Optic Nerve
Congenital Defects and Anatomic Variations
Aplasia
I. Aplasia of the optic nerve (Fig. 13.3) is rare, especially in eyes without multiple congenital anomalies.
IV. Histology
Hypoplasia
I. Although rare, hypoplasia (underdevelopment of the optic nerve) is more common than aplasia (congenital absence of the optic nerve).
A. Hypoplasia of the optic nerve is a major cause of blindness in children.
B. In optic nerve hypoplasia, a small optic disc with central vessels is present.
III. Visual acuity is generally markedly decreased.
IV. The cause is failure of the retinal ganglion cells to develop normally.
B. Histologically, the nerve shows partial or complete absence of neurites.
Dysplasia
Anomalous Shape of Optic Disc and Cup
A. Oval discs (vertically, horizontally, or obliquely elongated) are common.
B. They are congenital and nonprogressive.
II. Myopia (see later in this chapter and see Chapter 11)
III. Congenital excavation of optic disc (i.e., an exaggeration of physiologic cup)
IV. Pseudoneuritis or pseudopapilledema (i.e., the opposite of congenital excavation)
B. Often, it is associated with hypermetropia or drusen of the optic nerve head.
Congenital Crescent or Conus
Congenital (Familial) Optic Atrophies
I. Simple recessive congenital optic atrophy
A. It has an autosomal-recessive inheritance pattern and significant visual disability.
C. Histology—see Optic Atrophy later in this chapter.
II. Behr’s syndrome
B. One form of Behr’s syndrome has been reported in Iraqi Jews who have 3-methylglutaconic aciduria.
C. Histology—see Optic Atrophy later in this chapter.
III. Dominant optic atrophy (Kjer)
B. The visual loss in dominant optic atrophy (Kjer type) has an insidious onset in approximately the first five years of life, with considerable variation in families. Approximately 58% of affected patients have onset of symptoms before the age of 10 years.
1. Long-term visual prognosis is relatively good, with stable or slow progression of visual loss.
C. Histology—see Optic Atrophy later in this chapter.
IV. Leber’s hereditary optic neuropathy (LHON)
A. LHON, one of the mitochondrial myopathies (see Chapter 14), is inherited through the maternal transmission of one or more mitochondrial DNA (mtDNA) mutations.
1. At least 11 pathogenetic point mutations of mtDNA have been described.
4. Class I/II contains two mutations that have an intermediate effect between classes I and II.
C. LHON is characterized by a subacute, sequential, bilateral, central loss of vision mainly in young men (usually between the ages of 18 and 30 years). Color vision is affected early.
D. Histology—see Optic Atrophy later in this chapter.
Coloboma (Table 13.1)
I. A coloboma (Figs. 13.4 and 13.5) may involve the optic disc alone or may be part of a complete coloboma involving the entire embryonic fissure.
B. The surrounding retina may be involved.
IV. Vision may be normal but is usually defective.
V. Histologically, the coloboma appears as a large defect at the side of the nerve usually involving the neural retina, choroid, and sclera.
B. The wall of the defect may contain adipose tissue and even smooth muscle cells.
C. The coloboma may protrude into the retrobulbar tissue and cause microphthalmos with cyst (see Figs. 13.4B and 13.4C and Chapter 14).
VI. An optic nerve pit (see Fig. 13.5) is a form of coloboma of the optic nerve that shows a small, circular or triangular depression approximately one-eighth to one-half the diameter of the optic disc, usually located in the inferotemporal quadrant of the disc.
B. The optic disc is usually of greater size than the one in the uninvolved fellow eye.
VII. Morning glory syndrome (see Fig. 13.4A) is a form of coloboma of the optic nerve that shows an enlarged, deeply excavated optic disc, resembling the morning glory flower.
A. Although the condition is usually unilateral, rare bilateral cases have been reported.
B. Girls are affected twice as often as boys, and visual acuity is usually poor.
D. The demarcation of the elevated peripapillary tissue and normal surrounding retina is indistinct.
VIII. Choristoma
A. Rarely, choristomatous elements can be found in the optic nerve in the absence of a coloboma.
TABLE 13.1
Ophthalmoscopic Findings That Distinguish Morning Glory Disk Anomaly from Optic Disk Coloboma
Morning Glory Disk | Optic Disk Coloboma |
(From Brodsky MC: Congenital optic disk anomalies. Surv Ophthalmol 39:89, 1994.)
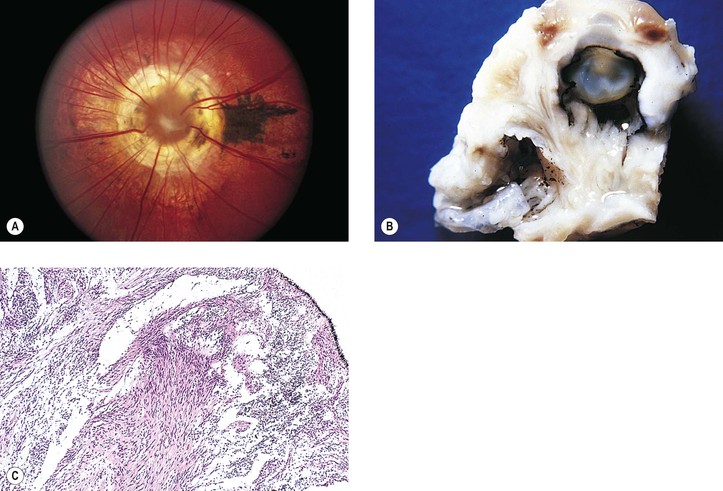
Myopia
I. Even before the onset of juvenile myopia, children of myopic parents have longer-than-normal eyes (Fig. 13.6; see Chapter 11).
IV. Histologically, the optic nerve passes obliquely through the scleral canal.
A. Temporal side of optic disc
1. The RPE and Bruch’s membrane do not extend to the temporal margin of the optic disc.
Optic Disc Edema
General Information (Fig. 13.7; see Fig. 13.22)
Causes
Pseudopapilledema
Optic disc edema may be simulated by hypermetropic optic disc, drusen of optic nerve head, congenital developmental abnormalities, optic neuritis and perineuritis, and myelinated (medullated) nerve fibers.
Histology of Optic Disc Edema
I. Acute (see Fig. 13.7)
A. Edema and vascular congestion of the nerve head result in increased tissue volume.
1. Hemorrhages may be seen in the optic nerve or in the retinal nerve fiber layer.
2. The increased tissue mass causes the physiologic cup to narrow.
B. The aforementioned changes result in a displacement of the neural retina away from the edge of the optic disc.
1. The outer layers of the neural retina may buckle (retinal and choroidal folds are seen clinically).
2. The rods and cones are displaced away from the end of Bruch’s membrane.
II. Chronic
Optic Neuritis
In general, visual acuity is severely affected.
Causes
I. Secondary to ocular disease (e.g., acute corneal ulcer, anterior or posterior uveitis, endophthalmitis or panophthalmitis, and retinochoroiditis; see Fig. 4.26)
II. Secondary to orbital disease [Fig. 13.8; e.g., as bilateral idiopathic inflammation of the optic nerve sheaths, cellulitis (may be primary but more commonly secondary to sinusitis), thrombophlebitis, arteritis, and midline granuloma syndrome]
III. Secondary to intracranial disease (e.g., meningitis, encephalitis, and meningoencephalitis)
V. Secondary to vascular disease [Figs. 13.9 and 13.10; e.g., temporal arteritis, periarteritis nodosa, pulseless (Takayasu’s) disease, and arteriosclerosis]
A.