~40% of retreated eyes in each group. Regression of NVD to less than that shown in Fig. 48.15 was observed in almost identical proportions of the two groups (41.4% and 41.8%, respectively, at 3 months and 55.0% and 52.8%, respectively, at 1 year). No differences were found in the effectiveness of argon versus krypton treatment, although retrobulbar anesthesia was used more frequently with krypton treatment. In two small randomized trials, diode (810 nm) and double-frequency Nd : YAG (532 nm) lasers gave results similar to those of the argon green laser.166,167
Regression of new vessels after initial photocoagulation and indications for retreatment
There is general agreement that substantial regression of new vessels usually occurs within days or weeks after the initial application of scatter photocoagulation and that eyes in which new vessels continue to grow despite initial treatment or recur after partial or complete regression may respond well to additional treatment. However, data documenting the rapidity and completeness of regression are sparse, and many reports do not clearly separate results after initial treatment and results after initial treatment plus retreatment as needed. Table 48.8 summarizes much of the available information. Doft and Blankenship168 combined the single- and multiple-treatment groups previously discussed and described the course of new-vessel regression over a 6-month period without retreatment. At the 3-day post-treatment visit 10 (20%) of the 50 eyes had regressed from the high-risk stage; at 2 weeks, 25 (50%); at 3 weeks, 36 (72%); and at 6 months, 31 (62%). About one-third of the eyes that were still in the high-risk stage after 3 weeks were no longer high-risk at 6 months. Conversely, approximately one-third of those with regression at 3 weeks had progressed to the high-risk stage again at 6 months. Blankenship169 reported similar findings in his study comparing peripheral and posterior laser treatment. In both groups combined there were 31 eyes with NVD greater than or equal to one-quarter disc area at baseline. In all but one of these 31 eyes, NVD were less than one-quarter disc area at the 1-month follow-up visit while at the 6-month visit, 24 of the 31 (77%) met this criterion. Vander and coworkers 170 reported that 59% of 59 eyes with high-risk PDR had a satisfactory response (retinopathy no longer in the high-risk category) 3 months after initial full-scatter photocoagulation. In the KARNS, 3 months after the initial treatment (with or without retreatment), NVD had decreased to less than 50% of the baseline area in 54% of eyes and had decreased by a lesser amount in an additional 19%.142 Among DRS eyes with NVD equaling or exceeding those in Fig. 48.15 at baseline (with or without NVE), all new vessels had regressed completely 1 year after the initial treatment in 20% of argon-treated eyes, and regression of NVD to less than those in Fig. 48.15 had occurred in an additional 34%. Results after xenon arc photocoagulation were similar.26 Because the DRS protocol did not provide mandated guidelines for retreatment, and because treatment was being compared with no treatment, these results probably reflect outcomes to be expected after initial treatment.
Efficacy of retreatment can be difficult to compare between studies. After applying 1100–1500 500 µm moderately intense argon laser burns, divided between two sittings about 1 week apart, Rogell171 followed patients at monthly intervals, applying 300–400 additional burns at each visit until “substantial regression” of new vessels had occurred. In 21 of 55 eyes (38%) with PDR, the initial treatment was sufficient. Additional photocoagulation was carried out once or twice in 29 eyes (53%), three to five times in 5 eyes (1%), and peripheral retinal cryotherapy was added in 2 eyes (4%). Response to retreatment was considered satisfactory in 30 of 34 eyes (88%). In a retrospective case review of 182 patients (294 eyes), Reddy and coworkers172 assessed the need for retreatment after initial full-scatter photocoagulation in patients with two or more NV-VH risk factors. In 177 of 294 eyes (60%) the response to initial treatment was considered satisfactory. The remaining 117 eyes were retreated, 71 with photocoagulation alone and 46 with a combination of photocoagulation and anterior retinal cryotherapy. Retreatment was successful in 98 of 117 eyes (84%). Among an unspecified number of eyes with high-risk PDR treated initially with extensive argon laser scatter photocoagulation during a 2-year period, 23 eyes remained in the high-risk stage 8 weeks after completion of the initial treatment and were given follow-up treatment by Vine.173 Eyes were observed at 6- to 8-week intervals, and follow-up treatment (1000–2000 low-intensity burns placed between scars) was applied until regression from the high-risk stage occurred or further treatment was not feasible. Retreatment was successful in 12 of 23 eyes (52%).
From these studies it appears that, on average, about two-thirds of eyes have a satisfactory response to initial scatter treatment. As mentioned earlier, this ratio tends to be more favorable in patients with type 2 diabetes. Patients with severe intraretinal lesions and actively growing new vessels, who typically have type 1 diabetes, often need multiple treatments.174
The ETDRS protocol contains guidelines for follow-up treatment that seem suitable for general use. Six factors are considered: (1) change in new vessels since the last visit or last photocoagulation treatment; (2) appearance of the new vessels (caliber, degree of network formation, extent of accompanying fibrous tissue); (3) frequency and extent of vitreous hemorrhage since the last visit or last photocoagulation treatment; (4) status of vitreous detachment; (5) extent of photocoagulation scars; and (6) extent of traction retinal detachment and fibrous proliferations.140 If new vessels appear to be active, as suggested by formation of tight networks, paucity of accompanying fibrous tissue, and increase in extent in comparison to the previous visit, additional photocoagulation is considered. The case for additional treatment is stronger if the extent of new vessels is substantially greater than it was at the time of initial treatment for high-risk characteristics or if vitreous or preretinal hemorrhages are occurring repeatedly (Fig. 48.6). Decreased caliber of new vessels and fibrous proliferation development suggest that retinopathy is entering a quiescent stage in which additional treatment may not be needed. A single episode of vitreous hemorrhage coincident with the occurrence of extensive posterior vitreous detachment, particularly if the only vitreoretinal adhesion remaining is at the disc, argues less for additional photocoagulation than do recurrent hemorrhages unrelated to such an occurrence. Additional photocoagulation may be needed less frequently in the setting of extensive posterior vitreous detachment because additional growth of new vessels is limited as is the extent of vitreoretinal adhesions. The extent and location of photocoagulation scars may also influence the decision regarding additional photocoagulation treatment. If the scars are widely spaced, or if there are areas where treatment was omitted, benefit from additional photocoagulation is more likely. In the presence of extensive scars where additional treatment would be placed over old scars, additional treatment may be less beneficial and be associated with increased risk of side-effects such as loss of visual field or nyctalopia. In such cases indefinite deferral of photocoagulation may sometimes be the best alternative, even though there are extensive new vessels on the detached posterior vitreous surface and/or small vitreous hemorrhages are occurring occasionally. If severe vitreous hemorrhage or vision threatening proliferation occurs, vitrectomy may be the best treatment option.
Complications of prp
Although remarkably effective, visually threatening complications from PRP may arise (Box 48.2). It is important that such complications are identified and treated promptly to prevent further vision loss. Diabetes affects most ocular structures and may increase risks of potential complications following PRP. Diabetes-related corneal neuropathy may predispose eyes to corneal epithelial trauma possibly leading to secondary infection or recurrent corneal erosions if undetected.175 The use of the appropriate coupling solution, proper placement and minimal manipulation of the contact lens during laser treatment may minimize shearing and friction of the corneal surface. Iritis or iris burns and lenticular burns may occur with poorly dilated pupils, cataractous lenses or improper laser focus.176 Careful focusing and optimizing papillary dilation can help avoid these complications, particularly when high-power, long-duration laser treatment is used. Some degree of discomfort is reported in most patients undergoing PRP. Carefully titrating burn intensity, shortening exposure time, and avoiding the ciliary nerves at the horizontal meridian will minimize patient discomfort. Judicious use of retrobulbar, peribulbar, subtenon or subconjunctival anesthesia may be used to increase patient comfort and effectively complete treatment in some patients. Such procedures are usually not required, however, in the vast majority of individuals.
Box 48.2
Complications of scatter (PRP) photocoagulation
The inherent destructive nature of PRP may also lead to adverse effects on the retina, including macular edema, serous macular/retinal detachment, contraction of preretinal membranes, choroidal detachment and angle closure glaucoma.177 Choroidal effusion is commonly observed after PRP within the first week. These effusions are usually asymptomatic but may cause angle closure, especially in predisposed hyperopic eyes with shallow chambers. Inadvertent direct photocoagulation of the central macula is a potential and obviously detrimental complication that can result in permanent scotoma and substantial visual loss. Careful attention of anatomic landmarks and adherence to a strict standardized treatment regimen for each patient can reduce this risk. Intense, small spot-size burns, especially in the red light spectrum, may predispose to the rupture of Bruch’s membrane. Clinically, Bruch’s membrane rupture is associated with risk of hemorrhage and secondary choroidal neovascularization. Intermittent direct pressure on the globe with the contact lens often stops any associated hemorrhaging and low intensity, confluent large laser burns may be applied to the bleeding area to help control hemorrhaging. Inadvertent photocoagulation of the long posterior ciliary nerve may result in permanent mydriasis and loss of accommodation.178 Substantial visual field loss may be caused by high-density, high-intensity PRP and a sudden decrease in central vision may occur with occlusion of limited remaining arterioles supplying the macula.132 Even with uncomplicated PRP, some decline in visual function has been reported, including moderate visual loss, impaired night vision, diminished visual field, reduced color vision, and reduced contrast sensitivity.179
Antiangiogenic therapies for proliferative diabetic retinopathy
Intravitreal antiangiogenic agents can induce rapid initial regression of ischemia-related ocular neovascularization. Although the neovascular regression effect can be remarkable in terms of its speed and extent, the effect generally appears to be temporary, requiring either additional definitive treatment (such as laser photocoagulation) or repeated dosing. Generalized use of VEGF inhibitors for PDR will likely be limited until there is development of tolerable continuous delivery mechanisms or identification of anti-VEGF agents with long duration of action. Various VEGF inhibitors have shown at least transient beneficial activity in PDR. Mendrinos and coworkers published a case report demonstrating rapid regression of neovascularization after a single injection of pegaptanib which was sustained for more than 15 months.180 Bevacizumab has been shown by Avery et al. to induce regression of new vessels in PDR as early as 24 hours after injection, but with a variable sustained effect ranging between 2 and 11 weeks.181 Jorge et al. demonstrated short-term resolution of leakage from retinal neovascularization at 6 weeks after a single intravitreal injection of bevacizumab in 15 patients with persistent new vessels despite panretinal laser photocoagulation. At 12 weeks, 14 out of 15 patients had recurrent leakage but to a lesser extent than at baseline.182 Other studies have reported similar short-term efficacy of bevacizumab in inducing regression of retinal neovascularization.183–185 The Diabetic Retinopathy Clinical Research Network (DRCR.net) is conducting two trials: one to study the efficacy of ranibizumab in cases of vitreous hemorrhage due to PDR, and another to assess the efficacy of adjunctive use of ranibizumab compared to triamcinolone with laser treatment in PDR. The results of these trials will help further define the role of VEGF inhibitors in the management of diabetic ocular neovascular complications and provide additional safety data for long-term exposure that is presently unknown.
It has been postulated that VEGF inhibition for PDR would require extended treatment durations since the underlying ischemia leading to VEGF expression would not be ameliorated. Some initial data suggest, however, that this may not be the case. The natural history of PDR is to become quiescent eventually with or without laser treatment (although of course visual outcomes are improved with laser photocoagulation). Intraocular VEGF concentrations have been shown to be lower in quiescent PDR, whether following laser photocoagulation or spontaneously.13A similar argument regarding the need for prolonged treatment might be made for DME; however, studies from DRCR.net and others have shown a reduced need for VEGF inhibitors over time.152 The mean number of intravitreal ranibizumab injections was 8–9 of 13 possible the first year but fell to 2–3 the second year without a loss of visual acuity or an increase in retinal thickness. Together, these data suggest that after a period of VEGF inhibitor treatment, it may be possible to reduce such treatment without reactivation of disease. Whether this holds true, and to what extent, remains to be demonstrated by ongoing and upcoming clinical trials.
Pharmaceutical vitreolysis for treatment of pdr
As discussed earlier in this chapter, the vitreous plays a key role in the pathology and natural course of PDR. The presence of a complete posterior vitreous detachment (PVD) has been observed in diabetic eyes to be associated with lower risk for developing PDR.186,187 The widespread utilization of the intravitreal injections has led to research into pharmacologic agents such as plasmin, tissue plasminogen activator, microplasmin, hyaluronidase, chondroitinase, collagenase, dispase, and nattokinase.188 These agents may be used to facilitate the cleavage of the cortical hyaloid at the vitreoretinal junction, thereby inducing complete PVD and liquefaction of the vitreous gel.189 In a pooled analysis of the initial clinical trials with hyalonidase involving 1125 patients with persistent vitreous hemorrhage who were randomized to receive one of two doses of intravitreal hyaluronidase (55 or 75 IU) or saline,190 only patients receiving 55 IU reached the primary efficacy endpoint, defined as (3 months after baseline) clearance of hemorrhage sufficient to see the underlying pathology or allow completion of treatment when indicated. Acute self-limited iritis was the most common adverse event, occurring in a dose-dependent fashion.184
Microplasmin, a recombinant protein that contains only the catalytic domain of human plasmin, has shown ability to induce vitreolysis in patients with vitreomacular adhesion. A phase II randomized double-masked clinical trial with control sham injection enrolled 60 patients. Patients in each of the four cohorts were randomized (4 : 1) to active treatment or sham injection. In the first three cohorts, increasing doses of microplasmin (75, 125, and 175 µg) were administered. In the fourth cohort, an initial injection of 125 µg microplasmin or sham was administered followed 1 month later by an injection of 125 µg microplasmin if no release of vitreoretinal adhesion occurred. A third dose was injected 4 weeks later if there was still no release of adhesion. Within 28 days of sham, 75, 125, and 175 µg microplasmin resulted in nonsurgical resolution of vitreomacular adhesion in 8%, 25%, 44%, and 27% of the patients, respectively. When the 125 µg dose was repeated up to two times, adhesion release was observed in 58% of patients 28 days after the final injection.191These data suggest that nonsurgical enzymatic release of vitreoretinal adhesions may potentially provide a less invasive means to achieve a more nearly complete cleavage of the vitreous as compared to conventional vitrectomy. In addition to the mechanical removal of vitreoretinal adhesions, complete vitreolysis changes the molecular flux across the vitreoretinal interface and improves oxygen supply to the retina.12,192 This action could potentially delay the onset or progression of PDR. Further studies are underway to better define the role of pharmacologic vitreolysis in the treatment of PDR.
Indications for vitrectomy
When vitrectomy was initially introduced in 1970 by Machemer et al.,193 the major indications in eyes with PDR were severe vitreous hemorrhage that had failed to clear spontaneously after a year and traction retinal detachment involving the center of the macula. As this procedure came into widespread use, it was recognized that it can be of value earlier in the course of severe PDR and its indications have broadened56,57 (Box 48.3). A more extensive discussion of vitrectomy and surgical interventions for the proliferative complications of diabetic retinopathy is presented in Chapter 111 (Surgery for proliferative diabetic retinopathy).
Box 48.3
Indications for vitrectomy
1 Diabetic Retinopathy Study Research Group. Preliminary report on effects of photocoagulation therapy. Am J Ophthalmol. 1976;81:383–396.
2 Diabetic Retinopathy Study Research Group. Four risk factors for severe visual loss in diabetic retinopathy. The third report from the Diabetic Retinopathy Study. Arch Ophthalmol. 1979;97:654–655.
3 Klein R, Klein BE, Moss SE, et al. The Wisconsin Epidemiologic Study of Diabetic Retinopathy. IX. Four-year incidence and progression of diabetic retinopathy when age at diagnosis is less than 30 years. Arch Ophthalmol. 1989;107:237–243.
4 Klein R, Klein BE, Moss SE, et al. The Wisconsin Epidemiologic Study of Diabetic Retinopathy. X. Four-year incidence and progression of diabetic retinopathy when age at diagnosis is 30 years or more. Arch Ophthalmol. 1989;107:244–249.
5 Klein R, Knudtson MD, Lee KE, et al. The Wisconsin Epidemiologic Study of Diabetic Retinopathy. XXII. The twenty-five-year progression of retinopathy in persons with type 1 diabetes. Ophthalmology. 2008;115(11):1859–1868.
6 Aiello LP, Cahill MT, Wong JS. Systemic considerations in the management of diabetic retinopathy. Am J Ophthalmol. 2001;132:760–766.
7 Klein R, Klein BE, Moss SE. Epidemiology of proliferative diabetic retinopathy. Diabetes Care. 1992;15:1875–1891.
8 Hovind P, Tarnow L, Rossing K, et al. Decreasing incidence of severe diabetic microangiopathy in type 1 diabetes. Diabetes Care. 2003;26:1258–1264.
9 Klein R, Klein BE, Moss SE, et al. The medical management of hyperglycemia over a 10-year period in people with diabetes. Diabetes Care. 1996;19:744–750.
10 Wong TY, Mwamburi M, Klein R, et al. Rates of progression in diabetic retinopathy during different time periods: a systematic review and meta-analysis. Diabetes Care. 2009;32:2307–2313.
11 Klein R, Klein BE. Are individuals with diabetes seeing better? A long-term epidemiological perspective. Diabetes. 2010;59:1853–1860.
12 Stefansson E. Ocular oxygenation and the treatment of diabetic retinopathy. Surv Ophthalmol. 2006;51:364–380.
13 Aiello LP, Avery RL, Arrigg PG, et al. Vascular endothelial growth factor in ocular fluid of patients with diabetic retinopathy and other retinal disorders. N Engl J Med. 1994;331:1480–1487.
14 Miller JW, Adamis AP, Shima DT, et al. Vascular endothelial growth factor/vascular permeability factor is temporally and spatially correlated with ocular angiogenesis in a primate model. Am J Pathol. 1994;145:574–584.
15 Pierce EA, Avery RL, Foley ED, et al. Vascular endothelial growth factor/vascular permeability factor expression in a mouse model of retinal neovascularization. Proc Natl Acad Sci U S A. 1995;92:905–909.
16 Adamis AP, Miller JW, Bernal MT, et al. Increased vascular endothelial growth factor levels in the vitreous of eyes with proliferative diabetic retinopathy. Am J Ophthalmol. 1994;118:445–450.
17 Avery RL. Regression of retinal and iris neovascularization after intravitreal bevacizumab (Avastin) treatment. Retina. 2006;26:352–354.
18 Cai J, Kehoe O, Smith GM, et al. The angiopoietin/Tie-2 system regulates pericyte survival and recruitment in diabetic retinopathy. Invest Ophthalmol Vis Sci. 2008;49:2163–2171.
19 Takagi H, Koyama S, Seike H, et al. Potential role of the angiopoietin/tie2 system in ischemia-induced retinal neovascularization. Invest Ophthalmol Vis Sci. 2003;44:393–402.
20 Geraldes P, Hiraoka-Yamamoto J, Matsumoto M, et al. Activation of PKC-delta and SHP-1 by hyperglycemia causes vascular cell apoptosis and diabetic retinopathy. Nat Med. 2009;15:1298–1306.
21 Watanabe D, Suzuma K, Matsui S, et al. Erythropoietin as a retinal angiogenic factor in proliferative diabetic retinopathy. N Engl J Med. 2005;353:782–792.
22 Tong Z, Yang Z, Patel S, et al. Promoter polymorphism of the erythropoietin gene in severe diabetic eye and kidney complications. Proc Natl Acad Sci U S A. 2008;105:6998–7003.
23 Abhary S, Burdon KP, Casson RJ, et al. Association between erythropoietin gene polymorphisms and diabetic retinopathy. Arch Ophthalmol. 2010;128:102–106.
24 Ogata N, Tombran-Tink J, Nishikawa M, et al. Pigment epithelium-derived factor in the vitreous is low in diabetic retinopathy and high in rhegmatogenous retinal detachment. Am J Ophthalmol. 2001;132:378–382.
25 Praidou A, Androudi S, Brazitikos P, et al. Angiogenic growth factors and their inhibitors in diabetic retinopathy. Curr Diabetes Rev. 2010;6:304–312.
26 Diabetic Retinopathy Study Research Group. Photocoagulation treatment of proliferative diabetic retinopathy: the second report of diabetic retinopathy study findings. Ophthalmology. 1978;85:82–106.
27 Shimizu K, Kobayashi Y, Muraoka K. Midperipheral fundus involvement in diabetic retinopathy. Ophthalmology. 1981;88:601–612.
28 Muraoka K, Shimizu K. Intraretinal neovascularization in diabetic retinopathy. Ophthalmology. 1984;91:1440–1446.
29 Kohner EM, Dollery CT, Bulpitt CJ. Cotton-wool spots in diabetic retinopathy. Diabetes. 1969;18:691–704.
30 Kohner EM, Dollery CT. The rate of formation and disappearance of microaneurysms in diabetic retinopathy. Trans Ophthalmol Soc U K. 1970;90:369–374.
31 Davis MD. Vitreous contraction in proliferative diabetic retinopathy. Arch Ophthalmol. 1965;74:741–751.
32 Taylor E, Dobree JH. Proliferative diabetic retinopathy. Site and size of initial lesions. Br J Ophthalmol. 1970;54:11–18.
33 Diabetic Retinopathy Study. Report number 6. Design, methods, and baseline results. Report number 7. A modification of the Airlie House classification of diabetic retinopathy. Prepared by the Diabetic Retinopathy Study Research Group. Invest Ophthalmol Vis Sci. 1981;21:1–226.
34 Prud’homme G, Rand L. The Diabetic Retinopathy Study Research Group: distribution of maximum grade of lesion in proliferative diabetic retinopathy. Invest Ophthalm Vis Sci. 1981;20(Suppl):59.
35 Joondeph BC, Joondeph HC, Flood TP. Foveal neovascularization in diabetic retinopathy. Arch Ophthalmol. 1987;105:1672–1675.
36 Chew EY, Murphy RP, Newsome DA, et al. Parafoveal telangiectasis and diabetic retinopathy. Arch Ophthalmol. 1986;104:71–75.
37 Early Treatment Diabetic Retinopathy Study Research Group. Grading diabetic retinopathy from stereoscopic color fundus photographs – an extension of the modified Airlie House classification. ETDRS report no. 10. Ophthalmology. 1991;98:786–806.
38 Wilson PJ, Ellis JD, MacEwen CJ, et al. Screening for diabetic retinopathy: a comparative trial of photography and scanning laser ophthalmoscopy. Ophthalmologica. 2010;224:251–257.
39 Oliver SC, Schwartz SD. Peripheral vessel leakage (PVL): a new angiographic finding in diabetic retinopathy identified with ultra wide-field fluorescein angiography. Semin Ophthalmol. 2010;25:27–33.
40 Schwartz JS, Pavan PR. Optic disc edema. Int Ophthalmol Clin. 1984;24:83–91.
41 Kampik A, Kenyon KR, Michels RG, et al. Epiretinal and vitreous membranes. Comparative study of 56 cases. Arch Ophthalmol. 1981;99:1445–1454.
42 Nork TM, Wallow IH, Sramek SJ, et al. Muller’s cell involvement in proliferative diabetic retinopathy. Arch Ophthalmol. 1987;105:1424–1429.
43 Dobree JH. Proliferative diabetic retinopathy: evolution of the retinal lesions. Br J Ophthalmol. 1964;48:637–649.
44 Bandello F, Gass JD, Lattanzio R, et al. Spontaneous regression of neovascularization at the disk and elsewhere in diabetic retinopathy. Am J Ophthalmol. 1996;122:494–501.
45 Tolentino FI, Lee PF, Schepens CL. Biomicroscopic study of vitreous cavity in diabetic retinopathy. Arch Ophthalmol. 1966;75:238–246.
46 Anderson B, Jr. Activity and diabetic vitreous hemorrhages. Ophthalmology. 1980;87:173–175.
47 Tasman W. Diabetic vitreous hemorrhage and its relationship to hypoglycemia. Mod Probl Ophthalmol. 1979;20:413–414.
48 Larsen HW. Diabetic retinopathy. An ophthalmoscopic study with a discussion of the morphologic changes and the pathogenetic factors in this disease. Acta Ophthalmol. 1960;Suppl 60:1–89.
49 Bresnick GH, Haight B, De Venecia G. Retinal wrinkling and macular heterotopia in diabetic retinopathy. Arch Ophthalmol. 1979;97:1890–1895.
50 Ramsay WJ, Ramsay RC, Purple RL, et al. Involutional diabetic retinopathy. Am J Ophthalmol. 1977;84:851–858.
51 Beetham WP. Visual prognosis of proliferating diabetic retinopathy. Br J Ophthalmol. 1963;47:611–619.
52 Klein R, Klein BE, Moss SE, et al. The Wisconsin epidemiologic study of diabetic retinopathy. II. Prevalence and risk of diabetic retinopathy when age at diagnosis is less than 30 years. Arch Ophthalmol. 1984;102:520–526.
53 Klein R, Klein BE, Moss SE, et al. The Wisconsin epidemiologic study of diabetic retinopathy. III. Prevalence and risk of diabetic retinopathy when age at diagnosis is 30 or more years. Arch Ophthalmol. 1984;102:527–532.
54 Klein R, Klein BE, Moss SE, et al. The Wisconsin Epidemiologic Study of Diabetic Retinopathy. XV. The long-term incidence of macular edema. Ophthalmology. 1995;102:7–16.
55 Diabetic Retinopathy Vitrectomy Study Research Group. Early vitrectomy for severe vitreous hemorrhage in diabetic retinopathy. Two-year results of a randomized trial. Diabetic Retinopathy Vitrectomy Study report 2. Arch Ophthalmol. 1985;103:1644–1652.
56 Diabetic Retinopathy Vitrectomy Study Research Group. Early vitrectomy for severe proliferative diabetic retinopathy in eyes with useful vision. Clinical application of results of a randomized trial – Diabetic Retinopathy Vitrectomy Study report 4. Ophthalmology. 1988;95:1321–1334.
57 Diabetic Retinopathy Vitrectomy Study Research Group. Early vitrectomy for severe proliferative diabetic retinopathy in eyes with useful vision. Results of a randomized trial. Diabetic Retinopathy Vitrectomy Study report 3. Ophthalmology. 1988;95:1307–1320.
58 Diabetic Retinopathy Vitrectomy Study Research Group. Early vitrectomy for severe vitreous hemorrhage in diabetic retinopathy. Four-year results of a randomized trial. Diabetic Retinopathy Vitrectomy Study report 5. Arch Ophthalmol. 1990;108:958–964.
59 Aiello LM, Rand LI, Briones JC, et al. Diabetic retinopathy in Joslin Clinic patients with adult-onset diabetes. Ophthalmology. 1981;88:619–623.
60 Zhang X, Saaddine JB, Chou CF, et al. Prevalence of diabetic retinopathy in the United States, 2005–2008. JAMA. 2010;304:649–656.
61 The effect of intensive treatment of diabetes on the development and progression of long-term complications in insulin-dependent diabetes mellitus. The Diabetes Control and Complications Trial Research Group. N Engl J Med. 1993;329:977–986.
62 UK Prospective Diabetes Study (UKPDS) Group. Intensive blood-glucose control with sulphonylureas or insulin compared with conventional treatment and risk of complications in patients with type 2 diabetes (UKPDS 33). Lancet. 1998;352:837–853.
63 Diabetes Control and Complications Trial Research Group. Progression of retinopathy with intensive versus conventional treatment in the Diabetes Control and Complications Trial. Ophthalmology. 1995;102:647–661.
64 The effect of intensive diabetes treatment on the progression of diabetic retinopathy in insulin-dependent diabetes mellitus. The Diabetes Control and Complications Trial. Arch Ophthalmol. 1995;113:36–51.
65 Effect of intensive therapy on the microvascular complications of type 1 diabetes mellitus. JAMA. 2002;287:2563–2569.
66 Sustained effect of intensive treatment of type 1 diabetes mellitus on development and progression of diabetic nephropathy: the Epidemiology of Diabetes Interventions and Complications (EDIC) study. JAMA. 2003;290:2159–2167.
67 Gerstein HC, Miller ME, Byington RP, et al. Effects of intensive glucose lowering in type 2 diabetes. N Engl J Med. 2008;358:2545–2559.
68 Holman RR, Paul SK, Bethel MA, et al. 10-year follow-up of intensive glucose control in type 2 diabetes. N Engl J Med. 2008;359:1577–1589.
69 White NH, Sun W, Cleary PA, et al. Prolonged effect of intensive therapy on the risk of retinopathy complications in patients with type 1 diabetes mellitus: 10 years after the Diabetes Control and Complications Trial. Arch Ophthalmol. 2008;126:1707–1715.
70 White NH, Cleary PA, Dahms W, et al. Beneficial effects of intensive therapy of diabetes during adolescence: outcomes after the conclusion of the Diabetes Control and Complications Trial (DCCT). J Pediatr. 2001;139:804–812.
71 Davis MD, Fisher MR, Gangnon RE, et al. Risk factors for high-risk proliferative diabetic retinopathy and severe visual loss: Early Treatment Diabetic Retinopathy Study (ETDRS) report no. 18. Invest Ophthalmol Vis Sci. 1998;39:233–252.
72 Early worsening of diabetic retinopathy in the Diabetes Control and Complications Trial. Arch Ophthalmol. 1998;116:874–886.
73 Dahl-Jorgensen K, Brinchmann-Hansen O, Hanssen KF, et al. Rapid tightening of blood glucose control leads to transient deterioration of retinopathy in insulin dependent diabetes mellitus: the Oslo study. Br Med J (Clin Res Ed). 1985;290:811–815.
74 Dandona P, Bolger JP, Boag F, et al. Rapid development and progression of proliferative retinopathy after strict diabetic control. Br Med J (Clin Res Ed). 1985;290:895–896.
75 Funatsu H, Yamashita H, Ohashi Y, et al. Effect of rapid glycemic control on progression of diabetic retinopathy. Jpn J Ophthalmol. 1992;36:356–367.
76 Lauritzen T, Frost-Larsen K, Larsen HW, et al. Two-year experience with continuous subcutaneous insulin infusion in relation to retinopathy and neuropathy. Diabetes. 1985;34(Suppl 3):74–79.
77 Brinchmann-Hansen O, Dahl-Jorgensen K, Hanssen KF, et al. The response of diabetic retinopathy to 41 months of multiple insulin injections, insulin pumps, and conventional insulin therapy. Arch Ophthalmol. 1988;106:1242–1246.
78 Blood glucose control and the evolution of diabetic retinopathy and albuminuria. A preliminary multicenter trial. The Kroc Collaborative Study Group. N Engl J Med. 1984;311:365–372.
79 Agardh CD, Eckert B, Agardh E. Irreversible progression of severe retinopathy in young type I insulin-dependent diabetes mellitus patients after improved metabolic control. J Diabetes Complications. 1992;6:96–100.
80 Ernest JT, Goldstick TK, Engerman RL. Hyperglycemia impairs retinal oxygen autoregulation in normal and diabetic dogs. Invest Ophthalmol Vis Sci. 1983;24:985–989.
81 Grunwald JE, Brucker AJ, Braunstein SN, et al. Strict metabolic control and retinal blood flow in diabetes mellitus. Br J Ophthalmol. 1994;78:598–604.
82 Grunwald JE, Brucker AJ, Schwartz SS, et al. Diabetic glycemic control and retinal blood flow. Diabetes. 1990;39:602–607.
83 Bain SC, Gill GV, Dyer PH, et al. Characteristics of type 1 diabetes of over 50 years duration (the Golden Years Cohort). Diabet Med. 2003;20:808–811.
84 Keenan HA, Costacou T, Sun JK, et al. Clinical factors associated with resistance to microvascular complications in diabetic patients of extreme disease duration: the 50-year medalist study. Diabetes Care. 2007;30:1995–1997.
85 Sun JK, Keenan HA, Cavallerano JD, et al. Protection from retinopathy and other complications in patients with type 1 diabetes of extreme duration: the Joslin 50-year medalist study. Diabetes Care.. 2011;34(4):968–974.
86 Ismail-Beigi F, Craven T, Banerji MA, et al. Effect of intensive treatment of hyperglycaemia on microvascular outcomes in type 2 diabetes: an analysis of the ACCORD randomised trial. Lancet. 2010;376:419–430.
87 Patel A, MacMahon S, Chalmers J, et al. Effects of a fixed combination of perindopril and indapamide on macrovascular and microvascular outcomes in patients with type 2 diabetes mellitus (the ADVANCE trial): a randomised controlled trial. Lancet. 2007;370:829–840.
88 Chew EY, Ambrosius WT, Davis MD, et al. Effects of medical therapies on retinopathy progression in type 2 diabetes. N Engl J Med. 2010;363:233–244.
89 Keech AC, Mitchell P, Summanen PA, et al. Effect of fenofibrate on the need for laser treatment for diabetic retinopathy (FIELD study): a randomised controlled trial. Lancet. 2007;370:1687–1697.
90 Chaturvedi N, Sjolie AK, Stephenson JM, et al. Effect of lisinopril on progression of retinopathy in normotensive people with type 1 diabetes. The EUCLID Study Group. EURODIAB controlled trial of lisinopril in insulin-dependent diabetes mellitus. Lancet. 1998;351:28–31.
91 Chaturvedi N, Porta M, Klein R, et al. Effect of candesartan on prevention (DIRECT-Prevent 1) and progression (DIRECT-Protect 1) of retinopathy in type 1 diabetes: randomised, placebo-controlled trials. Lancet. 2008;372:1394–1402.
92 Sjolie AK, Klein R, Porta M, et al. Effect of candesartan on progression and regression of retinopathy in type 2 diabetes (DIRECT-Protect 2): a randomised placebo-controlled trial. Lancet. 2008;372:1385–1393.
93 Mauer M, Zinman B, Gardiner R, et al. Renal and retinal effects of enalapril and losartan in type 1 diabetes. N Engl J Med. 2009;361:40–51.
94 Pershadsingh HA, Moore DM. PPARgamma agonists: potential as therapeutics for neovascular retinopathies. PPAR Res. 2008;2008:164273.
95 Shen LQ, Child A, Weber GM, et al. Rosiglitazone and delayed onset of proliferative diabetic retinopathy. Arch Ophthalmol. 2008;126:793–799.
96 Panigrahy D, Singer S, Shen LQ, et al. PPARgamma ligands inhibit primary tumor growth and metastasis by inhibiting angiogenesis. J Clin Invest. 2002;110:923–932.
97 Chew EY, Klein ML, Ferris FL, III., et al. Association of elevated serum lipid levels with retinal hard exudate in diabetic retinopathy. Early Treatment Diabetic Retinopathy Study (ETDRS) report no. 22. Arch Ophthalmol. 1996;114:1079–1084.
98 Biasotti A, Houssay BA. Phlorrhizin diabetes in fasting or fed hypophysectomized dogs. J Physiol. 1932;77:81–91.
99 Luft R, Olivecrona H, Sjogren B. [Hypophysectomy in man.]. Nord Med. 1952;47:351–354.
100 Poulsen JE. Recovery from retinopathy in a case of diabetes with Simmonds’ disease. Diabetes. 1953;2:7–12.
101 Poulsen JE. Diabetes and anterior pituitary insufficiency. Final course and postmortem study of a diabetic patient with Sheehan’s syndrome. Diabetes. 1966;15:73–77.
102 Kohner EM, Joplin GF, Blach RK, et al. Pituitary ablation in the treatment of diabetic retinopathy. (A randomized trial). Trans Ophthalmol Soc U K. 1972;92:79–90.
103 Panisset A, Kohner EM, Cheng H, et al. Diabetic retinopathy: new vessels arising from the optic disc. II. Response to pituitary ablation by yttrium 90 implant. Diabetes. 1971;20:824–833.
104 Kohner EM, Hamilton AM, Joplin GF, et al. Florid diabetic retinopathy and its response to treatment by photocoagulation or pituitary ablation. Diabetes. 1976;25:104–110.
105 Smith LE, Shen W, Perruzzi C, et al. Regulation of vascular endothelial growth factor-dependent retinal neovascularization by insulin-like growth factor-1 receptor. Nat Med. 1999;5:1390–1395.
106 Growth Hormone Antagonist for Proliferative Diabetic Retinopathy Study Group (2001). The effect of a growth hormone receptor antagonist drug on proliferative diabetic retinopathy. Ophthalmology. 2001;108:2266–2272.
107 Boehm BO, Lang GK, Jehle PM, et al. Octreotide reduces vitreous hemorrhage and loss of visual acuity risk in patients with high-risk proliferative diabetic retinopathy. Horm Metab Res. 2001;33:300–306.
108 Beetham WP. Visual prognosis of proliferating diabetic retinopathy. Br J Ophthalmol. 1963;47:611–619.
109 Aiello LM, Beetham WP, Balodimos MC, et al. Ruby laser photocoagulation in treatment of diabetic proliferating retinopathy: Preliminary report. In: Goldberg MF, Fine SL, editors. Symposium on the treatment of diabetic retinopathy. Arlington, VA: U S Department of Health, Education, and Welfare, 1968. pp. 437–63.
110 Meyer-Schwickerath G. [Light coagulation; a method for treatment and prevention of the retinal detachment.]. Albrecht Von Graefes Arch Ophthalmol. 1954;156:2–34.
111 Okun E, Cibis PA. The role of photocoagulation in the therapy of proliferative diabetic retinopathy. Arch Ophthalmol. 1966;75:337–352.
112 Wetzig PC, Jepson CN. Treatment of diabetic retinopathy by light coagulation. Am J Ophthalmol. 1966;62:459–465.
113 Wetzig PC, Worlton JT. Treatment of diabetic retinopathy by light-coagulation: a preliminary study. Br J Ophthalmol. 1963;47:539–541.
114 Beetham WP, Aiello LM, Balodimos MC, et al. Ruby-laser photocoagulation of early diabetic neovascular retinopathy: preliminary report of a long-term controlled study. Trans Am Ophthalmol Soc. 1969;67:39–67.
115 Beetham WP, Aiello LM, Balodimos MC, et al. Ruby laser photocoagulation of early diabetic neovascular retinopathy. Preliminary report of a long-term controlled study. Arch Ophthalmol. 1970;83:261–272.
116 Meyer-Schwickerath RE, Schott K. Diabetic retinopathy and photocoagulation. Am J Ophthalmol. 1968;66:597–603.
117 Okun E. The effectiveness of photocoagulation in the therapy of proliferative diabetic retinopathy (PDR). (A controlled study in 50 patients. Trans Am Acad Ophthalmol Otolaryngol. 1968;72:246–252.
118 Glaser BM, Campochiaro PA, Davis JL, Jr., et al. Retinal pigment epithelial cells release an inhibitor of neovascularization. Arch Ophthalmol. 1985;103:1870–1875.
119 Ederer F, Hiller R. Clinical trials, diabetic retinopathy and photocoagulation. A reanalysis of five studies. Surv Ophthalmol. 1975;19:267–286.
120 Stefansson E, Hatchell DL, Fisher BL, et al. Panretinal photocoagulation and retinal oxygenation in normal and diabetic cats. Am J Ophthalmol. 1986;101:657–664.
121 Gerstein DD, Dantzker DR. Retinal vascular changes in hereditary visual cell degeneration. Arch Ophthalmol. 1969;81:99–105.
122 Molnar I, Poitry S, Tsacopoulos M, et al. Effect of laser photocoagulation on oxygenation of the retina in miniature pigs. Invest Ophthalmol Vis Sci. 1985;26:1410–1414.
123 Weiter JJ, Zuckerman R. The influence of the photoreceptor-RPE complex on the inner retina. An explanation for the beneficial effects of photocoagulation. Ophthalmology. 1980;87:1133–1139.
124 Wolbarsht ML, Landers MB, III. The rationale of photocoagulation therapy for proliferative diabetic retinopathy: a review and a model. Ophthalmic Surg. 1980;11:235–245.
125 Grunwald JE, Riva CE, Brucker AJ, et al. Altered retinal vascular response to 100% oxygen breathing in diabetes mellitus. Ophthalmology. 1984;91:1447–1452.
126 Patel V, Rassam S, Newsom R, et al. Retinal blood flow in diabetic retinopathy. BMJ. 1992;305:678–683.
127 Stefansson E, Machemer R, de Juan E, Jr., et al. Retinal oxygenation and laser treatment in patients with diabetic retinopathy. Am J Ophthalmol. 1992;113:36–38.
128 Cheng H. Multicentre trial of xenon-arc photocoagulation in the treatment of diabetic retinopathy. A Randomized controlled study. Interim report. Trans Ophthalmol Soc U K. 1975;95:351–357.
129 Editorial: The diabetic retinopathy study. Arch Ophthalmol. 1973;90:347–348.
130 Diabetic Retinopathy Study Research Group. Photocoagulation treatment of proliferative diabetic retinopathy. Clinical application of Diabetic Retinopathy Study (DRS) findings. Diabetic Retinopathy Study report 8. Ophthalmology. 1981;88:583–600.
131 Diabetic Retinopathy Study Research Group. Photocoagulation treatment of proliferative diabetic retinopathy: relationship of adverse treatment effects to retinopathy severity. Diabetic Retinopathy Study report 5. Dev Ophthalmol. 1981;2:248–261.
132 Rand LI, Prud’homme GJ, Ederer F, et al. Factors influencing the development of visual loss in advanced diabetic retinopathy. Diabetic Retinopathy Study (DRS) report 10. Invest Ophthalmol Vis Sci. 1985;26:983–991.
133 Little HL, Rosenthal AR, Dellaporta A, et al. The effect of pan-retinal photo-coagulation on rubeosis iridis. Am J Ophthalmol. 1976;81:804–809.
134 Okun E, Johnston GP, Boniuk I, et al. Xenon arc photocoagulation of proliferative diabetic retinopathy. A review of 2688 consecutive eyes in the format of the Diabetic Retinopathy Study. Ophthalmology. 1984;91:1458–1463.
135 British Multicentre Study Group. Photocoagulation for proliferative diabetic retinopathy: a randomised controlled clinical trial using the xenon-arc. Diabetologia. 1984;26:109–115.
136 Hercules BL, Gayed II, Lucas SB, et al. Peripheral retinal ablation in the treatment of proliferative diabetic retinopathy: a three-year interim report of a randomised, controlled study using the argon laser. Br J Ophthalmol. 1977;61:555–563.
137 Early Treatment Diabetic Retinopathy Study Research Group. Early photocoagulation for diabetic retinopathy. ETDRS report no. 9. Ophthalmology. 1991;98:766–785.
138 Early Treatment Diabetic Retinopathy Study Research Group. Photocoagulation for diabetic macular edema. ETDRS report no. 1. Arch Ophthalmol. 1985;103:1796–1806.
139 Early Treatment Diabetic Retinopathy Study Research Group. Effects of aspirin treatment on diabetic retinopathy. ETDRS report no. 8. Ophthalmology. 1991;98:757–765.
140 Early Treatment Diabetic Retinopathy Study Research Group. Techniques for scatter and local photocoagulation treatment of diabetic retinopathy. ETDRS report no. 3. Int Ophthalmol Clin. 1987;27:254–264.
141 Ferris F. Early photocoagulation in patients with either type I or type II diabetes. Trans Am Ophthalmol Soc. 1996;94:505–537.
142 Chey EY for the Krypton Argon Regression Neovascularization Study Research Group. Randomized comparison of krypton versus argon scatter photocoagulation for diabetic disc neovascularization: KARNS Study report number 1. Ophthalmology. 1993;100:1655–1664.
143 Meyer-Schwickerath G, Gerke E. Bjerrum lecture. Treatment of diabetic retinopathy with photocoagulation. Results of photocoagulation therapy of proliferative retinopathy in childhood-onset and maturity-onset diabetes and an approach to the dosage in photocoagulation. Acta Ophthalmol (Copenh). 1983;61:756–768.
144 Chew EY, Mills JL, Metzger BE, et al. Metabolic control and progression of retinopathy. The Diabetes in Early Pregnancy Study. National Institute of Child Health and Human Development Diabetes in Early Pregnancy Study. Diabetes Care. 1995;18:631–637.
145 Klein BE, Moss SE, Klein R. Effect of pregnancy on progression of diabetic retinopathy. Diabetes Care. 1990;13:34–40.
146 Mathiesen ER, Ronn B, Storm B, et al. The natural course of microalbuminuria in insulin-dependent diabetes: a 10-year prospective study. Diabet Med. 1995;12:482–487.
147 McDonald HR, Schatz H. Macular edema following panretinal photocoagulation. Retina. 1985;5:5–10.
148 McDonald HR, Schatz H. Visual loss following panretinal photocoagulation for proliferative diabetic retinopathy. Ophthalmology. 1985;92:388–393.
149 Meyers SM. Macular edema after scatter laser photocoagulation for proliferative diabetic retinopathy. Am J Ophthalmol. 1980;90:210–216.
150 Ferris FL, III., Podgor MJ, Davis MD. Macular edema in diabetic retinopathy study patients. Diabetic Retinopathy Study report number 12. Ophthalmology. 1987;94:754–760.
151 Early Treatment Diabetic Retinopathy Study Research Group. Treatment techniques and clinical guidelines for photocoagulation of diabetic macular edema. ETDRS report no. 2. Ophthalmology. 1987;94:761–774.
152 Elman MJ, Aiello LP, Beck RW, et al. Randomized trial evaluating ranibizumab plus prompt or deferred laser or triamcinolone plus prompt laser for diabetic macular edema. Ophthalmology. 2010;117:1064–1077.
153 Sultan MB, Zhou D, Loftus J, et al. A phase 2/3, multicenter, randomized, double-masked, 2-year trial of pegaptanib sodium for the treatment of diabetic macular edema. Ophthalmology. 2011;118:1107–1118.
154 Arevalo JF, Sanchez JG, Fromow-Guerra J, et al. Comparison of two doses of primary intravitreal bevacizumab (Avastin) for diffuse diabetic macular edema: results from the Pan-American Collaborative Retina Study Group (PACORES) at 12-month follow-up. Graefes Arch Clin Exp Ophthalmol. 2009;247:735–743.
155 Googe J, Brucker AJ, Bressler NM, et al. for the Diabetic Retinopathy Clinical Research Network. Randomized trial evaluating short-term effects of intravitreal ranibizumab or triamcinolone acetonide on macular edema after focal/grid laser for diabetic macular edema in eyes also receiving panretinal photocoagulation. Retina. 2011;31(6):1009–1027.
156 Shimura M, Yasuda K, Nakazawa T, et al. Quantifying alterations of macular thickness before and after panretinal photocoagulation in patients with severe diabetic retinopathy and good vision. Ophthalmology. 2003;110:2386–2394.
157 Diabetic Retinopathy Clinical Research NetworkBrucker AJ, Qin H, Antoszyk AN, et al. Observational study of the development of diabetic macular edema following panretinal (scatter) photocoagulation given in 1 or 4 sittings. Arch Ophthalmol. 2009;127:132–140.
158 Pavan PR, Folk JC, Weingeist TA, et al. Diabetic rubeosis and panretinal photocoagulation. Arch Ophthalmol. 1983;101:882–884.
159 Blumenkranz MS, Yellachich D, Andersen DE, et al. Semiautomated patterned scanning laser for retinal photocoagulation. Retina. 2006;26:370–376.
160 Jain A, Blumenkranz MS, Paulus Y, et al. Effect of pulse duration on size and character of the lesion in retinal photocoagulation. Arch Ophthalmol. 2008;126:78–85.
161 Muqit MM, Sanghvi C, McLauchlan R, et al. Study of clinical applications and safety for Pascal((R)) laser photocoagulation in retinal vascular disorders. Acta Ophthalmol 2010. Epub ahead of print February 16, 2010; PMID 20163363
162 Velez-Montoya R, Guerrero-Naranjo JL, Gonzalez-Mijares CC, et al. Pattern scan laser photocoagulation: safety and complications, experience after 1301 consecutive cases. Br J Ophthalmol. 2010;94:720–724.
163 Kriechbaum K, Bolz M, Deak GG, et al. High-resolution imaging of the human retina in vivo after scatter photocoagulation treatment using a semiautomated laser system. Ophthalmology. 2010;117:545–551.
164 Liang JC, Huamonte FU. Reduction of immediate complications after panretinal photocoagulation. Retina. 1984;4:166–170.
165 Doft BH, Blankenship GW. Single versus multiple treatment sessions of argon laser panretinal photocoagulation for proliferative diabetic retinopathy. Ophthalmology. 1982;89:772–779.
166 Bandello F, Brancato R, Lattanzio R, et al. Double-frequency Nd:YAG laser vs argon-green laser in the treatment of proliferative diabetic retinopathy: randomized study with long-term follow-up. Lasers Surg Med. 1996;19:173–176.
167 Bandello F, Brancato R, Trabucchi G, et al. Diode versus argon-green laser panretinal photocoagulation in proliferative diabetic retinopathy: a randomized study in 44 eyes with a long follow-up time. Graefes Arch Clin Exp Ophthalmol. 1993;231:491–494.
168 Doft BH, Blankenship G. Retinopathy risk factor regression after laser panretinal photocoagulation for proliferative diabetic retinopathy. Ophthalmology. 1984;91:1453–1457.
169 Blankenship GW. A clinical comparison of central and peripheral argon laser panretinal photocoagulation for proliferative diabetic retinopathy. Ophthalmology. 1988;95:170–177.
170 Vander JF, Duker JS, Benson WE, et al. Long-term stability and visual outcome after favorable initial response of proliferative diabetic retinopathy to panretinal photocoagulation. Ophthalmology. 1991;98:1575–1579.
171 Rogell GD. Incremental panretinal photocoagulation. Results in treating proliferative diabetic retinopathy. Retina. 1983;3:308–311.
172 Reddy VM, Zamora RL, Olk RJ. Quantitation of retinal ablation in proliferative diabetic retinopathy. Am J Ophthalmol. 1995;119:760–766.
173 Vine AK. The efficacy of additional argon laser photocoagulation for persistent, severe proliferative diabetic retinopathy. Ophthalmology. 1985;92:1532–1537.
174 Early Treatment Diabetic Retinopathy Study Research Group. Case reports to accompany Early Treatment Diabetic Retinopathy Study reports 3 and 4. Int Ophthalmol Clin. 1987;27:273–333.
175 Dogru M, Kaderli B, Gelisken O, et al. Ocular surface changes with applanation contact lens and coupling fluid use after argon laser photocoagulation in noninsulin-dependent diabetes mellitus. Am J Ophthalmol. 2004;138:381–388.
176 Bloom SM, Mahl CF, Schiller SB. Lenticular burns following argon panretinal photocoagulation. Br J Ophthalmol. 1992;76:630–631.
177 Moriarty AP, Spalton DJ, Shilling JS, et al. Breakdown of the blood-aqueous barrier after argon laser panretinal photocoagulation for proliferative diabetic retinopathy. Ophthalmology. 1996;103:833–838.
178 Patel JI, Jenkins L, Benjamin L, et al. Dilated pupils and loss of accommodation following diode panretinal photocoagulation with sub-tenon local anaesthetic in four cases. Eye (Lond). 2002;16:628–632.
179 Fong DS, Girach A, Boney A. Visual side effects of successful scatter laser photocoagulation surgery for proliferative diabetic retinopathy: a literature review. Retina. 2007;27:816–824.
180 Mendrinos E, Donati G, Pournaras CJ. Rapid and persistent regression of severe new vessels on the disc in proliferative diabetic retinopathy after a single intravitreal injection of pegaptanib. Acta Ophthalmol. 2009;87:683–684.
181 Avery RL, Pearlman J, Pieramici DJ, et al. Intravitreal bevacizumab (Avastin) in the treatment of proliferative diabetic retinopathy. Ophthalmology. 2006;113:1695.e1–1695.e15.
182 Jorge R, Costa RA, Calucci D, et al. Intravitreal bevacizumab (Avastin) for persistent new vessels in diabetic retinopathy (IBEPE study). Retina. 2006;26:1006–1013.
183 Erdol H, Turk A, Akyol N, et al. The results of intravitreal bevacizumab injections for persistent neovascularizations in proliferative diabetic retinopathy after photocoagulation therapy. Retina. 2010;30:570–577.
184 Mirshahi A, Roohipoor R, Lashay A, et al. Bevacizumab-augmented retinal laser photocoagulation in proliferative diabetic retinopathy: a randomized double-masked clinical trial. Eur J Ophthalmol. 2008;18:263–269.
185 Tonello M, Costa RA, Almeida FP, et al. Panretinal photocoagulation versus PRP plus intravitreal bevacizumab for high-risk proliferative diabetic retinopathy (IBeHi study). Acta Ophthalmol. 2008;86:385–389.
186 Takahashi M, Trempe CL, Maguire K, et al. Vitreoretinal relationship in diabetic retinopathy. A biomicroscopic evaluation. Arch Ophthalmol. 1981;99:241–245.
187 Ono R, Kakehashi A, Yamagami H, et al. Prospective assessment of proliferative diabetic retinopathy with observations of posterior vitreous detachment. Int Ophthalmol. 2005;26:15–19.
188 Rheaume MA, Vavvas D. Pharmacologic vitreolysis. Semin Ophthalmol. 2010;25:295–302.
189 Gandorfer A. Objective of pharmacologic vitreolysis. Dev Ophthalmol. 2009;44:1–6.
190 Kuppermann BD, Thomas EL, de Smet MD, et al. Pooled efficacy results from two multinational randomized controlled clinical trials of a single intravitreous injection of highly purified ovine hyaluronidase (Vitrase) for the management of vitreous hemorrhage. Vitrase for Vitreous Hemorrhage Study Groups. Am J Ophthalmol. 2005;140:573–584.
191 Stalmans P, Delaey C, de Smet MD, et al. Intravitreal injection of microplasmin for treatment of vitreomacular adhesion: results of a prospective, randomized, sham-controlled phase II trial (the MIVI-IIT trial). Retina. 2010;30:1122–1127.
192 Goldenberg DT, Trese MT. Pharmacologic vitreodynamics and molecular flux. Dev Ophthalmol. 2009;44:31–36.
193 Machemer R, Buettner H, Norton EW, et al. Vitrectomy: a pars plana approach. Trans Am Acad Ophthalmol Otolaryngol. 1971;75:813–820.