Chapter 21 Overview of Disorders of Brain Development
Introduction




Thus, an understanding of brain malformations is important in assessing almost all types of neurological disorders in children. On a practical basis, we have separated brain malformations into disorders involving development of the neural tube (Chapter 22), forebrain (Chapter 23), and mid-hindbrain (Chapter 24); disorders of cortical development, separated into those of brain size (Chapter 25) and those of migration and later cortical development (Chapter 26); disorders predisposing to hydrocephalus (Chapter 27); and disorders of skull development (Chapter 28). We end with an overview of prenatal diagnosis for all brain malformations (Chapter 29). Here, we will address a few topics relevant to brain malformations generally, including epidemiology, classification, clinical recognition, relationship to other neurological disorders and selected environmental factors, and genetic counseling.
Epidemiology
The incidence of brain malformations has been estimated to be approximately 3.32 per 1000 and the prevalence approximately 2.21 per 1000 at age 14 years from studies of a 1-year birth cohort from northern Finland [Von Wendt and Rantakallio, 1986]. These are much higher rates than were recognized in the era before magnetic resonance imaging (MRI) and recent increases in surgical treatment of hydrocephalus and epilepsy [Kuzniecky et al., 1993; Massimi et al., 2009; Warkany et al., 1981]. Not surprisingly, the incidence is much higher (i.e., approximately 88 per 1000) in studies of children with cerebral palsy [Rankin et al., 2010]. This is an important point. To emphasize this, a boy was recently evaluated with apparent cerebral palsy attributed to prematurity at approximately 29 weeks’ gestation. But his brain MRI revealed mild callosal and cerebellar vermis hypoplasia, and chromosome microarray revealed a small deletion in 22q11.2, which implies either a genetic or a mixed pathogenesis.
Classification
While the chapters that follow review many different malformations, they are not complete, as the number of recognized malformations continues to grow steadily. Presenting these data is also complicated by the tendency for malformations to co-occur in some patients. For example, Figure 21-1 shows a striking example of a boy with malformations of the forebrain (agenesis of the corpus callosum), mid-hindbrain (severe cerebellar hypoplasia and mega-cisterna magna), brain size (megalencephaly), neuronal migration (periventricular nodular heterotopia), and cortical organization (polymicrogyria overlying the heterotopia).
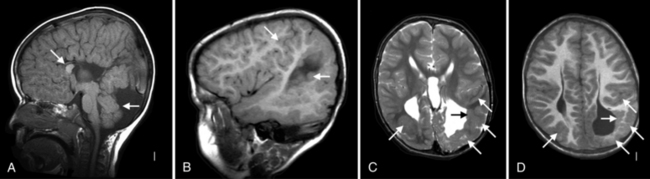
Fig. 21-1 Brain images from a single patient showing multiple malformations.
(Courtesy of WB Dobyns, research subject LR00-086.)
From time to time, flexible classification schemes have been constructed for many of these malformations that primarily rely on traditional concepts such as embryology and anatomy, with a contribution from genetic discoveries. While recent discoveries are leading us to think more of genes and gene pathways than of embryology and anatomy, a more traditional classification scheme is presented in this and the following chapters. The most current outline for brainstem and cerebellar (mid-hindbrain) malformations is shown in Box 21-1, and an outline for cortical malformations in Box 21-2. Further details regarding most subgroups of malformations and the basis for the classification are given in the primary references [Barkovich et al., 2005, 2009]. These schemes were constructed relying on – in decreasing order of priority – the underlying genetic basis when known, the relevant embryology, brain imaging features, and miscellaneous other clinical features.
Box 21-1 Classification Scheme for Mid-Hindbrain Malformations