Pleural Diseases
After reading this chapter you will be able to:
Describe important anatomic features and physiologic function of the visceral and parietal pleural membranes.
Describe how pleural effusions occur and the difference between transudative and exudative effusions.
Identify common causes of transudative and exudative pleural effusions.
Write definitions of chylothorax, hemothorax, and pneumothorax.
Describe the impact of moderate to large pleural effusions on lung function.
State the role of a chest radiograph in recognizing pleural effusions.
State the purpose of thoracentesis and the potential complications.
Identify the definitions of spontaneous, secondary, and tension pneumothorax.
Pleural Space
Overview and Definitions
The blood vessels and airways that enter the lung connect to the mediastinum at the lung hilum. At this juncture, the visceral pleura meets the mediastinal parietal pleura to form a single, continuous pleural membrane (Figure 25-1).
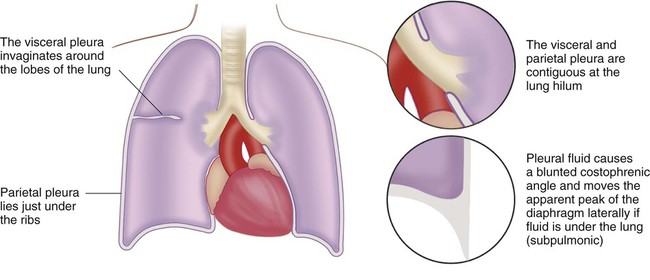
The average person has approximately 8 ml of pleural fluid per hemithorax.1 The pleural fluid is estimated to have a total protein concentration similar to that of interstitial fluid elsewhere in the body: between 1.3 g/dl and 1.4.2
Pleural Effusions
Any abnormal amount of pleural fluid in the pleural space is called pleural effusion. The many causes of pleural effusion are categorized according to etiologic factor and the content of the fluid.3
Transudative Effusions
Any pleural effusion that forms when the integrity of the pleural space is undamaged is called a transudative pleural effusion. A pleural fluid total protein concentration less than 50% of the serum total protein level and lactate dehydrogenase values in the pleural fluid less than 60% of the serum value indicate the presence of a transudative pleural effusion. In the absence of serum values, an absolute pleural fluid lactate dehydrogenase level less than two-thirds normal for serum suggests the presence of a transudate. These numbers were derived from large patient series in which pleural fluid and serum protein concentrations were measured while the cause of the effusion was being determined and corrected.4
The classification system listed in Box 25-1 is not perfect, and refinements continue to be proposed. For practical purposes, these numbers help narrow the possible causes of pleural fluid formation. Transudative pleural effusions form when hydrostatic and oncotic pressures are abnormal (Figure 25-2).5 The list of diseases that cause transudative pleural effusions is short. These diseases remain relatively easy to diagnose.
Congestive Heart Failure
Elevation of pressure in the left atrium and pulmonary veins is the hallmark of congestive heart failure (CHF). Elevation of pulmonary venous pressure increases the amount of interstitial fluid in the lung. In severe cases, flooding of the alveoli causes pulmonary edema, but in less severe cases, interstitial lung water increases and decompresses into the pleural space. Because systemic venous pressure also is elevated, there is limited capability to remove pleural fluid through the intercostal veins. Pleural fluid must be predominantly removed by the lymphatic vessels. Pleural effusions result when the capacity of pleural lymphatic drainage is overcome.6
CHF is the most common cause of clinical pleural effusions. The effusions can be massive, filling the entire hemithorax and compressing the lung. More commonly, they are small and bilateral. The effusions are rarely drained because outcome is heavily influenced by successful management of the underlying CHF, which also clears the effusions.7
Liver Disease
All ascitic fluid can end up in the chest because of the pressure gradient, and true ascites can be absent. This condition often is quite difficult to manage except with methods that limit ascites formation, such as sodium restriction and diuretics. Excessive pleural fluid is present in approximately 6% of patients with ascites, and 70% of these fluid collections are on the right side.8
Exudative Effusions
An exudative pleural effusion is caused by inflammation in the lung or pleura. This type of pleural effusion has more protein and inflammatory cells present than a transudative effusion. Because therapy for pleural effusion depends on the cause, thoracentesis often is performed to determine the specific biochemical and cellular characteristics of the pleural effusion. Box 25-1 lists the common causes of exudative pleural effusion. These account for approximately 70% of all pleural effusions.
Parapneumonic Effusion
Pleural effusions form in pneumonia because inflammation in the lung increases interstitial lung water and pleural fluid production. Most effusions are small and resolve with resolution of bacterial pneumonia.9 Complicated parapneumonic pleural effusion develops when the pleural fluid has a high enough protein content to clot. The clotting causes fibrin strands to span the visceral and parietal pleurae. The net result is collection of pleural fluid into different loculi within the pleural cavity. These often cannot be drained by a single chest tube.
Progression to empyema is marked by the presence of bacteria within the pleural space, seen as pus or bacteria on Gram stain. Empyema necessitates drainage. Whether complicated parapneumonic effusions necessitate drainage is controversial, although most physicians perform drainage because some of these effusions can progress to empyema.10
Tuberculous Pleurisy
Although these patients require respiratory isolation, only 25% of them have sputum that subsequently grows Mycobacterium tuberculosis. The PPD skin test result is negative in 30% of patients when they come to medical attention but turns positive in 6 to 8 weeks in almost everyone.11
Postoperative Causes
Various operations involving the chest or upper abdomen produce pleural fluid.12 Effusions following cardiac surgery usually are predominant on the left side and tend to be bloody. These effusions are particularly prevalent after a cutdown of the internal mammary artery for coronary artery bypass.
Chylothorax
The thoracic duct is a lymphatic channel that runs from the abdomen through the mediastinum to enter the left subclavian vein. Disruption of the thoracic duct anywhere along its course can cause leakage of chyle into the mediastinum, which may rupture into the pleural space and cause a chylothorax. The most common causes of rupture are malignancy (50%), surgery (20%), and trauma (5%).13 The thoracic duct courses through the right side of the mediastinum in the lower thoracic cavity before crossing to the left side of the mediastinum at T4 to T6. Rupture below this level causes right-sided pleural effusion, whereas rupture above this level causes left-sided pleural effusion.
In a patient who has eaten recently, the effusions are milky white as a result of the presence of chylomicrons (microscopic fat particles) absorbed by abdominal lymphatic vessels. In a fasting patient, these effusions usually are yellow. The effusions may be bloody. A pleural fluid triglyceride concentration greater than 110 mg/dl confirms the diagnosis.14 Computed tomography (CT) should be performed to evaluate the cause of the chylothorax.
Hemothorax
Although hemothorax is seen most commonly after blunt or penetrating chest trauma, numerous medical conditions can give rise to blood in the pleural space. These conditions should be considered in the absence of trauma. Any vein or artery in the thorax can bleed into the pleural space. A chest tube usually is inserted to monitor the rate of bleeding and determine whether the source is arterial or venous.15