Chapter 29 Prenatal Diagnosis of Structural Brain Anomalies
Introduction
The fetal brain develops during the course of pregnancy, from its primitive three-vesicle stage into a complex structure with an array of sulci and gyri resembling the adult brain by the end of gestation [Nadich et al., 1994]. Fetal brain development is characterized by well-defined, genetically programmed, morphological and maturational changes, including neuronal migration, sulcation, and myelination that can be studied by ultrasound, starting from gestational week 6–7 until delivery [Ertl-Wagner et al., 2002]. Fetal magnetic resonance imaging (MRI) enables good demonstration of anatomy, particularly of cortical development and diagnosis of diverse anomalies, starting at around 18–20 weeks of pregnancy.
Sonography is the most important imaging method for prenatal malformation screening. It is noninvasive, widely available, and safe for both mother and child. The ultrasonographic approach to the evaluation of the fetal brain is based on the transabdominal visualization of three different axial planes: the transventricular, the transthalamic, and the transcerebellar (Figure 29-1). The use of these three planes enables good visualization of the lateral ventricles but is not sufficient for depiction of malformations of the cortex and of midline brain structures, mainly the corpus callosum, the third ventricle, and the cerebellar vermis [Malinger et al., 2007]. The use of a more comprehensive, multiplanar approach, in which coronal and sagittal planes are added to the classical axial planes, and addition of a transvaginal or transfundal approach overcome these limitations [Malinger et al., 1993] (Figure 29-2 and Figure 29-3).
Fetal MRI is used primarily to confirm and characterize brain abnormalities detected by routine prenatal sonography. More than 25 years after its introduction into fetal imaging, MRI is considered a valuable complementary tool in the imaging of structural anomalies of the fetal brain [Guibaud, 2009; Sonigo et al., 1998].
Fetal MRI is performed primarily using ultrafast MRI techniques known as single-shot, fast spin-echo (SS-FSE) or half-Fourier acquired single-shot turbo spin-echo (HASTE). Using these rapid-pulse sequences, a single T2-weighted image can be acquired in less than 1 second, reducing the likelihood of fetal motion during image acquisition [Brugger et al., 2006; Glenn and Barkovich, 2006]. When the fetal brain is being evaluated, images are obtained with a section thickness of 3 mm with no gap, in axial, sagittal, and coronal planes. Good orthogonal planes may not always be obtainable.
T1-weighted imaging and fast multiplanar gradient recalled-echo techniques, such as FMPSPGR (fast multiplanar spoiled gradient recalled acquisition in the steady state), are primarily used to detect hemorrhage or calcification [Glenn and Barkovich, 2006].
With the recent application of advanced MRI techniques, an opportunity to investigate the developing brain is available beyond the qualitative morphologic evaluation [Limperopoulos and Clouchoux, 2009; Glenn, 2009]. Diffusion-weighted imaging (DWI) and diffusion tensor imaging (DTI) are used to evaluate maturation-dependent microstructural changes associated with brain growth and development of cerebral white matter. DWI provides quantitative information about water motion and tissue microstructure, and provides additional applications for destructive brain processes. DTI allows the visualization and quantification of white-matter fiber direction. Advanced postprocessing techniques, such as the formation of high-resolution three-dimensional structural images, can be used to study morphometry [Glenn, 2009]. MR spectroscopy can be applied in the third trimester for the study of cerebral metabolism in vivo, since metabolites such as N-acetyl aspartate, creatine, and choline can be detected [Limperopoulos and Clouchoux, 2009].
Prenatal Assessment of Normal Brain Development in the First Trimester
Brain development in the embryonic phase is only assessed by ultrasound. Blaas and Eik-Nes [2009] describe the milestones of normal brain development between 7 and 12 weeks’ gestation based on longitudinal two-dimensional and three-dimensional ultrasound studies. Cortical development starts at about 7 weeks’ gestation and other brain structures develop synchronously with the cortex, including the commissures and the cerebellum. At 7–8 weeks, the lateral ventricles are seen as separated, small, round hypoechogenic brain cavities, and the future third ventricle runs posteriorly. The curved tube-like mesencephalic cavity (future sylvian aqueduct) lies anteriorly, and the broad and shallow rhombencephalic cavity is in the cranial pole of the embryo (Figure 29-4).
By the end of the first trimester (weeks 10–12), the cortex is about 1–2 mm thick and the crescent hemispheres fill the anterior part of the head and conceal the diencephalic cavity. The lateral ventricles are large and filled by the echogenic choroid plexuses. The insula appears as a slight depression on the lateral surface of the hemispheres. The diencephalon lies between the hemispheres, and the mesencephalon gradually moves towards the center of the head. The width of the third ventricle narrows. The corpus callosum is not yet visible. The cerebellar hemispheres seem to meet in the midline [Blaas and Eik-Nes, 2009].
Prenatal Assessment of Normal Development of the Cortex
Initially, the brain surface is smooth. Gyration can be observed by the second month of intrauterine life. It continues to the end of the pregnancy and even later after birth. The primary sulci appear as shallow grooves on the surface of the brain that become progressively more deeply infolded and then develop side branches, designated secondary sulci. Gyration proceeds with the formation of other side branches of the secondary sulci, referred to as tertiary sulci [Nadich et al., 1994]. The major fissures and sulci develop in predictable patterns, starting at about 16 weeks [Dorovini-Zis and Dolman, 1997], and therefore the timing of their appearance is a reliable estimate of gestational age. Anatomical changes precede ultrasound and MRI detection by about 2 weeks [Levine and Barnes, 1999] (Table 29-1).
Table 29-1 Chronology of Sulcation According to Neuropathological, Sonographic, and Magnetic Resonance Imaging Studies
Several studies [Toi et al., 2004; Cohen-Sacher et al., 2006] have assessed the development of the fetal cortex by ultrasound. Major sulci can be discerned, starting at about 18–19 weeks’ gestation. At 18–20 weeks, the brain surface is almost completely smooth, with only the major fissures present. The callosal sulcus is observed between 18 and 20 weeks. Between 22 and 26 weeks, the calcarine fissure, the cingulate sulcus, and the central sulcus gradually appear. The most active period of cortical development, as demonstrated by ultrasound, is between 28 and 30 weeks. During this time, most sulci become detectable. After 32 weeks, all structures, other than the insular and olfactory sulci, are present. During the last 4 weeks of pregnancy, the sulci over the convexity are best demonstrated using sagittal planes [Cohen-Sacher et al., 2006] (see Table 29-1). Early sulci are easier to evaluate by ultrasound than later-developing complex secondary and tertiary patterns [Malinger et al., 2006].
MRI after about 20 weeks of gestation provides excellent information unimpeded by calvarial calcification. Similar to ultrasound, it shows age-related predictable patterns of development, but, in addition, brain parenchyma and myelination can be evaluated [Garel et al., 2001].
Brain MRI can depict the walls of the ventricles, which are critical to normal brain development. They are lined by the ventricular zone (germinal matrix), which is very thick earlier in gestation, and appears as a smooth band of dark T2 signal and bright T1 signal lining the lateral ventricles. The cerebral mantle is seen on fetal MRI until about 28 weeks of gestation in a multilayered pattern [Kostovic and Vasung, 2009]. When viewed from the ventricular margin to the pial surface of the brain, five layers can be demonstrated (Figure 29-5).
The development of the cortex can be visualized from midpregnancy. On MRI, gyration starts with the appearance of a shallow indentation of the fetal brain in the temporal regions at the 18th gestational week. At the same time, the anterior cingular fissure and the parieto-occipital fissure occur. The central sulcus begins to be visible at 24 weeks’ gestation. Fissures then become deeper and tighter, and gyri bulge into the subarachnoid space. Until the 35th gestational week, all primary and most of the secondary sulci are present, with secondary sulci appearing from the 24th gestational week, and tertiary after the 28th gestational week [Garel et al., 2001; Prayer et al., 2006] (see Table 29-1; Figure 29-6).
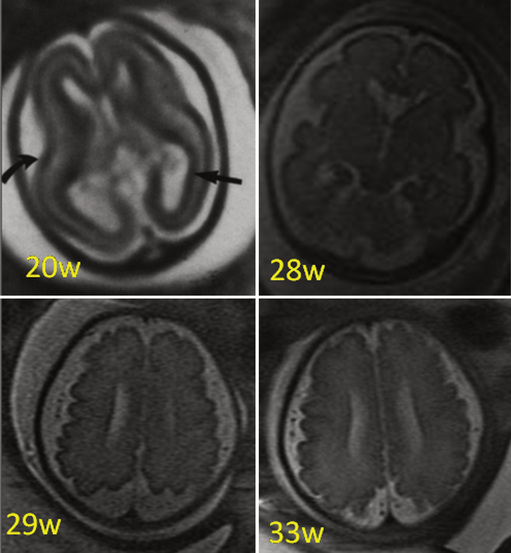
Fig. 29-6 Magnetic resonance imaging: axial images at different weeks of gestation.
Normal development of sulcation and normal “closure” of the insula are demonstrated.
The development of the sylvian fissure is one of the major brain maturational processes occurring in fetal life, and abnormalities in this process are frequent in children with developmental delay. The appearance and shape of the sylvian fissure can be evaluated both by ultrasound and MRI [Lerman-Sagie and Malinger, 2008; Garel et al., 2001; Chen et al., 1995]. It is observed in all fetuses at 22–23 weeks’ gestation as a shallow depression at the surface of the brain, and the insula is wide open at this time. The temporal lobe folds over to engulf the insula, and the progressive closure begins posteriorly and extends anteriorly. By 33 weeks’ gestation, insular sulci are recognizable and the surface of the Sylvian fissure becomes more and more indented [Garel et al., 2001; Quarello et al., 2008].
Prenatal Assessment of Normal Development of the Corpus Callosum
The corpus callosum develops between gestational weeks 8 and 20 as axons from the developing cerebral hemispheres navigate to and through the hemispheres and interhemispheric fissure. The corpus callosum is difficult to visualize by ultrasound during the performance of routine transabdominal evaluations using axial planes. For a complete demonstration of this structure, it is necessary to obtain sagittal planes through the anterior or posterior fontanels or the sagittal suture with the transvaginal or transfundal approach. Coronal planes are useful for studying the relationship between the corpus callosum and neighboring structures. The completely formed corpus callosum may be depicted using high-resolution transducers by 18–20 weeks of gestation, as an arched hypoechogenic structure delimited by the more echogenic callosal sulcus cranially and the anechogenic cavum septi pelucidi caudally. At this age, its shape is already very similar to that of the mature corpus callosum, and the genu, body, and splenium can be well demonstrated (see Figures 29-2 and 29-3). The corpus callosum develops in a linear pattern during pregnancy, its length progressively increasing from 20 mm at 20 weeks to 40 mm close to term. Nomograms of callosal growth during pregnancy, including length and thickness, can be used to assess normal development [Malinger et al., 1993].
The corpus callosum can be depicted by MRI on midline sagittal T2 images by gestational week 20 as a band of low signal intensity superior to the fornix (Figure 29-7). It can also be seen on coronal T2 images. It has fairly uniform thickness [Toi et al., 2009].
Prenatal Assessment of Normal Development of the Posterior Fossa
Both ultrasound [Malinger et al., 2001] and MRI [Glenn, 2009; Adamsbaum et al., 2005] can evaluate the development of the fetal cerebellum.
The ultrasonographic evaluation should include multiplanar images of the cerebellum. The axial plane is useful for determining the transcerebellar diameter, cisterna magna size, and the cerebellar peduncle’s thickness. The coronal plane enables differentiation between the cerebellar hemispheres and the vermis. The midsagittal plane is the most important plane to be investigated, since it allows depiction of the vermian lobules, fissures, and shape of the fastigium; measurements of the vermian diameter and surface; and calculation of the ratio between the superior and inferior parts. This plane also permits evaluation of the size and shape of the pons, cisterna magna, and tentorium [Malinger et al., 2001].
The fourth ventricle is uniformly observed as a triangular structure anterocaudal to the vermis. The inferior lobe of the vermis and the nodulus separate the fourth ventricle and the cistern magna [Malinger et al., 2001].
MRI is highly accurate in illustrating the morphologic MRI biometry of cerebellar development [Adamsbaum et al., 2005; Triulzi et al., 2005]. The cerebellar vermis is best assessed by MRI on direct midline sagittal images and on coronal images; the measurements should be compared with established norms. The cerebellar hemispheres are best assessed on nonoblique axial and coronal views.
Fetal MRI shows gestational age-specific changes in signal intensity in the normal development and maturation of the cerebellar hemispheres and brainstem [Huisman et al., 2002]. The cerebellar cortex, dentate nucleus, tectum, dorsal pons, and medulla are T1-hyperintense and T2-hypointense. The changes in signal intensity in the brainstem and cerebellum are not encountered until 20–23 weeks of gestation. By 26–27 weeks of gestation, a three-layered pattern is noted in the cerebellar hemispheres, corresponding to the cerebellar cortex, cerebellar white matter, and dentate nucleus. Fetal brain MRI can show the fissures of the cerebellum, depending on the gestational age. The primary fissure is identified on sagittal images at 22 weeks but the cerebellar surface is smooth. From 24 to 29 weeks, foliation of the vermis and posterior lobes of the cerebellum is seen on sagittal images. The cerebellar surface is smooth, with the appearance of some indentations corresponding to the horizontal and secondary fissures on axial images. The convoluted pattern of the cerebellum is well identified from 30 weeks on and is always seen beyond 33 weeks. [Fogliarini et al., 2005a].
Posterior fossa anatomy is sufficiently well defined in order to diagnose most cerebellar abnormalities prenatally; however, there may be many pitfalls in the differentiation of normal and abnormal cerebellar development [Malinger et al., 2009; Limperopoulos et al., 2008].
Prenatal Diagnosis of Ventriculomegaly
The lateral ventricle should be measured by ultrasound in the axial plane, at the level of the frontal horns and cavum septi pellucidi, with the calipers positioned at the level of the internal margin of the medial and lateral wall of the atria, at the level of the glomus of the choroid plexus, on an axis perpendicular to the long axis of the lateral ventricle [ISUOG guidelines, 2007].
Ventriculomegaly is defined as a lateral ventricular width equal to or larger than 10 mm [ISUOG guidelines, 2007]. Fetal ventriculomegaly may be classified as mild when the lateral ventricular width is between 10 and 15 mm, and severe when larger than 15 mm; it may be unilateral or bilateral (Figure 29-8).
Ventriculomegaly is defined as isolated if there is no sonographic evidence of associated malformations or markers of aneuploidy at the time of the initial presentation. Isolated mild ventriculomegaly represents a diagnostic and counseling difficulty, as it can be an apparently benign finding, but can also be associated with chromosomal abnormalities, congenital infection, cerebral vascular accidents or hemorrhage, and other fetal cerebral and extracerebral abnormalities; it may also have implications regarding long-term neurodevelopmental outcome [Melchiorre et al., 2009]. Therefore, upon confirmation of ventriculomegal, a complete search for associated central nervous system and non-central nervous system anomalies should be attempted, including a study of the brain in a multiplanar approach with particular attention to the shape of the ventricles, periventricular white matter, sulcation, corpus callosum, and posterior fossa [Malinger et al., 2006]. The investigation should also include screening for in utero infection, amniocentesis, and fetal echocardiography. Many studies have indicated that MRI may add important information to that obtained by ultrasound imaging [Ouahba et al., 2006]. Information relevant enough to modify obstetric management can be obtained in 6–9 percent of cases, including cortical malformations, absence of the septum pellucidum, partial agenesis of the corpus callosum, and agenesis of the cerebellar vermis [Salomon et al., 2006; Valsky et al., 2004].
Follow-up examinations at 3–4-week intervals are indicated to assess progressive enlargement of ventricles or to diagnose associated pathologies not previously detected [Ouahba et al., 2006].
When mild ventriculomegaly is isolated, the outcome is usually good [Ouahba et al., 2006; Melchiorre et al., 2009]. However, the risk for abnormal outcome increases when there are associated anomalies, the ventriculomegaly is asymmetric, the atrial width is greater than 12 mm, or there is a progressive increase of the lateral ventricular width [Pilu et al., 1999; Sadan et al., 2007; Falip et al., 2007]. A false-negative diagnosis of associated anomalies is possible in up to 12.8 percent of cases [Melchiorre et al., 2009].
The outcome of severe ventriculomegaly depends mainly on the presence of associated pathologies. When associated pathologies are diagnosed, the prognosis is usually very poor. Even when isolated, the risk of perinatal death or severe neurologic sequelae is in the range of 50 percent of survivors [Kennelly et al., 2009].
Prenatal Diagnosis of Abnormalities of the Corpus Callosum
Developmental abnormalities of the corpus callosum include agenesis, hypogenesis (or partial agenesis), dysgenesis, hypoplasia, and secondary destruction [Glenn and Barkovich, 2006]. Agenesis of the corpus callosum (ACC) can be detected prenatally by routine sonography, for which the important signs include absence of the cavum septum pellucidum, colpocephaly, high-riding third ventricle, widening of the interhemispheric fissure, and radiating medial hemispheric sulci. Ultrasound can also depict an abnormally thick corpus callosum, which usually signifies a poor prognosis [Lerman-Sagie et al., 2009].
Fetal MRI is clinically helpful in suspected cases of ACC because it can confirm the absence of the corpus callosum and diagnose associated anomalies. Since the corpus callosum is not myelinated in utero, it can often be very difficult to distinguish on sagittal images, so agenesis of the corpus callosum can often be concluded from an altered shape of the lateral ventricles with straight anterior horns and colpocephalic posterior horns (Figure 29-9).
ACC is rarely isolated. Additional abnormalities occur frequently (93 percent). The most common findings are sulcation and posterior fossa abnormalities. Abnormal sulcal morphology can be detected as early as 19 gestational weeks. The gyral abnormalities include polymicrogyria, lissencephaly, pachygyria, schizencephaly, and other nonclassified abnormalities [Tang et al., 2009].
Interestingly, in 10 percent of fetuses with ACC, there may be evidence of destructive changes in the brain parenchyma, suggesting either an acquired etiology or a genetic/metabolic abnormality [Prasad et al., 2009].
Prenatal Diagnosis of Malformations of Cortical Development
Although the migration process terminates around the end of the first half of pregnancy, malformations of cortical development are seldom diagnosed in utero, possibly because the time of appearance of significant morphological changes is beyond the recommended time of the anatomic scan (19–23 weeks), and because ultrasound evaluation of the brain is often limited to visualization of the lateral ventricles and cerebellum [Malinger et al., 2004].
Abnormal sulcation landmarks can be observed as early as the 22nd week of gestation in fetuses suffering from Miller–Dicker and Walker–Warburg lissencephaly [Fong et al., 2004]. In patients with migration disorders, the ultrasound usually demonstrates one of the following patterns: delayed or premature appearance of sulcation; a thin and irregular cortical mantle; wide abnormal overdeveloped gyri; wide opening of isolated sulci, nodular bulging into the lateral ventricles, cortical clefts, and intraparenchymal echogenic nodules [Malinger et al., 2007; Malinger et al., 2006] (Figure 29-10).
MRI may identify cortical malformations more accurately, particularly late in pregnancy. The imaging signs suggestive of abnormal sulcation are mild ventriculomegaly associated with delayed cortical development, dysgenesis of the sylvian fissure, delayed sulcal appearance, callosal abnormality, cortical thickening, heterotopias, absence or abnormal appearance of fissure, irregular, abnormal, asymmetric gyri, and noncontinuous cortex in schizencephaly [Guibaud et al., 2008; Fong et al., 2004].
Chen et al. [1995] defined abnormal patterns of operculization and divided them into five types. Further studies [Quarello et al., 2008] have proven that, when the operculum is unformed or abnormally formed, the prognosis is usually poor and an underlying malformation of cortical development may be detected. When the operculum is underdeveloped for gestational age, it is usually associated with an abnormal head circumference or other brain anomalies. When it is an isolated finding, it may represent a metabolic disease, chromosomal anomaly, or benign delayed maturation of the operculum, usually associated with macrocephaly and enlargement of the subarachnoid spaces.
Prenatal Diagnosis of Lissencephaly Type I
Fetuses with lissencephaly type 1 or Miller–Dieker syndrome can be diagnosed after 27–30 weeks’ gestation when most of the primary sulci are already present, either by detailed neurosonography or MRI, by demonstration of dysgenesis of the sylvian fissure, delayed sulcal appearance, callosal abnormality, and cortical thickening [Saltzman et al., 1991; Greco et al., 1991] (see Figure 29-10C and Figure 29-11). Sometimes, zones of normal cortex and zones of pachygyric or agyric cortex alternate. The bilateral opercular dysplasia is responsible for a figure-eight-shaped brain [Fong et al., 2004].
Mild ventriculomegaly and delayed sulcal development are the earliest signs and can be diagnosed by 23 weeks’ gestation in fetuses at risk [Malinger et al., 2004]. Fetuses with Miller–Dieker syndrome have abnormal parieto-occipital and sylvian fissures by the time of the second-trimester ultrasound examination [Malinger et al., 2004]. Fluorescence in situ hybridization (FISH) or mutation analysis for 17p13.3 abnormalities helps confirm the diagnosis.
Prenatal Diagnosis of Cobblestone Complex/Lissencephaly Type 2
The prenatal diagnosis of Walker–Warburg syndrome has been reported in families at risk [Crowe et al., 1985; Rodgers et al., 1994]. An early diagnosis in the first trimester may be made if there is a cephalocele [Crowe et al., 1985]. Other findings suggestive of lissencephaly type 2 are early enlargement of the lateral ventricles, abnormal vermis, retinal detachment [Farrell et al., 1987], cataract, and abnormal sulcation [Monteagudo et al., 2001].
The presence of abnormal sulcation may only be demonstrated during the third trimester. MRI can better visualize the posterior fossa and gyration [Gasser et al., 1998; Kojima et al., 2002], and can demonstrate a kinked brainstem and bifid pons [Strigini et al., 2009; Mitchell et al., 2000] (see Figure 29-10B and Figure 29-12).
Prenatal Diagnosis of Periventricular Nodular Heterotopia
Periventricular nodular heterotopia should be considered when ultrasound depicts an irregular lateral ventricular wall with indentations of periventricular tissue and a signal similar to that of the cortex (see Figure 29-10A). The sonographic diagnosis is difficult, particularly when the lateral ventricle width is normal. MRI demonstrates multiple small nodular subependymal foci of low signal intensity, isointense to the germinal matrix, similar to that of the gray matter, located in the margins of the lateral ventricles (Figure 29-13). They cannot be distinguished reliably from the subependymal nodules seen in tuberous sclerosis; therefore, it is important to search for other manifestations of tuberous sclerosis, such as cortical hamartomas (which are hypointense compared with normal unmyelinated white matter) and cardiac rhabdomyomas [Glenn and Barkovich, 2006] A mega cisterna magna, which can be seen in females with filamin A mutations, may be the initial abnormality and, in association with periventricular nodules, may suggest the prenatal diagnosis of periventricular nodular heterotopia [Mitchell et al., 2000; Bargallo et al., 2002].
Heterotopia may be not recognized when the nodules are small or subcortical. Large nodules may be confused with tumoral or hemorrhagic masses [Onyeije et al., 1998].
Prenatal Diagnosis of Polymicrogyria
The cortical changes of polymicrogyria take place late in pregnancy and appear as localized and/or generalized absence of normal sulcation with multiple abnormal infoldings of the affected cortex [Glenn and Barkovich, 2006]. It is more apparent on MRI than ultrasound. In young fetuses (24 weeks), the identification of the cortical malformation is quite difficult and the manifestations in both ultrasound and MRI are subtle. They include presence of sulci that are not as expected, according to the gestational age; an irregular surface of the brain; and absence of the normal signal of the cortical ribbon (Figure 29-14). Late in pregnancy, the MRI features are similar to what is known in the postnatal period: packed and serrated microgyri, irregular cortex–white-matter junction, and an aberrant and asymmetrical sulcal pattern [Fogliarini et al., 2005a]. The most frequent MRI presentation diagnosed in utero demonstrates mild ventricular dilatation associated with numerous sulci in the perisylvian area, with irregular cortex–white-matter junction and a prominent subarachnoid space overlying the cortical malformation. Since the MRI pattern of polymicrogyria changes with increasing gestational age, it is important to follow young fetuses and repeat the ultrasound and MRI examinations in order to confirm the diagnosis.
Prenatal Diagnosis of Schizencephaly
Prenatal ultrasound enables diagnosis of schizencephaly, although prenatal MRI is more specific in detection of the gray matter lining the defect, communication with the ventricle, and other associated structural abnormalities [Oh et al., 2005]. The prenatal diagnosis of schizencephaly depends on the extent of cleft separation; closed-lip and open-lip variants with a very small gap remain undiagnosed. The prenatal diagnosis of schizencephaly has been described as early as 21 weeks. Fetuses are usually diagnosed after a routine ultrasound examination demonstrates enlarged lateral ventricles, fluid-filled cavities, or absence of the cavum septum pellucidum [Malinger et al., 2007]. Neurosonography demonstrates bilateral or unilateral open-lip wedge-shaped defects, usually in the parietotemporal regions, frequently accompanied by absence of the cavum septum pellucidum. Fetal MRI shows schizencephalic clefts extending from the pial surface to the ventricle lined with gray matter, which is seen as a low-signal-intensity line covering the edge of the remaining brain parenchyma (Figure 29-15). This finding allows differentiation of the defect from porencephaly [Oh et al., 2005; Denis et al., 2001].
Prenatal Diagnosis of Posterior Fossa Anomalies
Infratentorial anomalies are usually diagnosed in utero when associated with an enlarged cisterna magna, with or without an abnormal fourth ventricle. The suspicion of abnormal cerebellar development is usually raised following the visualization of a small cerebellum or a large communication between the fourth ventricle and the cisterna magna, using the transcerebellar axial plane [Malinger et al., 2006]. Severe anomalies, such as Arnold–Chiari malformation type 2, Dandy–Walker malformation [Oh et al., 2005; Denis et al., 2001], and cerebellar hypoplasia, are usually detected during routine second-trimester ultrasound examinations. However, prenatal diagnosis of isolated cerebellar and/or vermian anomalies may be difficult because of the complexity of normal development of these structures.
A systematic approach to the posterior fossa is recommended in order to enable differentiation between normal and abnormal development [Guibaud and des Portes, 2006; Adamsbaum et al., 2005]. Understanding the anatomy is vital to avoid misdiagnosis and to characterize the abnormalities accurately. Confirmation of the diagnosis necessitates the visualization of the cerebellum in the coronal planes, and a true midline sagittal picture of the vermis, brainstem, and fourth ventricle. Particular care should be taken in order to differentiate between the vermis and the cerebellar hemisphere in cases of suspected vermian agenesis or hypoplasia. Visualization of the normal triangular shape of the fourth ventricle, and of the primary vermian fissure, facilitates exclusion of vermian pathologies [Denis et al., 2001]. Vermian agenesis may also be part of the molar tooth-associated syndromes.
The in utero diagnosis of the pontocerebellar hypoplasias [Klein et al., 2003] necessitates measurements of the pons circumference in addition to the cerebellar dimensions.
In cases of diagnostic uncertainties, a follow-up examination is pertinent, since a false positive diagnosis of vermian agenesis may be made before 24 weeks of pregnancy, due to delayed closure of the fourth ventricle owing to a persistent Blake pouch cyst [Robinson and Goldstein, 2007], and cerebellar hypoplasia may be missed early in pregnancy because the arrest of growth occurs later [Malinger et al., 2009].
A dysplastic cerebellum, which refers to disorganized development, such as an abnormal folial pattern or the presence of heterotopic nodules of gray matter, is rarely diagnosed in utero, even by MRI, since it does not produce signal abnormalities [Tilea et al., 2007]. However, in most cases of posterior fossa anomalies, there is good agreement between fetal MRI and fetopathological results.
Prenatal Diagnosis of Chiari Type II
The posterior fossa is relatively small in Chiari II, and spinal neural tube defects are present with variable hindbrain herniation. The Chiari II malformation may be associated with callosal anomalies, fenestration of the falx, and cortical malformations. The cisterna magna is often obliterated with a low-lying torcula, vermian herniation through the foramen magnum, and a small slitlike fourth ventricle. The herniated cerebellum often degenerates and may be virtually absent in severe cases [Boltshauser et al., 2002]. When visible, the cerebellum is banana-shaped due to the herniation and small posterior fossa. The fetus may demonstrate a lemon-shaped skull [Van den Hof et al., 1990]. The lemon sign is created by the flattened frontal bones and, in most cases, is seen with an open myelomeningocele before 24 weeks’ gestation. Ultrasound is sensitive and specific for detection of the Chiari II malformation [Oh et al., 2007].
Prenatal Diagnosis of Dandy–Walker Malformation
Prenatal diagnosis of Dandy–Walker malformation is usually possible during the second trimester; midsagittal planes enable visualization of the abnormal vermis, the communication between the fourth ventricle and the enlarged cisterna magna, and the elevated torcula (Figure 29-16C). It should not be confused with partial vermian agenesis, in which there is a large communication between the fourth ventricle and the cisterna magna without elevation of the torcula (Figure 29-16B and Figure 29-17C). Dandy–Walker malformation may be associated with other central nervous system anomalies, such as callosal dysgenesis, occipital encephalocele, polymicrogyria, or heterotopias, so these should be sought. Hydrocephalus may only develop late in pregnancy or postnatally.
The prognosis following a prenatal diagnosis of Dandy–Walker malformation is variable; however, the presence of a normally lobulated vermis and the absence of associated brain anomalies are associated with a more favorable outcome [Bolduc and Limperopoulos, 2009].
Prenatal Diagnosis of Mega Cisterna Magna, Posterior Fossa Arachnoid Cyst, Blake’s Pouch Cyst
Isolated enlargement of the posterior fossa is frequently diagnosed by fetal ultrasound or MRI. It may be seen in three situations: mega cisterna magna, posterior fossa arachnoid cyst, and Blake’s pouch cyst. The prognosis in all three entities is usually good [Dror et al., 2009].
In mega cisterna magna, the posterior fossa depth is greater than 10 mm, but the vermis and torcula location are normal. The term has been loosely applied to a large-appearing retrocerebellar cerebrospinal fluid space with a normal vermis and cerebellar hemispheres. It is usually an incidental finding, but it can be associated with other anomalies. Mega cisterna magna can be difficult to distinguish from an arachnoid cyst, as both are anechoic fluid spaces and may result in mild scalloping of the skull (see Figure 29-17A). Some authors [Nelson et al., 2004] claim that mega cisterna magna is not a true diagnosis since, when such spaces are carefully examined at autopsy, delicate membranes of a retrocerebellar cyst become apparent and histologically they are usually of the arachnoid or Blake’s pouch variety.
Doppler ultrasound should be used to exclude any internal vascularity, which would be seen in a vascular malformation or cystic tumor [Oh et al., 2007].
Blake’s pouches have the same radiographic appearance as do arachnoidal cysts, with the exception that, in some cases, the choroid plexus may be identified, as it extends through the median aperture along the superior cyst wall, carrying the anterior lip of the median aperture far up the vallecula. Blake’s pouches usually communicate with the fourth ventricle and may or may not produce mass effect on the cerebellum. The falx cerebelli is usually present and the torcula is usually in a normal position, but may be elevated. There may be pressure erosion of the occipital bone. Occasionally, there is the appearance of compression or absence of the inferior vermis (see Figure 29-17D). Other central nervous system malformations are rarely associated with Blake’s pouches [Nelson et al., 2004]. It may be hard to differentiate between inferior vermis hypoplasia and a Blake’s pouch cyst [Limperopoulos et al., 2006].
Prenatal Diagnosis of Vermis Hypoplasia/Agenesis
The term Dandy–Walker variant has been used to describe a heterogeneous group of disorders with different degrees of cerebellar vermis agenesis, slight or absent upward rotation of the vermis, and variably sized posterior fossa fluid collections, but without enlargement of the posterior fossa. However, in recent years, it has been strongly advocated that the term Dandy–Walker variant be abandoned altogether, given its multiple and variable definitions [Parisi and Dobyns, 2003]. The terms hypoplasia and agenesis are used interchangeably in the literature on fetal vermian abnormalities, but actually the definitions of these entities are completely different [Guibaud and des Portes, 2006]. Vermian agenesis means either complete or partial absence of the vermis. In partial vermian agenesis, part of the vermis is absent and the remaining part is anatomically of normal volume. Due to the craniocaudal development of the vermis, partial agenesis involves its inferior part (see Figure 29-17C). Vermian hypoplasia means a small but complete vermis with congenital volume diminution.
Vermis agenesis is associated with central nervous system and non-central nervous system anomalies in up to 71 percent of children, with the most common being ventriculomegaly and agenesis of corpus callosum. Extra-central nervous system anomalies have also been reported in up to 65 percent, with cardiac, renal, extremity, and facial anomalies occurring most frequently [Bolduc and Limperopoulos, 2009].
Vermian development is assessed in the midline sagittal view from the caudal extent of the inferior vermis over the fourth ventricle. The diagnosis of inferior vermis agenesis (still referred to in the literature as hypoplasia) is made when there is partial absence of the inferior portion of the cerebellar vermis with normal- or near-normal-shaped cerebellar hemispheres, a normal-sized posterior fossa without obvious cystic lesions, and normal supratentorial structures. The normal proportion of anterior vermis to posterior vermis (1:2) is lost. A correct diagnosis of inferior vermian agenesis is difficult and, even with MRI, there is a false-positive rate of 32 percent [Limperopoulos et al., 2006].
In cases of sonographically suspected vermian hypoplasia or agenesis, fetal MRI is helpful in determining the shape and size of the vermis, and the relationship between a posterior fossa cyst and the fourth ventricle [Glenn and Barkovich, 2006]. The identification of an intact vermis can help to differentiate it from a mega cisterna magna. However, even when apparently isolated vermian hypoplasia/agenesis is detected, it is difficult to be certain of the diagnosis, and therefore it warrants subsequent fetal MRI studies or postnatal MRI confirmation [Triulzi et al., 2006].
Inferior vermian agenesis should be differentiated from failure of “closure” of the vermis with normal morphology and biometry, and without any associated fetal abnormalities, this probably represents isolated elevation or rotation of the vermis due to a persistent Blake’s pouch [Robinson et al., 2007] and does not necessarily indicate an adverse outcome. Failure of “closure” seems to result from two potential processes: arrest of vermian development so that it does not cover the fourth ventricle at its inferior extent, or failure of adequate fenestration of the fourth ventricular outflow foramina, leading to a secondary elevation of an otherwise normal vermis. The prognosis in these situations is completely different.
Prenatal Diagnosis of Cerebellar Hypoplasia
Cerebellar hypoplasia implies abnormal development rather than atrophy. When a small-appearing cerebellum is seen, either the cerebellum is truly small or the posterior fossa is relatively large. Diagnosis is made by measurement of the transverse cerebellar diameter in millimeters, which should be about equal to the gestational age in weeks [Oh et al., 2007]. Many fetuses with cerebellar hypoplasia or agenesis do not present with an enlarged cisterna magna [Tilea et al., 2007].
Isolated cerebellar hypoplasia with a normal bulge of the pons is more challenging and difficulty in distinguishing malformation from necrosis can occur, especially in unilateral hypoplasia. An intact cerebellar cortex, whether irregular or not, is most likely seen in cerebellar hypoplasia, as opposed to cerebellar necrosis, in which the cortical ribbon is usually absent [Fogliarini et al., 2005b].
Prenatal Diagnosis of Rhombencephalosynapsis
Ultrasound diagnosis of rhombencephalosynapsis is generally suspected after 22 weeks of gestation, and usually the abnormality is suggested by ventriculomegaly [Pasquier et al., 2009]. The diagnosis is raised, following demonstration of a small transcerebellar diameter. Ultrasound and MRI both demonstrate a hypoplastic, single-lobed cerebellum with fused cerebellar hemispheres, no vermis, and transverse folia (Figure 29-18). Associated cerebral abnormalities are usually found and include additional midline defects, such as aqueductal stenosis leading to hydrocephalus, holoprosencephaly, corpus callosum dysgenesis, and septo-optic dysplasia. Extracranial anomalies include segmentation and fusion anomalies of the spine, musculoskeletal anomalies, and cardiovascular, respiratory, and urinary tract defects. Rhombencephalosynapsis may be frequently associated with VACTERL-H (vertebral anomalies, anal atresia, cardiac defects, tracheoesophageal fistula, esophageal atresia, renal anomalies, hydrocephalus) syndrome [Pasquier et al., 2009].
It is important to emphasize that coronal and axial planes are superior for diagnosis of rhombencephalosynapsis, since a midline sagittal cut through the cerebellum can be mistaken for a vermis. A narrow diamond-shaped fourth ventricle and a fused horseshoe-shaped dentate nucleus are the features seen on axial MRI planes. Sagittal views, when carefully interpreted, can demonstrate the lack of visualization of the primary vermian fissure and lack of a normal fastigial point of the fourth ventricle [McAuliffe et al., 2008].
Prenatal Diagnosis of Molar Tooth-Related Syndromes
The diagnosis of the molar tooth (deep interpeduncular fossa, thick and elongated superior cerebellar peduncles, vermis agenesis) is difficult to visualize in utero. However, the prenatal diagnosis of Joubert’s syndrome or related disorders has been described in the literature, usually following a positive family history and the finding of abnormal posterior fossa anatomy on fetal ultrasonography or the presence of associated suggestive features, such as kidney anomalies, polydactyly, or an abnormal fetal breathing pattern [Doherty et al., 2005; Fluss et al., 2006]. Ultrasound and MRI demonstrate an enlarged fourth ventricle with an abnormal fastigium, and the vermis is not clearly observed in the coronal and sagittal planes (see Figure 29-17B). The molar tooth features may be visualized in the axial plane, including the interpeduncular fossa, cerebellar peduncles, and brainstem.
Adamsbaum C., Moutard M.L., Andre C., et al. MRI of the fetal posterior fossa. Pediatr Radiol. 2005;35:124-140.
Bargallo N., Puerto B., De Juan C., et al. Hereditary subependymal heterotopia associated with mega cisterna magna: antenatal diagnosis with magnetic resonance imaging. Ultrasound Obstet Gynecol. 2002;20:86-89.
Blaas H.G., Eik-Nes S.H. Sonoembryology and early prenatal diagnosis of neural anomalies. Prenat Diagn. 2009;29:312-325.
Bolduc M.E., Limperopoulos C. Neurodevelopmental outcomes in children with cerebellar malformations: a systematic review. Dev Med Child Neurol. 2009;51:256-267.
Boltshauser E., Schneider J., Kollias S., et al. Vanishing cerebellum in myelomeningocoele. Eur J Paediatr Neurol. 2002;6:109-113.
Brugger P.C., Stuhr F., Lindner C., et al. Methods of fetal MR: beyond T2- weighted imaging. Eur J Radiol. 2006;57:172-181.
Chen C.-Y., Zimmerman R.A., Faro S., et al. MR of the cerebral operculum: topographic identification and measurement of interopercular distances in healthy infants and children. Am J Neuroradiol. 1995;16:1677-1687.
Chi J.G., Dooling E.C., Gilles F.H. Gyral development of the human brain. Ann Neurol. 1977;1:86-93.
Cohen-Sacher B., Lerman-Sagie T., Lev D., et al. Sonographic developmental milestones of the fetal cerebral cortex: a longitudinal study. Ultrasound Obstet Gynecol. 2006;27:494-502.
Crowe C., Jassani M., Dickerman L. The prenatal diagnosis of Warburg syndrome. Am J Hum Genet. 1985;37:A214.
Denis D., Maugey-Laulom B., Carles D., et al. Prenatal diagnosis of schizencephaly by fetal magnetic resonance imaging. Fetal Diagn Ther. 2001;16:354-359.
Doherty D., Glass I.A., Siebert J.R., et al. Prenatal diagnosis in pregnancies at risk for Joubert syndrome by ultrasound and MRI. Prenat Diagn. 2005;25:442-447.
Dorovini-Zis K., Dolman C.L. Gestational development of brain. Arch Pathol Lab Med. 1977;101:192-195.
Dror R., Malinger G., Ben-Sira L., et al. Developmental Outcome of Children With Enlargement of the Cisterna Magna Identified in Utero. J Child Neurol. 2009. Feb 23. [Epub ahead of print]
Ertl-Wagner B., Lienemann A., Strauss A., et al. Fetal magnetic resonance imaging: indications, technique, anatomical considerations and a review of fetal abnormalities. Eur Radiol. 2002;12:1931-1940.
Falip C., Blanc N., Maes E., et al. Postnatal clinical and imaging follow-up of infants with prenatal isolated mild ventriculomegaly: a series of 101 cases. Pediatr Radiol. 2007;37:981-989.
Farrell S.A., Toi A., Leadman M.L., et al. Prenatal diagnosis of retinal detachment in Walker-Warburg syndrome. Am J Hum Genet. 1987;28:619-624.
Fluss J., Blaser S., Chitayat D., et al. Molar tooth sign in fetal brain magnetic resonance imaging leading to the prenatal diagnosis of Joubert syndrome and related disorders. J Child Neurol. 2006;21:320-324.
Fogliarini C., Chaumoitre K., Chapon F., et al. Assessment of cortical maturation with prenatal MRI. Part I: normal cortical maturation. Eur Radiol. 2005;15:1671-1685.
Fogliarini C., Chaumoitre K., Chapon F., et al. Assessment of cortical maturation with prenatal MRI. Part II: abnormalities of cortical maturation. Eur Radiol. 2005;15:1781-1789.
Fong K.W., Ghai S., Toi A., et al. Prenatal ultrasound findings of lissencephaly associated with Miller–Dieker syndrome and comparison with pre- and postnatal magnetic resonance imaging. Ultrasound Obstet Gynecol. 2004;24:716-723.
Garel C., Chantrel E., Brisse H., et al. Fetal cerebral cortex: normal gestational landmarks identified using prenatal MR imaging. Am J Neuroradiol. 2001;22:184-189.
Gasser B., Lindner V., Dreyfus M., et al. Prenatal diagnosis of Walker-Warburg syndrome in three sibs. Am J Med Genet. 1998;76:107-110.
Glenn O.A. Normal development of the fetal brain by MRI. Semin Perinatol. 2009;33:208-219.
Glenn O.A., Barkovich J. Magnetic resonance imaging of the fetal brain and spine: an increasingly important tool in prenatal diagnosis: part 2. Am J Neuroradiol. 2006;27:1807-1814.
Greco P., Resta M., Vimercati A., et al. Antenatal diagnosis of isolated lissencephaly by ultrasound and magnetic resonance imaging. Ultrasound Obstet Gynecol. 1991;12:276-279.
Guibaud L. Contribution of fetal cerebral MRI for diagnosis of structural anomalies. Prenat Diagn. 2009;29:420-433.
Guibaud L., de Portes V. Plea for an anatomical approach to abnormalities of the posterior fossa in prenatal diagnosis. Ultrasound Obstet Gynecol. 2006;27:477-481.
Guibaud L., Selleret L., Larroche J.C., et al. Abnormal Sylvian fissure on prenatal cerebral imaging: significance and correlation with neuropathological and postnatal data. Ultrasound Obstet Gynecol. 2008;32:50-60.
Huisman T.A., Wisser J., Martin E., et al. Fetal magnetic resonance imaging of the central nervous system: a pictorial essay. Eur Radiol. 2002;12:1952-1961.
ISUOG guidelines. Sonographic examination of the fetal central nervous system: guidelines for performing the basic examination and the fetal neurosonogram. Ultrasound Obstet Gynecol. 2007;29:109-116.
Kennelly M.M., Cooley S.M., McParland P.J. Natural history of apparently isolated severe fetal ventriculomegaly: perinatal survival and neurodevelopmental outcome. Prenat Diagn. 2009;29:1135-1140.
Klein O., Pierre-Kahn A., Boddaert N., et al. Dandy-Walker malformation: prenatal diagnosis and prognosis. Childs Nerv Syst. 2003;19:484-489.
Kojima K., Suzuki Y., Seki K., et al. Prenatal diagnosis of lissencephaly (type II) by ultrasound and fast magnetic resonance imaging. Fetal Diagn Ther. 2002;17:34-36.
Kostovic I., Vasung L. Insights from in vitro fetal magnetic resonance imaging of cerebral development. Semin Perinatol. 2009;33:220-233.
Lerman-Sagie T., Ben-Sira L., Achiron R., et al. Thick fetal corpus callosum: an ominous sign? Ultrasound Obstet Gynecol. 2009;34:55-61.
Lerman-Sagie T., Malinger G. Focus on the fetal Sylvian fissure. Ultrasound Obstet Gynecol. 2008;32(1):3-4.
Levine D., Barnes P.D. Cortical maturation in normal and abnormal fetuses as assessed with prenatal MR imaging. Radiology. 1999;210:751-758.
Limperopoulos C., Clouchoux C. Advancing fetal brain MRI: targets for the future. Semin Perinatol. 2009;33:289-298.
Limperopoulos C., Robertson R.L., Estroff J.A., et al. Diagnosis of inferior vermian hypoplasia by fetal magnetic resonance imaging: potential pitfalls and neurodevelopmental outcome. Am J Obstet Gynecol. 2006;194:1070-1076.
Limperopoulos C., Robertson R.L., Khwaja O.S., et al. How accurately does current fetal imaging identify posterior fossa anomalies? Am J Roentgenol. 2008;190:1637-1643.
Malinger G., Ginath S., Lerman-Sagie T., et al. The fetal cerebellar vermis: normal development as shown by transvaginal ultrasound. Prenat Diagn. 2001;21:687-692.
Malinger G., Katz A., Zakut H. Transvaginal fetal neurosonography. Supratentorial structures. Isr J Obstet Gynecol. 1993;4:1-5.
Malinger G., Kidron D., Schreiber L., et al. Prenatal diagnosis of malformations of cortical development by dedicated neurosonography. Ultrasound Obstet Gynecol. 2007;29:178-191.
Malinger G., Lev D., Lerman-Sagie T. Abnormal sulcation as an early sign for migration disorders. Ultrasound Obstet Gynecol. 2004;24:704-705.
Malinger G., Lev D., Lerman-Sagie T. Normal and abnormal fetal brain development during the third trimester as demonstrated by neurosonography. Eur J Radiol. 2006;57:226-232.
Malinger G., Lev D., Lerman-Sagie T. The fetal cerebellum. Pitfalls in diagnosis and management. Prenat Diagn. 2009;29:372-380.
McAuliffe F., Chitayat D., Halliday W., et al. Rhombencephalosynapsis: prenatal imaging and autopsy findings. Ultrasound Obstet Gynecol. 2008;31:542-548.
Melchiorre K., Bhide A., Gika A.D., et al. Counseling in isolated mild fetal ventriculomegaly. Ultrasound Obstet Gynecol. 2009;34:212-224.
Mitchell L.A., Simon E.M., Filly R.A., et al. Antenatal diagnosis of subependymal heterotopia. Am J Neuroradiol. 2000;21:296-300.
Monteagudo A., Alayón A., Mayberry P. Walker-Warburg syndrome: case report and review of the literature. J Ultrasound Med. 2001;20:419-426.
Nadich T.P., Grant J.L., Altman N., et al. The developing cerebral surface. Neuroimaging Clin N Am. 1994;4:201-240.
Nelson M.D.Jr, Maher K., Gilles F.H. A different approach to cysts of the posterior fossa. Pediatr Radiol. 2004;34:720-732.
Oh K.Y., Kennedy A.M., Frias A.E., et al. Fetal schizencephaly: pre- and postnatal imaging with a review of the clinical manifestations. Radiographics. 2005;25:647-657.
Oh K.Y., Rassner U.A., Frias A.E., et al. The fetal posterior fossa: clinical correlation of findings on prenatal ultrasound and fetal magnetic resonance imaging. Ultrasound Q. 2007;23:203-210.
Onyeije C.I., Sherer D.M., Jarosz C.J., et al. Prenatal sonographic findings associated with sporadic subcortical nodular heterotopia. Obstet Gynecol. 1998;91:799-801.
Ouahba J., Luton D., Vuillard E., et al. Prenatal isolated mild ventriculomegaly: outcome in 167 cases. BJOG. 2006;113:1072-1079.
Parisi M.A., Dobyns W.B. Human malformations of the midbrain and hindbrain: Review and proposed classification scheme. Mol Genet Metab. 2003;80:36-53.
Pasquier L., Marcorelles P., Loget P., et al. Rhombencephalosynapsis and related anomalies: a neuropathological study of 40 fetal cases. Acta Neuropathol. 2009;117(2):185-200.
Pilu G., Falco P., Gabrielli S., et al. The clinical significance of fetal isolated cerebral borderline ventriculomegaly: report of 31 cases and review of the literature. Ultrasound Obstet Gynecol. 1999;14:320-326.
Prasad A.N., Malinger G., Lerman-Sagie T. Primary disorders of metabolism and disturbed fetal brain development. Clin Perinatol. 2009;36:621-638.
Prayer D., Kasprian G., Krampl E., et al. MRI of normal fetal brain development. Eur J Radiol. 2006;57:199-216.
Quarello E., Stirnemann J., Ville Y., et al. Assessment of fetal Sylvian fissure operculization between 22 and 32 weeks: a subjective approach. Ultrasound Obstet Gynecol. 2008;32:44-49.
Robinson A.J., Blaser S., Toi A., et al. The fetal cerebellar vermis: assessment for abnormal development by ultrasonography and magnetic resonance imaging. Ultrasound Q. 2007;23:211-223.
Robinson A.J., Goldstein R. The cisterna magna septa: vestigial remnants of Blake’s pouch and a potential new marker for normal development of the rhombencephalon. J Ultrasound Med. 2007;26:83-95.
Rodgers B.L., Vanner L.V., Pai G.S., et al. Walker-Warburg syndrome: report of three affected sibs. Am J Med Genet. 1994;49:198-201.
Sadan S., Malinger G., Schweiger A., et al. Neuropsychological outcome of children with asymmetric ventricles or unilateral mild ventriculomegaly identified in utero. BJOG. 2007;114:596-602.
Salomon L.J., Ouahba J., Delezoide A.L., et al. Third-trimester fetal MRI in isolated 10–12 mm ventriculomegaly: is it worth it? BJOG. 2006;113:942-947.
Saltzman D.H., Krauss C.M., Goldman J.M., et al. Prenatal diagnosis of lissencephaly. Prenat Diagn. 1991;11:139-143.
Sonigo P.C., Rypens F.F., Carteret M., et al. MR imaging of fetal cerebral anomalies. Pediatr Radiol. 1998;28:212-222.
Strigini F., Valleriani A., Cecchi M., et al. Prenatal ultrasound and magnetic resonance imaging features in a fetus with Walker-Warburg syndrome. Ultrasound Obstet Gynecol. 2009;33:363-365.
Tang P.H., Bartha A.I., Norton M.E., et al. Agenesis of the corpus callosum: an MR imaging analysis of associated abnormalities in the fetus. Am J Neuroradiol. 2009;30:257-263.
Tilea B., Delezoide A.L., Khung-Savatovski S., et al. Comparison between magnetic resonance imaging and fetopathology in the evaluation of fetal posterior fossa non-cystic abnormalities. Ultrasound Obstet Gynecol. 2007;29:651-659.
Toi A., Chitayat D., Blaser S. Abnormalities of the foetal cerebral cortex. Prenat Diagn. 2009;29:355-371.
Toi A., Lister W.S., Fong K.W. How early are fetal cerebral sulci visible at prenatal ultrasound and what is the normal pattern of early fetal sulcal development? Ultrasound Obstet Gynecol. 2004;24:706-715.
Triulzi F., Parazzini C., Righini A. Magnetic resonance imaging of fetal cerebellar development. Cerebellum. 2006;5:199-205.
Triulzi F., Parazzini C., Righini A. MRI of fetal and neonatal cerebellar development. Semin Fetal Neonatal Med. 2005;10:411-420.
Valsky D.V., Ben-Sira L., Porat S., et al. The role of magnetic resonance imaging in the evaluation of isolated mild ventriculomegaly. J Ultrasound Med. 2004;23:519-523.
Van den Hof M.C., Nicolaides K.H., Campbell J., et al. Evaluation of the lemon and banana signs in one hundred thirty fetuses with open spina bifida. Am J Obstet Gynecol. 1990;162:322-327.