Chapter 61 Pediatric Retinal Vascular Diseases
For additional online content visit http://www.expertconsult.com
Retinopathy of prematurity
Historical perspective
Early history
ROP was first described in 1942,1,2 and quickly became the primary cause of childhood blindness throughout the developed world.3 Terry’s original reports designated the condition retrolental fibroplasia (RLF), based on his impression that it involved a proliferation of the embryonic hyaloid system, but Owens and Owens4 found that the hyaloid system was normal at birth and that RLF developed postnatally. As the pathogenesis and clinical manifestations became better understood, the term “retinopathy of prematurity” was adopted.
The discovery of the relationship between supplementary oxygen and ROP in the 1950s5–9 led to rigid curtailment of oxygen supplementation in the nursery, and a dramatic decrease in ROP incidence followed. Unfortunately this had an adverse effect on infant morbidity and mortality (e.g., respiratory distress syndrome, cerebral palsy, neurologic disorders).10–12
Retinopathy of prematurity and contemporary nursery practices
By the early 1970s, arterial blood gas analysis had come into general use, and the oxygen requirements of premature infants with respiratory distress syndrome were better documented.13 This enabled pediatricians to titrate the incubator oxygen concentration to more nearly meet the individual premature infant’s oxygen needs.
Modern transcutaneous oxygen monitoring and continuous pulse oximetry have provided additional noninvasive tools, allowing neonatologists to monitor babies in real time more closely. These have stimulated studies, including large mutlicenter oygen restriction trials, which have found that lower target oxygen saturation levels (e.g., 85–89%) correlate with significantly improved rates of severe ROP but are also associated with increased mortality rates.14–16 At this time, general consensus regarding target oxygen saturation levels that balance ROP risk and mortality has not been reached.
With improvements in neonatology practice, more of the smallest premature infants are now surviving. Eight percent of low-birth-weight infants survived in 1950, but with ventilators, surfactant, intravenous nutrition, and other gains in knowledge, survival has risen to 37–72%.17–19 Clearly, infants are surviving today with more immature retinal vasculature and therefore higher ROP risk. For this reason, and because of common pathophysiology with other proliferative vascular diseases such as diabetic retinopathy, there has been mounting interest in ROP.20–22
The role of oxygen
Clinical findings
Results of controlled nursery studies5,8 that suggested supplementary oxygen to be the principal cause of ROP in the epidemic of the early 1950s were confirmed, and the role of prolonged oxygen was documented in a collaborative randomized controlled trial.9
Since that time, attempts to delineate the critical blood oxygen levels producing ROP have not resulted in definitive conclusions. In a prospective study of 589 infants monitored by intermittent blood gas measurements, and where clinical goals were to avoid elevated arterial oxygen, the occurrence of ROP was not related to arterial oxygen levels.23 Only the duration of oxygen exposure was a risk factor. Somewhat unexpectedly, continuous transcutaneous monitoring of blood oxygen levels was found in one study to be of no more value than intermittent monitoring in preventing visual disability.24 It was suggested by Lucey and Dangman25 that therapeutic oxygen, although important, has been overemphasized as a cause of ROP in contemporary neonatal practice.* They emphasized that other factors related to very low birth weight are also important, especially in view of current nursery oxygen monitoring.
Recent studies, including large mutlicenter oygen restriction trials, have found that lower target oxygen saturation levels (e.g., 85–89%) correlate with significantly improved rates of severe ROP, but are associated with increased mortality rates.14–16 At this time, a consensus about optimal target oxygen saturation levels has not been reached.
Experimental findings
In the early 1950s the laboratory kitten model, which produced lesions resembling the early stages of human ROP, was used extensively because it demonstrated selective response to oxygen by the immature retinal vessels.6,7 In the full-term newborn kitten, the immature retinal vascularization is comparable to that of a human fetus at 6 months’ gestation, thus providing the unique opportunity to study the response to oxygen of the immature retina, albeit in a full-term, healthy animal.3 When hyperoxia studies were extended to other animal models, such as the young mouse and puppy,6 the general concept of oxygen toxicity to immature retinal vessels was reinforced.
Investigators26,27 have pointed out the histologic differences in the retinas of these animal models from the human but were unable to explain why progression to retinal detachment did not occur. It is noteworthy that McLeod et al. reported the production of intravitreal neovascularization with traction retinal folds in young dogs exposed to hyperoxia.28 These findings add to the potential application of the canine model to investigate these stages of ROP (Figs 61.1 and 61.2 online).
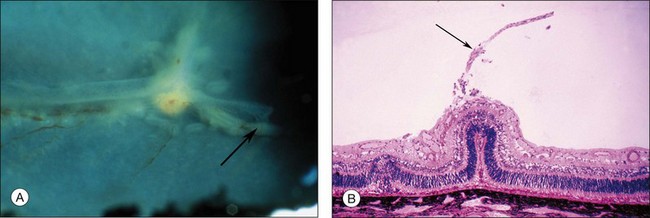
Fig. 61.2 (A) Gross appearance of the areas of neovascularization extending temporally and two smaller areas in the same 45-day-old dog shown in Figure 61.1. A denser 2 × 0.5 mm area is present inferior nasally (arrow). (B) Area from A (marked by arrow) discloses retinal neovascularization (arrow) attached to the apex of a retinal fold (periodic acid–Schiff and hematoxylin; ×125).
The hyperoxic animal models demonstrated that only the incompletely vascularized retina was susceptible to oxygen’s adverse effect, and that the more immature the vascularization, the greater the pathologic response to oxygen.29 These findings supported the clinical observation that the infant with a less mature retina has greater ROP susceptibility, and that the infant with a fully vascularized retina has no risk. Accordingly, the temporal retina, the last part to vascularize, remains susceptible to ROP the longest (Fig. 61.3 online).
Mechanism of oxygen’s effects on the immature retina
Primary stage of retinal vasoconstriction and vascular occlusion
The primary effect of elevated blood oxygen in any retina is vasoconstriction, which, if sustained, is followed by some degree of vascular closure. In young kittens, initial vasoconstriction occurs within several minutes after oxygen exposure. Vascular caliber is reduced by approximately 50% initially, but then rebounds to its original dimensions. Continued oxygen exposure results in gradual vasospasm during the next 4–6 hours, until the vessels are approximately 80% constricted.30 At this stage, constriction is still reversible. However, if significantly elevated arterial oxygen partial pressure levels persist for an additional period (e.g., 10–15 hours), some immature peripheral vessels are permanently occluded.7,23
This occlusion progresses as the duration of hyperoxia increases, and local vascular obliteration is complete after 2–3 days of exposure. In the dog, after 4 days of exposure to hyperoxia, most capillaries are lost and only major blood vessels survive.31
Electron microscopic observations demonstrate selective hyperoxic injury to the endothelial cells of the most immature vessels, without obvious changes in the neuronal elements of the retina.32
Secondary stage of retinal neovascularization
After removal of the laboratory animal to ambient air following sustained hyperoxia, marked endothelial proliferation arises from the residual vascular complexes adjacent to retinal capillaries ablated during hyperoxia (Fig. 61.4 online). This can be demonstrated on fluorescein angiography (Fig. 61.5). Nodules of proliferating endothelial cells canalize to form new vessels that not only grow within the retina, but also erupt through the internal limiting membrane to grow on its surface, similar to the neovascularization in other proliferative retinopathies (Figs 61.6–61.9). In the dog and cat, the initial preretinal neovascular formations are like angioblastic masses with few lumens (formations sometimes called “popcorn”), which mature into neovascular formations that include vessels invested with pericytes.33,34 Although the neovascularization may be extensive, this is generally the maximum response to oxygen in the kitten model and is followed by progressive vascular remodeling and involution of abnormalities. The preretinal neovascularization in the dog persists and can develop into tented membranes which create tractional retinal folds in the retina (Figs 61.1 and 61.2).28 The mouse and rat preretinal neovascular formations, however, will regress after 5 days.30–35–37 Although regression is rapid and spontaneous in mice, the mouse model has been useful in evaluating topical38 and systemic drugs,39,40 experimental gene therapy strategies41,42 and endogenous inhibitors like pigment epithelial growth factor.43
Oxygen exerts an important effect on the remodeling of the original primitive capillary network that develops in the retina.44 Capillaries regress from areas of higher oxygen concentration and grow toward areas of lower oxygen. Penn et al.45 used experimentally alternating periods of high and low oxygen in the rat pup model to produce a more proliferative form of retinopathy. Pierce and colleagues21 used hyperoxia and hypoxia in a mouse pup model to demonstrate the correlation of vascular endothelial growth factor (VEGF) protein production with periods of low oxygen, and its disappearance during oxygenation.
Pathogenesis
Normal retinal vasculogenesis
It is appropriate to review normal retinal vascular development as background for understanding ROP pathogenesis. Michaelson33 originally suggested that retinal capillaries arise by budding from pre-existent arteries and veins that originate from the hyaloid vessels at the optic nerve head. Cogan34 proposed a similar mechanism, except for the hypothesis of budding of solid endothelial cords from hyaloid vessels. Ashton37 suggested that mesenchyme, the blood vessel precursor, grows from the optic disc through the nerve fiber layer to the periphery of the retina. Mesenchymal precursors have recently been observed far in advance of formed blood vessels in human fetal retinas.46 On the posterior edge of the advancing mesenchyme, a “chicken-wire” meshwork of capillaries develops, and undergoes absorption and remodeling to produce mature retinal arteries and veins that are surrounded by the capillary meshwork.37,44 Variations in capillary development may be species-specific. However, across all species studied to date, VEGF appears to be a key factor guiding vascular growth, which most closely fits the identity of Michaelson’s proposed “factor X.”33 In the kitten, Chan-Ling and Stone demonstrated the role of astrocytes leading to growth of the capillary network.47–49 Provis et al.50 demonstrated the expression of VEGF message in the predicted location in the developing normal human retina, just anterior to the developing vessels (Fig. 61.10).
Figure 61.11 shows the normal rate of progression of the retinal vessels into the far retinal periphery in human premature infants without ROP according to their postconceptional age (gestational age at birth plus chronologic age). More than 80% of prematurely born infants have been observed to develop this relatively mature retinal vasculature by the time they reach full term.
Pathogenesis of ROP
Initial changes in the developing vessels are described above, and historically this was believed to be an injury initiated by “excess” oxygen. Alon et al. demonstrated that hyperoxia caused downregulation of VEGF and death of endothelial cells, suggesting that VEGF is an endothelial survival factor.51 In the time that follows closure of these growing vessels, the differentiating retina becomes increasingly ischemic and hypoxic and VEGF is upregulated52,53 driving the neovascularization.48
Theoretically, the provision of increased oxygen should downregulate the release of such growth factor(s) and permit neovascularization to remodel and regress in an orderly fashion. Szewczyk54 proposed this, and treated infants with significant ROP by returning them to oxygen. With no controls, it is difficult to know from his report if this success was simply due to spontaneous involution of ROP. This hypothesis was tested in the kitten model of oxygen-induced retinopathy. Systemic mild hypoxia was found to worsen the retinopathy,55 whereas mild hyperoxia improved it.56 With National Institutes of Health sponsorship, the multicenter Supplemental Therapeutic Oxygen for Prethreshold ROP (STOP-ROP) trial, chaired by Dale L. Phelps, found that, once the ROP was established, raising the oxygen saturation mildly did not harm the ROP, but neither was it of clear benefit.57
The clinical and histopathologic observations of Flynn and coworkers58–62 led them to postulate the following sequence of events in human ROP pathogenesis:
1. Endothelial injury occurs where it has just differentiated from mesenchyme to form the primitive capillary meshwork. This is reminiscent of animal studies in which a short duration of hyperoxia resulted in capillary damage limited to the most recently differentiated vascular complexes (Fig. 61.4A). It is currently believed that environmental factors other than oxygen also are involved. For example, Brooks and associates found that nitric oxide may contribute to the vaso-obliterative stage of ROP,63 while Alon et al. found that reduced VEGF may result in death of endothelial cells51 because of its role as a survival factor.
2. After this injury to the vascular endothelium, the mesenchyme and mature arteries and veins survive and merge via the few remaining vascular channels to form a mesenchymal arteriovenous shunt which replaces the destroyed or damaged capillary bed.
3. The mesenchymal arteriovenous shunt is located at the demarcation between the avascular and vascularized retina. It consists of a nest of primitive mesenchymal and maturing endothelial cells that are fed by mature arteries and veins. No capillaries are found in the region of the shunt. Flynn60 suggested that this structure represents the pathognomonic lesion of acute ROP.
Flynn described a dormant period after the injury (days to months), during which retinal findings are relatively stable. The tissues comprising the shunt may thicken, and the gray-white initial color of the structure turns from pink to salmon to red. He stated: “during this period when vasculogenic activity resumes in the retina, the fate of the eye is decided.”60 Flynn pointed out that when the cells inside the shunt divide and differentiate into normal capillary endothelium, they form primitive endothelial tubes that send forth a brush border of capillaries that grows anteriorly into the avascular retina. This represents ROP involution, which he observed to occur in more than 90% of cases at this early stage. In progressive disease, however, the primitive cells inside the shunt proliferate and erupt through the internal limiting membrane, growing on the surface of the retina and into the vitreous body. Flynn stated: “it is this lack of differentiation and destructive proliferation of cells and their invasion into spaces and tissues where they do not belong that is the chief event in the process of membrane proliferation leading to traction detachment.”60
Foos64–66 suggested a pathogenesis of ROP based on examination of histopathologic material. He used the terms “vanguard” and “rearguard” to describe cellular components of the developing retina. The vanguard (anterior) component contains spindle-shaped cells thought to be glia, which play a role in nourishing the immature retina during development.67 The rearguard contains primitive endothelial cells. As the retina matures, the endothelial cells aggregate into cords that, according to Foos,66 subsequently lumenize and become the primordial capillaries of the retina. It is from the rearguard and primitive endothelial cells that neovascularization of ROP develops (Figs 61.12 and 61.13). Foos noted that, as the developing vasculature reaches its most anterior extent and matures, the spindle cells of the vanguard disappear. The work of Chan-Ling et al.,46 McLeod et al.,68 and Provis et al.50 showed that spindle cells are endothelial precursors and, in fetal human and neonatal dog retina,68 the precursors organize and differentiate to form the initial retinal vasculature.46
International classification
The international classification of ROP divided the retina into three anteroposterior zones and describes the extent of disease by the 30° meridians (clock-hours) involved (Fig. 61.14). Retinal changes are divided into stages of severity, based on descriptive and photographic standards.69
Zones of involved retina
Each of the three zones of the retina is centered on the optic disc (Fig. 61.14). Zone I includes the posterior pole, and is defined as a circle, centered on the disc, whose radius is twice the distance from the disc to the macula. It subtends an arc of about 60° (Fig. 61.15). Zone II extends from the peripheral border of zone I to a concentric circle tangential to the nasal ora serrata. Temporally, this boundary corresponds approximately to the anatomic equator. Once the nasal vessels have reached the ora serrata, zone III is the remaining temporal crescent of retina anterior to zone II. Zone III, which is the farthest from the disc, is the last zone to become vascularized. It is clinically important to continue classifying ROP as zone II if there remains any active ROP or immature vessels in the nasal retina.
Extent of retinopathy of prematurity
The extent of the ROP changes is described according to the 12 30° sectors involved, labeled as hours of the clock (Fig. 61.14): the nasal side of the right eye is at 3:00, and the nasal side of the left eye is at 9:00.
Staging
Abnormal peripheral changes are divided into three stages, which may progress to retinal detachment (stages 4–5) (Figs 61.16 and 61.17).
Stage 1: demarcation line
Stage 1 is characterized by the presence of a demarcation line, the first ophthalmoscopic sign of ROP (Fig. 61.16A). This represents a structure separating the anterior, avascular retina from the posterior, vascularized retina. It appears flat and white, and lies within the plane of the retina. Abnormal branching or arcading of vessels leads up to the line. Stage 1 is relatively evanescent, generally either progressing to stage 2 or involuting to normal vascularization within several weeks. According to Garner,70 the stage 1 demarcation line morphologically comprises two relatively distinct zones. The more anterior vanguard zone is formed by a mass of spindle-shaped cells, which are the progenitors of the differentiated vascular endothelium. As such it corresponds to the primitive mesenchyme (spindle cells) seen in normal fetal development but with a considerable increase in the number of cells. It is this hyperplasia, involving both thickening and widening, that makes the demarcation line visible.70
Stage 2: ridge
In stage 2, the demarcation line has grown into a ridge with height and width, which extends centripetally within the globe (Fig. 61.16B). The ridge may be white or pink and, rarely, vessels may even leave the surface of the retina to enter it. Small tufts of new vessels (“popcorn” lesions) may be seen located posterior to the ridge structure but not attached to it. The absence of fibrovascular growth from the surface of the ridge separates this stage from stage 3. According to Garner, the stage 2 retinal ridge results from the proliferation of endothelial cells “with some evidence of organization into recognizable vascular channels.”70 Flynn et al.61 demonstrated that in this stage these channels leak fluorescein on angiographic examination.
Stage 3: ridge with extraretinal fibrovascular proliferation
Stage 3 is characterized by the addition of extraretinal, fibrovascular tissue proliferating from the former ridge (Fig. 61.16C and D). This proliferating tissue is localized continuous with the posterior and interior aspect of the ridge, causing a ragged appearance of the ridge as proliferation increases into the vitreous. As in stage 2, vessels may leave the surface of the retina to enter the ridge and could be mistaken for retinoschisis or even detachment. The presence of elevated retinal vessels coursing from the retinal surface to the height of the ridge does not alone constitute a retinal detachment;69 however, this may signify presence of vitreous traction. According to Foos,66 the stage 3 “extraretinal vascularization” may appear placoid, polypoid, or pedunculated on histological examination. The placoid pattern is the most common and also the most important because it correlates with subsequent development of retinal detachment. Foos demonstrated that these extraretinal vessels are apparently derived from proliferating endothelial cells and not from the vasoformative mesenchymal “spindle” cells based on his factor VIII preparations. He also observed significant synchysis and condensation of the vitreous body in stage 3. Foos suggested that a condensation of the vitreous body over the ridge is related to depolymerization of hyaluronic acid and collapse of the collagenous framework into optically visible structures.66
“Plus” and “pre-plus” disease
Plus disease signifies a more florid form of ROP. Increasing dilation and tortuosity of the retinal vessels, iris vascular engorgement, pupillary rigidity, and vitreous haze indicate progressive vascular incompetence. When vascular changes are so marked that the posterior veins are enlarged and the arterioles tortuous, this represents plus disease, and a plus sign is added to the ROP stage number. This finding is a key sign of worse prognosis.71 A published standard photograph selected by expert consensus, which has been used in four multicenter clinical trials and represents the minimum arteriolar tortuosity and venous dilation required for plus disease, is shown in Figure 61.18A. There has been increasing recognition of a spectrum of retinal vascular abnormalities in ROP. In 2005, the revised international classification defined an intermediate “pre-plus” categorization as abnormal arteriolar tortuosity and venous dilation of the posterior pole which is insufficient for diagnosis of plus disease.72 Studies have shown that the diagnosis of plus disease may be subjective and qualitative, even among experts, and future definitions may be based on more quantitative measures.73–75
Zone I ROP
ROP located in zone I can be dangerously deceptive, in that the proliferation signifying stage 3 can appear spread out “flat” on the retina posterior to the ridge, rather than elevated.36 In severe plus disease cases inside zone I, centripetal proliferation from the ridge may occur virtually simultaneously with detachment of the retina. Flynn and Chan-Ling examined the distinction between vasculogenesis (de novo formation of new vessels by transformation of vascular precursor cells) and angiogenesis (budding from existing vessels) with regard to the distinction of zone I and zone II ROP. They proposed that zone I ROP correlates with vasculogenesis, and is therefore less sensitive to treatment by laser or cryotherapy because the disease mechanism is not VEGF-mediated. They proposed that zone II ROP corelates with angiogenesis, is mediated by hypoxia-induced VEGF-165, and is therefore more sensitive to treatment by laser or cryotherapy.76
Aggressive posterior ROP
The 2005 revised international classification of ROP designated an uncommon, severe form of disease as “aggressive posterior ROP.” This rapidly progressive disease variant had been previously termed “rush disease,” and is characterized by its location in zone I or posterior zone II, ill-defined nature of the peripheral retinopathy, and prominent plus disease out of proportion to the peripheral findings.72 This diagnosis can be made by a single examination without serial evaluation, and may not progress through the class stages 1–3. In fact, the peripheral disease may appear as a flat area of neovascularization at the junction of vascular and avascular retina.
Classification of retinal detachment
In 1987, ophthalmologists and pathologists established a second international committee, which expanded the original 1984 international classification to describe the morphology, location, and extent of retinal detachment (Fig. 61.17).77 This classification was based on an understanding of the development of severe ROP gained from surgical experience78 and pathology.66 Stage 4 (subtotal) retinal detachment is usually tractional elevation added to findings in stage 3, although there may also be exudative effusion from adjacent active stage 3 neovascularization.
Stage 4A: extrafoveal retinal detachment
Typically this is a concave, traction detachment in the peripheral retina without involvement of the central macula (Fig. 61.19). Generally, these detachments are located at the sites of extraretinal fibrovascular proliferation with associated vitreous traction. Elevation may start in any zone where there was stage 3 disease that incompletely involuted following ablative treatment with laser photocoagulation or cryotherapy, and they may become circumferential. They may extend for 360° in the periphery without elevation of the macula, or they may be segmental, occupying only a portion of the periphery. The prognosis anatomically and visually is relatively good in the absence of posterior extension. Frequently these areas will reattach spontaneously, without affecting macular function.
Stage 4B: partial retinal detachment including the fovea
This can follow extension of stage 4A, or may appear as a fold from the disc through zone I to zones II and III (Fig. 61.20). Once a stage 4 detachment involves the fovea, the prognosis for recovery of good visual acuity is poor.
Stage 5: total retinal detachment
This is virtually always funnel-shaped. The classification of stage 5 detachments divides the funnel into an anterior and a posterior part (Fig. 61.17). When open both anteriorly and posteriorly, the detachment has a concave configuration and extends to the optic disc. An alternative configuration is one in which the funnel is narrow both anteriorly and posteriorly, and the detached retina is located just behind the lens. A third, less common type is one in which the funnel is open anteriorly but narrowed posteriorly. Least common is a funnel that is narrow anteriorly and open posteriorly. The configuration of the funnel-shaped detachment may be appreciated by ultrasonography.
Other factors related to retinal detachment
1. Appearance of the retrolenticular space. This space may be occupied by heavily vascularized translucent tissue, which represents disease activity. As the disease subsides, the tissue occupying this space becomes white, with a scarcity of blood vessels. This is the appearance that gave rise to the original term “retrolental fibroplasia.”
2. Peripheral trough. The presence of a peripheral red reflex in combination with apparent narrow funnel stage 5 retinal detachment indicates the presence of attached or shallowly detached avascular, stretched, and nonfunctioning peripheral retina. Occasionally, we have seen a peripheral red reflex coming from choroid beneath detached retina that has become adherent to the posterior lens capsule.
3. Anterior segment. In more severe cases of ROP, the anterior segment may become involved as follows:
4. Other tissues. Subretinal blood and exudate may be identifiable by ultrasonography or optical coherence tomography, but it may be difficult to distinguish one from another. Subretinal fibrotic membranes may be present but usually are recognized only during surgery.
Involution of retinopathy of prematurity
Involution of ROP typically begins after 38 weeks’ postconceptional/postmenstrual age, and may be characterized by a downgrading of staging and/or growth of retinal vessels into a more peripheral zone.79
Regressed rop: retinal detachment, strabismus, and amblyopia
Although active ROP usually involutes without progressing to retinal detachment, cicatricial sequelae can remain even in those cases.80,81 The relatively stable state of the eye after retinopathy has run its course is referred to as regressed ROP. In Box 61.1 the residual changes have been classified into those affecting the retinal periphery and those affecting the posterior fundus. Retinal pigmentary changes may be mistaken for side-effects of treatment.82