Placenta and Extraembryonic Membranes
The tissues that compose the fetal-maternal interface (placenta and chorion) are derivatives of the trophoblast, which separates from the inner cell mass and surrounds the cellular precursors of the embryo proper even as the cleaving zygote travels down the uterine tube on its way to implanting into the uterine wall (see Fig. 3.18). Other extraembryonic tissues are derived from the inner cell mass. These include the following: the amnion (an ectodermal derivative), which forms a protective fluid-filled capsule around the embryo; the yolk sac (an endodermal derivative), which in mammalian embryos no longer serves a primary nutritive function; the allantois (an endodermal derivative), which is associated with the removal of embryonic wastes; and much of the extraembryonic mesoderm, which forms the bulk of the umbilical cord, the connective tissue backing of the extraembryonic membranes, and the blood vessels that supply them.
Extraembryonic Tissues
Amnion
The origin of the amniotic cavity within the ectoderm of the inner cell mass in the implanting embryo is described in Chapter 5 (see Figs. 3.18 and 5.2). As the early embryo undergoes cephalocaudal and lateral folding, the amniotic membrane surrounds the body of the embryo like a fluid-filled balloon (Fig. 7.1), thus allowing the embryo to be suspended in a liquid environment for the duration of pregnancy. The amniotic fluid serves as a buffer against mechanical injury to the fetus; in addition, it accommodates growth, allows normal fetal movements, and protects the fetus from adhesions.
The thin amniotic membrane consists of a single layer of extraembryonic ectodermal cells lined by a nonvascularized layer of extraembryonic mesoderm. Keeping pace with fetal growth, the amniotic cavity steadily expands until its fluid content reaches a maximum of nearly 1 L by weeks 33 to 34 of pregnancy (Fig. 7.2).
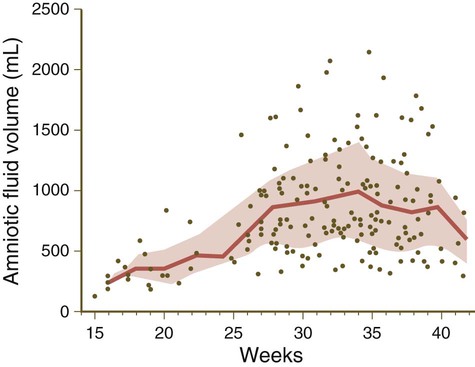
The lined and shaded area represents the mean ± standard deviation. Dots represent outlying values. (Data from Queenan JT and others: Am J Obstet Gynecol 114:34-38, 1972.)
In the third trimester of pregnancy, the amniotic fluid turns over completely every 3 hours, and at term, the fluid-exchange rate may approach 500 mL/hour. Although much of the amniotic fluid is exchanged across the amniotic membrane, fetal swallowing is an important mechanism in late pregnancy, with about 20 mL/hour of fluid swallowed by the fetus. Swallowed amniotic fluid ultimately enters the fetal bloodstream after absorption through the gut wall. The ingested water can leave the fetal circulation through the placenta. During the fetal period, excreted urine from the fetus contributes to amniotic fluid. Clinical Correlation 7.1 discusses conditions related to the amount of amniotic fluid or substance concentrations in the fluid.
Yolk Sac
The yolk sac, which is lined by extraembryonic endoderm, is formed ventral to the bilayered embryo when the amnion appears dorsal to the embryonic disk (see Fig. 5.2). In contrast to birds and reptiles, the yolk sac of mammals is small and devoid of yolk. Although vestigial in terms of its original function as a major source of nutrition, the yolk sac remains vital to the embryo because of other functions that have become associated with it. Some evidence indicates that before the placental circulation is established, nutrients, such as folic acid and vitamins A, B12, and E, are concentrated in the yolk sac and are absorbed by endocytosis. Because this form of histiotrophic nutrition occurs during the time of neurulation, it may play a role in the prevention of neural tube defects (see p. 138).
When it first appears, the yolk sac is in the form of a hemisphere bounded at the equatorial region by the dorsal wall of the primitive gut (see Fig. 7.1). As the embryo grows and undergoes lateral folding and curvature along the craniocaudal axis, the connection between the yolk sac and the forming gut becomes attenuated in the shape of a progressively narrowing stalk attached to a more spherical yolk sac proper at its distal end. In succeeding weeks, the yolk stalk becomes very long and attenuated as it is incorporated into the body of the umbilical cord. The yolk sac itself moves nearer the chorionic plate of the placenta (Fig. 7.3).
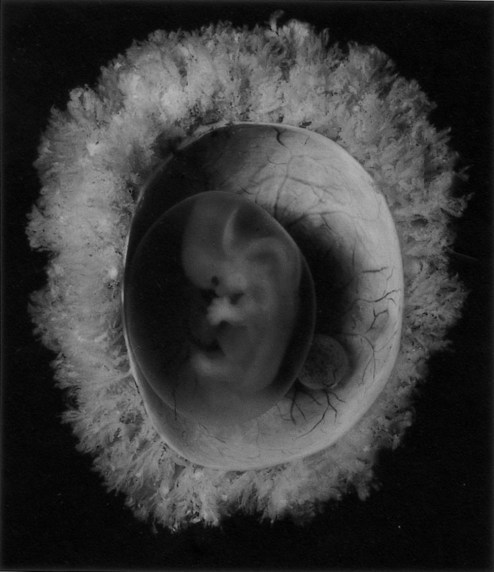
The embryo was exposed by cutting open the chorion. The small sphere to the right of the embryo is the yolk sac. (Carnegie embryo No. 8537A, Courtesy of Chester Reather, Baltimore.)
The endoderm of the yolk sac is lined on the outside by well-vascularized extraembryonic mesoderm. Cells found in each of these layers contribute vital components to the body of the embryo. During the third week, primordial germ cells, which arise in the extraembryonic mesoderm near the base of the allantois, become recognizable in the lining of the yolk sac (see Fig. 1.1). Soon these cells migrate into the wall of the gut and the dorsal mesentery as they make their way to the gonads, where they differentiate into oogonia or spermatogonia.
In the meantime, groups of extraembryonic mesodermal cells in the wall of the yolk sac become organized into blood islands (see Fig. 6.19), and many of the cells differentiate into primitive blood cells. Extraembryonic hematopoiesis continues in the yolk sac until about the sixth week, when blood-forming activity transfers to intraembryonic sites, especially the liver.
As the tubular gut forms, the attachment site of the yolk stalk becomes progressively less prominent, until by 6 weeks it has effectively lost contact with the gut. In a small percentage of adults, traces of the yolk duct persist as a fibrous cord or an outpouching of the small intestine known as Meckel’s diverticulum (see Fig. 15.15A). The yolk sac itself may persist throughout much of pregnancy, but it is not known to have a specific function in the fetal period. The proximal portions of the blood vessels of the yolk sac (the vitelline circulatory arc) persist as vessels that supply the midgut region.
Allantois
The allantois arises as an endodermally lined ventral outpocketing of the hindgut (see Fig. 7.1). In the human embryo, it is just a vestige of the large, saclike structure that is used by the embryos of many mammals, birds, and reptiles as a major respiratory organ and repository for urinary wastes. Similar to the yolk sac, the allantois in a human retains only a secondary function, in this case respiration. In humans, this function is served by the blood vessels that differentiate from the mesodermal wall of the allantois. These vessels form the umbilical circulatory arc, consisting of the arteries and veins that supply the placenta (see Fig. 6.26). (The postnatal fate of these vessels is discussed in Chapter 18.)
The allantois proper, which consists of little more than a cord of endodermal cells, is embedded in the umbilical cord. Later in development, the proximal part of the allantois (called the urachus) is continuous with the forming urinary bladder (see Fig. 16.2). After birth, it becomes transformed into a dense fibrous cord (median umbilical ligament), which runs from the urinary bladder to the umbilical region (see Fig. 18.19).
Chorion and Placenta
Formation of the placental complex represents a cooperative effort between the extraembryonic tissues of the embryo and the endometrial tissues of the mother. (Early stages of implantation of the embryo and the decidual reaction of the uterine lining are described in Chapter 3.) After implantation is complete, the original trophoblast surrounding the embryo has undergone differentiation into two layers: the inner cytotrophoblast and the outer syncytiotrophoblast (see Fig. 3.18D). Lacunae in the rapidly expanding trophoblast have filled with maternal blood, and the connective tissue cells of the endometrium have undergone the decidual reaction (containing increased amounts of glycogen and lipids) in response to the trophoblastic invasion.
Formation of Chorionic Villi
In the early implanting embryo, the trophoblastic tissues have no consistent gross morphological features; consequently, this is called the period of the previllous embryo. Late in the second week, defined cytotrophoblastic projections called primary villi begin to take shape (see Fig. 5.2). Shortly thereafter, a mesenchymal core appears within an expanding villus, at which point it is properly called a secondary villus (Fig. 7.4). Surrounding the mesenchymal core of the secondary villus is a complete layer of cytotrophoblastic cells, and outside of that is the syncytiotrophoblast. By definition, the secondary villus becomes a tertiary villus when blood vessels penetrate its mesenchymal core and newly formed branches. This event occurs toward the end of the third week of pregnancy.
The terminal portion of a villus remains trophoblastic, consisting of a solid mass of cytotrophoblast called a cytotrophoblastic cell column (see Fig. 7.4) and a relatively thin covering of syncytiotrophoblast over that. The villus is bathed in maternal blood. A further development of the tip of the villus occurs when, under the influence of the local hypoxic environment, the cytotrophoblastic cell column expands distally and penetrates the syncytiotrophoblastic layer (Fig. 7.5). These cytotrophoblastic cells abut directly on maternal decidual cells and spread over them to form a complete cellular layer known as the cytotrophoblastic shell, which surrounds the embryo complex. The villi that give off the cytotrophoblastic extensions are known as anchoring villi (see Fig. 7.4) because they represent the real attachment points between the embryo complex and the maternal tissues.
It is important to understand the overall relationships of the various embryonic and maternal tissues at this stage of development (see Fig. 7.5). The embryo, attached by the body stalk, or umbilical cord, is effectively suspended in the chorionic cavity. The chorionic cavity is bounded by the chorionic plate, which consists of extraembryonic mesoderm overlaid with trophoblast. The chorionic villi extend outward from the chorionic plate, and their trophoblastic covering is continuous with that of the chorionic plate. The villi and the outer surface of the chorionic plate are bathed in a sea of continually exchanging maternal blood. Because of this, the human placenta is designated the hemochorial type.*
Although chorionic villi are structurally very complicated, it is convenient to liken the basic structure of a villus complex to the root system of a plant. The anchoring villus is equivalent to the central tap root; by means of the cytotrophoblastic cell columns, it attaches the villus complex to the outer cytotrophoblastic shell. The unattached branches of the floating villi (see Fig. 7.12) dangle freely in the maternal blood that fills the space between the chorionic plate and the outer cytotrophoblastic shell. All surfaces of the villi, chorionic plate, and cytotrophoblastic shell that are in contact with maternal blood are lined with a continuous layer of syncytiotrophoblast.
Establishing the Uteroplacental Circulation
One of the critical features of the developing embryonic-maternal interface is the establishment of a uteroplacental circulation that serves as the medium for bringing food and oxygen to and removing wastes from the embryo. This is accomplished by erosion of the walls of the spiral arteries of the uterus and their modification so that, as the embryo grows, these arteries can provide an increasing flow of blood at low pressure to bathe the syncytiotrophoblastic surface of the placenta (see Fig. 7.10). Specialized invasive cytotrophoblastic cells, migrating out from the anchoring villi, invade the spiral arteries (but not the veins) and cause major modifications of their walls by secreting a specialized extracellular matrix and displacing many of the normal cellular elements of the spiral arteries. As a result, the arteries become wider, but the blood escaping from their open ends leaves at a much lower pressure than normal arterial pressure.
Hypoxia stimulates cytotrophoblastic cells to undergo mitosis. This may be one of the environmental conditions that underlies the rapid growth of the cytotrophoblast during the early embryonic period. After 12 weeks, when the maternal blood in the placental space contains large numbers of erythrocytes and is more highly oxygenated, the fetal erythrocytes, through an isoform switch, begin to produce fetal hemoglobin, which requires higher oxygen tension to bind oxygen efficiently. The maternal blood that leaves the spiral arteries freely percolates throughout the intervillous spaces and bathes the surfaces of the villi. The maternal blood is then picked up by the open ends of the uterine veins, which also penetrate the cytotrophoblastic shell (see Fig. 7.10).
Gross Relationships of Chorionic and Decidual Tissues
Within days after implantation of the embryo, the stromal cells of the endometrium undergo a striking transformation called the decidual* reaction. After the stromal cells swell as the result of the accumulation of glycogen and lipid in their cytoplasm, they are known as decidual cells (Fig. 7.6). The decidual reaction spreads throughout stromal cells in the superficial layers of the endometrium. The maternal decidua are given topographic names based on where they are located in relation to the embryo.
The decidual tissue that overlies the embryo and its chorionic vesicle is the decidua capsularis, whereas the decidua that lies between the chorionic vesicle and the uterine wall is the decidua basalis (Fig. 7.7). With continued growth of the embryo, the decidua basalis becomes incorporated into the maternal component of the definitive placenta. The remaining decidua, which consists of the decidualized endometrial tissue on the sides of the uterus not occupied by the embryo, is the decidua parietalis.
In human embryology, the chorion is defined as the layer consisting of the trophoblast and the underlying extraembryonic mesoderm (see Fig. 7.1). The chorion forms a complete covering (chorionic vesicle) that surrounds the embryo, amnion, yolk sac, and body stalk. During the early period after implantation, primary and secondary villi project almost uniformly from the entire outer surface of the chorionic vesicle. The formation of tertiary villi is asymmetric, however, and the invasion of the cytotrophoblastic core of the primary villi by mesenchyme and embryonic blood vessels occurs preferentially in the primary villi located nearest the decidua basalis. As these villi continue to grow and branch, the villi located on the opposite side (the abembryonic pole) of the chorionic vesicle fail to keep up and eventually atrophy as the growing embryo complex bulges into the uterine cavity (see Fig. 7.7). The region that contains the flourishing chorionic villi and that ultimately becomes the placenta is the chorion frondosum. The remainder of the chorion, which ultimately becomes smooth, is the chorion laeve (Fig. 7.8).
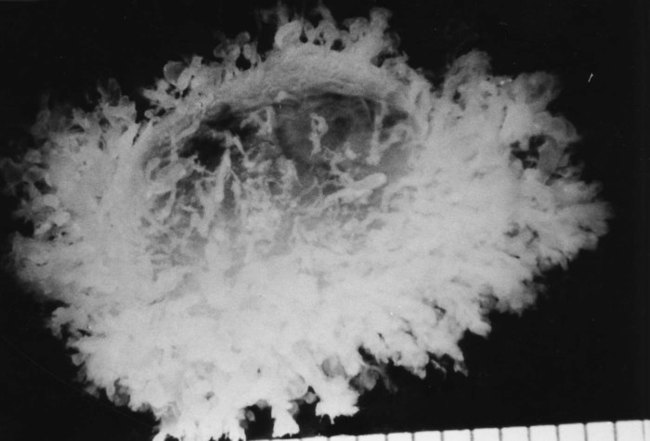
The small, bare area in this photograph of a human chorionic vesicle is a region where the chorionic villi have atrophied. This area will enlarge in succeeding weeks. (From Gilbert-Barness E, ed: Potter’s pathology of the fetus and infant, St Louis, 1997, Mosby.)
The overall growth of the chorionic vesicle (Fig. 7.9), with its bulging into the uterine lumen, pushes the decidua capsularis progressively farther from the endometrial blood vessels. By the end of the first trimester, the decidua capsularis itself undergoes pronounced atrophy. Within the next month, portions of the atrophic decidua capsularis begin to disappear and leave the chorion laeve in direct contact with the decidua parietalis on the opposite side of the uterus (see Fig. 7.7). By midpregnancy, the decidua capsularis has fused with the tissues of the decidua parietalis, thereby effectively obliterating the original uterine cavity. While the chorion laeve and decidua capsularis are undergoing progressive atrophy, the placenta takes shape in its definitive form and acts as the main site of exchange between the mother and embryo.
Formation and Structure of the Mature Placenta
As the distinction between the chorion frondosum and chorion laeve becomes more prominent, the limits of the placenta proper can be defined. The placenta consists of a fetal and a maternal component (Fig. 7.10). The fetal component is the part of the chorionic vesicle represented by the chorion frondosum. It consists of the wall of the chorion, called the chorionic plate