Obstructive Lung Disease
Chronic Obstructive Pulmonary Disease (COPD), Asthma, and Related Diseases
After reading this chapter you will be able to:
State definitions of chronic obstructive pulmonary disease (COPD), asthma, and bronchiectasis.
Identify how many Americans are diagnosed with COPD and how many deaths from COPD occur each year.
Understand the major risk factors associated with the onset of COPD.
Identify the common signs and symptoms associated with COPD.
Describe a treatment plan for a patient with stable COPD and for a patient with an acute exacerbation.
State the factors associated with the onset of asthma.
Describe the typical clinical presentation of a patient with asthma.
Identify the treatment currently available for a patient with acute asthma.
Describe the treatment currently available for patients with bronchiectasis.
Chronic Obstructive Pulmonary Disease
Overview and Definitions
The term chronic obstructive pulmonary disease (COPD), or sometimes chronic obstructive lung disease (COLD), refers to a disease state characterized by the presence of incompletely reversible airflow obstruction. New guidelines by the American Thoracic Society (ATS) and the Global Initiative for Chronic Obstructive Lung Disease (GOLD) guidelines recommend the use of the term COPD to encompass both chronic bronchitis and emphysema. The ATS guidelines statement regarding COPD define this entity as follows1:
Similarly, the GOLD guidelines define COPD as “a disease state characterized by airflow limitation that is not fully reversible, is usually progressive, and is associated with an abnormal inflammatory response of the lungs to noxious particles or gases.”2
The spectrum of COPD is shown in Figure 23-1, which presents a nonproportional Venn diagram representing the major components of COPD—chronic bronchitis and emphysema. Although asthma is no longer conventionally considered to be part of the spectrum of COPD, the diagram shows that considerable overlap between asthma and COPD exists. In actual practice, individuals with a history of asthma but with incompletely reversible airflow obstruction may be indistinguishable from patients with COPD.
The two major entities constituting COPD—emphysema and chronic bronchitis—are defined in different ways. Emphysema is defined in anatomic terms as a condition characterized by abnormal, permanent enlargement of the airspaces beyond the terminal bronchiole, accompanied by destruction of the walls of the airspaces without fibrosis. Chronic bronchitis is defined in clinical terms as a condition in which chronic productive cough is present for at least 3 months per year for at least 2 consecutive years. The definition specifies further that other causes of chronic cough (e.g., gastroesophageal reflux, asthma, and postnasal drip) have been excluded. Figure 23-1 shows considerable overlap between chronic bronchitis and emphysema and some overlap with asthma—that is, when airflow obstruction is incompletely reversible. Figure 23-1 also shows that chronic bronchitis and emphysema can occur without airflow obstruction, although the clinical significance of these diseases usually stems from obstruction to airflow.
Epidemiology
COPD is one of the most frequent causes of morbidity and mortality worldwide.3 The World Health Organization predicts that COPD will become the fifth most prevalent disease in the world and the fourth leading cause of worldwide mortality by 2020. In the United States, COPD is currently the third leading cause of death; it was responsible for 145,075 deaths in 2008.4 Estimates suggest that 24 million Americans are affected.4–6 Data from the National Health and Nutrition Examination Survey (NHANES) suggest that among adults 25 to 75 years old in the United States, mild COPD (defined as forced expiratory volume in 1 second [FEV1]/forced vital capacity [FVC] <70%, and FEV1 >80% predicted) occurs in 6.9% and moderate COPD (defined as FEV1/FVC <79% and FEV1 ≤80% predicted) occurs in 6.6%.3 COPD prevalence increases with aging, with a fivefold increased risk for adults older than 65 years compared with adults younger than 40 years, and some studies estimate a prevalence of 20% to 30% in adults older than 70 years.7
The growing health burden from COPD is caused in part by the aging of the population but mainly by the continued use of tobacco. The socioeconomic burden of COPD is also substantial. In 2000, COPD caused 726,000 hospitalizations (which accounted for 1.9% of all hospitalizations in the United States), 7,997,000 office visits to physicians, and 1,549,000 emergency department visits, and, in 2002, COPD resulted in a total health expenditure of $32.1 billion.3 In this regard, COPD is a problem that is a frequent challenge for the respiratory clinician.
Risk Factors and Pathophysiology
Although many risk factors exist for COPD (Box 23-1), the two most common are cigarette smoking (which has been estimated to account for 80% to 90% of all COPD-related deaths) and alpha1-antitrypsin (AAT) deficiency.8 Evidence linking cigarette smoking to the development of COPD is strong and includes the following:
• Symptoms of COPD (e.g., chronic cough and phlegm production) are more common in smokers than in nonsmokers.
• Impaired lung function with evidence of an obstructive pattern of lung dysfunction is more common in smokers than in nonsmokers.
• Pathologic changes of airflow obstruction and chronic bronchitis are evident in the lungs of smokers.
• So-called susceptible smokers, who represent approximately 15% of all cigarette smokers, experience more rapid rates of decline of lung function than nonsmokers.
Information from the Lung Health Study (Figure 23-2) highlighted the accelerated rate of decrease of FEV1 in smokers compared with former smokers who have achieved sustained quitting.9,10 Overall, the strength of evidence implicating cigarette smoking as a cause of COPD has allowed the U.S. Surgeon General to conclude, “Cigarette smoking is the major cause of chronic obstructive lung disease in the United States for both men and women. The contribution of cigarette smoking to chronic obstructive lung disease morbidity and mortality far outweighs all other factors.”11
As the second well-recognized cause of emphysema, AAT deficiency, sometimes called genetic emphysema, is a condition characterized by a deficient amount of the protein AAT, which may result in the early onset of emphysema and which is inherited as a so-called autosomal codominant condition. Accounting for 2% to 3% of all cases of COPD, AAT deficiency is severely underrecognized by health care providers but affects an estimated 100,000 Americans. In one 1995 survey, the mean interval between the first onset of pulmonary symptoms and initial diagnosis of AAT deficiency was 7.2 years, and 43% of individuals with severe deficiency of AAT reported seeing at least three physicians before the diagnosis of AAT deficiency was first made.12 More recent studies suggested that underrecognition of AAT deficiency persisted as of 2003 and that the diagnostic delay interval had not decreased significantly.12–14
The importance of early identification is emphasized by the need to test (by simply sending a serum level for AAT) first-degree relatives (e.g., siblings, parents, and children), by the favorable effect of primary prevention of smoking among individuals identified early, and by the availability of a specific therapy called intravenous augmentation therapy. The risk of developing emphysema for individuals with AAT deficiency increases as the serum AAT level decreases to less than 11 µmol/L, or less than approximately 57 mg/dl using nephelometry, and is enhanced by cigarette smoking.14
Study of AAT deficiency has helped formulate the protease-antiprotease hypothesis of emphysema.14,15 In this explanatory model (Figure 23-3), lung elastin, a major structural protein that supports the alveolar walls of the lung, is normally protected by AAT, a protein that opposes the degradative threat of neutrophil elastase. Neutrophil elastase is a protein contained within neutrophils that is released when neutrophils are attracted to the lung during inflammation or infection. Under normal circumstances of an adequate amount of AAT, neutrophil elastase is counteracted so as not to digest lung elastin. However, in the face of a severe deficiency of AAT (i.e., when serum levels decrease below a “protective threshold” value of 11 µmol/L, or 57 mg/dl), neutrophil elastase may go unchecked, causing breakdown of elastin and resulting in dissolution of alveolar walls. This protease-antiprotease model explains the pathogenesis of emphysema in AAT deficiency, but evidence suggesting its role in COPD in individuals with normal amounts of AAT is conflicting. Also, other proteases (e.g., matrix metalloproteinases and inflammation) are thought to contribute to the proteolysis that produces emphysema.16
COPD may occur in the absence of active cigarette smoking or AAT deficiency (see Box 23-1).17,18 Factors such as passive smoking, air pollution, occupational exposure, and airway hyperresponsiveness may contribute to fixed airflow obstruction.
The mechanisms of airflow obstruction in COPD include inflammation and obstruction of small airways (<2 mm in diameter); loss of elasticity, which keeps small airways open when elastin is destroyed in emphysema; and active bronchospasm. Although traditionally considered to be characteristic of asthma, some reversibility of airflow obstruction has been observed in up to two-thirds of COPD patients when tested serially with inhaled bronchodilators.19
Clinical Signs and Symptoms
Common symptoms of COPD include cough, phlegm production, wheezing, and shortness of breath, typically on exertion. Dyspnea is often slow but progressive in onset and occurs later in the course of the disease, characteristically in the late sixth or seventh decade of life. One notable exception is AAT deficiency, in which dyspnea characteristically begins sooner (mean age approximately 45 years).8
Table 23-1 reviews the characteristic features of emphysema and chronic bronchitis and emphasizes traits that should suggest the possibility of AAT deficiency, including early onset of emphysema, emphysema in a nonsmoker, or a family history of emphysema. Suspicion of AAT deficiency should lead to a simple blood test by which the serum level can be established.8,14
TABLE 23-1
Features | Chronic Bronchitis | Emphysema | Severe Alpha1-Antitrypsin Deficiency |
Symptoms and Signs | |||
Chronic cough, phlegm | Common | Less common | Less common, but may be present |
Dyspnea on exertion | Less common | Common | Common |
Cor pulmonale | Present (often with multiple exacerbations) | Present (but often in end-stage emphysema) | Present (but often in end-stage emphysema) |
Age of patient at symptom onset | 6th-7th decade | 6th-7th decade | 4th-5th decade (although late onset is possible) |
Family history of COPD | Possible but not characteristic | Possible but not characteristic | Common in parents, children, and siblings |
History of cigarette smoking | Present, often heavy | Present, often heavy | May be present, but COPD can occur in the absence of smoking |
Physiologic Function | |||
Airflow (FEV1, FEV1/FVC) | Decreased | Decreased | Decreased |
Lung volumes, residual volume | Normal | Increased, suggesting air trapping | Increased |
Gas exchange, diffusion PaO2 | Often decreased | Often preserved until advanced stage | Often preserved until advanced stage |
PaCO2 | May be increased | Often preserved until advanced disease, then elevated | Often preserved until advanced disease, then elevated |
Diffusion capacity | Often normal | Decreased | Decreased |
Static lung compliance | Normal | Increased | Increased |
Chest radiograph | “Dirty lungs” with peribronchial cuffing, suggesting thickened bronchial walls | Hyperinflation, with evidence of emphysema; greater at lung apex than at lung base | Hyperinflation, with evidence of emphysema; frequently greater at lung base than at lung apex (basilar hyperlucency) |
Management
In managing patients with chronic, stable COPD, the following goals must guide the clinician1,2:
• Establish the diagnosis of COPD.
• Maximize the patient’s functional status.
In managing an acute exacerbation of COPD, additional considerations are to reestablish the patient to baseline status as quickly and with as little incidence of morbidity and mortality as possible.20,21 Each of the treatments discussed in this section is considered in the context of these goals, recognizing differences in management between patients with chronic, stable COPD versus an acute exacerbation of COPD. In patients with COPD, PaCO2 usually is generally preserved until airflow obstruction is severe (FEV1 < 1 L), when the PaCO2 level may increase.
Establishing the Diagnosis
After the diagnosis of COPD is established, a secondary issue is for the clinician to consider whether the patient has an underlying predisposition to COPD, such as AAT deficiency or other cause listed in Box 23-1.17,18 Underlying causes are present in fewer than 5% of patients with COPD, with AAT deficiency being the most common (2% to 3% of all patients with COPD).
Optimizing Lung Function
Stable Chronic Obstructive Pulmonary Disease
Although airflow obstruction from emphysema itself is irreversible, most (up to two-thirds) patients with stable COPD exhibit a reversible component of airflow obstruction, defined as a 12% and 200-ml increase in postbronchodilator FEV1 or FVC or both. For this reason, as indicated in an algorithm developed by GOLD (Figure 23-4),2,22–24 bronchodilator therapy is recommended for patients with COPD.
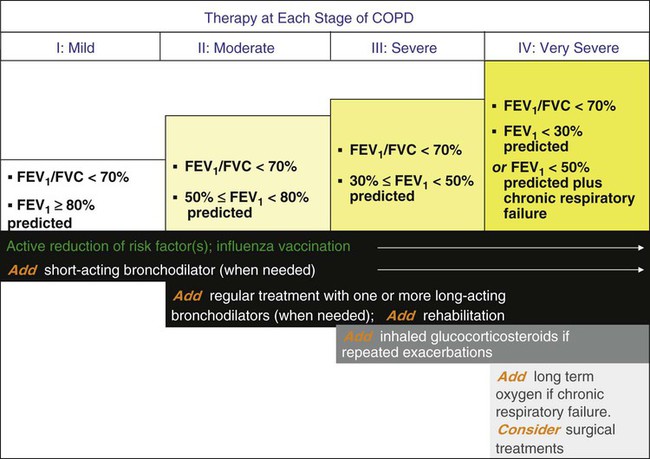
Bronchodilators produce smooth muscle relaxation resulting in improved airflow obstruction, improved symptoms and exercise tolerance, and decrease in the frequency and severity of exacerbations, but they do not enhance survival. The results of the Lung Health Study,9 which compared the effects of inhaled ipratropium bromide (two puffs four times daily) with placebo in patients with mild, stable COPD, showed that regular, long-term use of ipratropium did not change the rate of decline of lung function but offered a one-time, small increase in FEV1.
Both anticholinergic and adrenergic (beta agonist) bronchodilators can improve airflow in patients with COPD, although some clinicians favor an inhaled anticholinergic medication (e.g., ipratropium bromide or tiotropium22–24) as first-line therapy (Figure 23-5). More recent concerns about the possible adverse cardiovascular effects of anticholinergic therapy in patients with COPD25 have been dismissed by the results of a multicenter trial (UPLIFT [Understanding Potential Long-Term Impacts on Function with Tiotropium]), which found a significantly lower rate of cardiac adverse events and cardiovascular death in patients who received tiotropium.26
The GOLD guidelines2 recommend the use of short-acting beta-adrenergic agents (≤6 hours) for symptomatic management of all patients with COPD. Also, the use of a long-acting beta agonist (e.g., salmeterol) or a long-acting anticholinergic drug (e.g., tiotropium) can lessen the frequency of acute exacerbations of COPD.25
Other treatment options to optimize lung function include administering corticosteroids and methylxanthines. Systemic corticosteroids can produce significant improvements in airflow in a few (6% to 29%) patients with stable COPD.27 To assess whether airflow obstruction is completely reversible (i.e., the patient has asthma) and whether a patient with COPD is responsive to steroids, a brief course of corticosteroids (20 to 40 mg/day of prednisone or equivalent for 10 to 14 days) is often recommended. Patients with a significant clinical response often are treated with long-term inhaled corticosteroids or rarely with the smallest necessary dose of systemic corticosteroids, recognizing that long-term systemic steroid therapy has risks.28 Also, results of several major clinical trials (e.g., Lung Health Study II, Euroscop, ISOLDE, and Copenhagen City Study but not TORCH) agree that inhaled corticosteroids do not change the rate of decline of FEV1 in patients with COPD, although their use is associated with a decreased frequency of acute exacerbations.29,30
Studies of combined salmeterol and fluticasone versus placebo in patients with COPD suggest that adding an inhaled corticosteroid (fluticasone) to the long-acting beta agonist (salmeterol) can improve FEV1 and can reduce the frequency of acute exacerbations of COPD but does not improve survival.29,30 The finding of a higher rate of pneumonia in inhaled corticosteroid users is concerning. Overall, the GOLD guidelines2 recommend use of inhaled corticosteroids in patients with FEV1 less than 50% and history of recurrent exacerbations (three episodes in the last 3 years), whereas the ATS/European Respiratory Society (ERS) guidelines recommend use of inhaled corticosteroids in patients with FEV1 less than 50% who have required use of oral corticosteroids or oral antibiotics at least once within the last year.31
Treatment with methylxanthines offers little additional bronchodilation in patients using inhaled bronchodilators and generally is reserved for patients with debilitating symptoms from stable COPD despite optimal inhaled bronchodilator therapy. Controlled trials show lessened dyspnea in methylxanthine recipients despite a lack of measurable increases in airflow.32 Side effects of methylxanthines include anxiety, tremulousness, nausea, cardiac arrhythmias, and seizures. To minimize the chance of toxicity, current recommendations suggest maintaining serum theophylline levels at 8 to 10 mcg/ml.
Acute Exacerbations
Strategies for improving lung function during acute exacerbations of COPD generally include inhaled bronchodilators (especially beta-2 agonists), antibiotics, and systemic corticosteroids. Because of their rapid onset of action and efficacy, short-acting beta-2 agonists are first-line therapy for patients with COPD exacerbation. Inhaled beta-2 agonists are frequently administered through a nebulizer, although metered dose inhaler devices may have equal efficacy if administered appropriately.2 A common practice is to administer 2.5 mg of albuterol by nebulizer every 1 to 4 hours as needed. Higher doses of albuterol (i.e., 5 mg) do not produce further improvement in pulmonary function and may cause cardiac side effects.33 Similarly, continuous nebulization of short-acting beta-2 agonists in patients with COPD exacerbation is not recommended.
In addition to inhaled bronchodilator therapy, short-term systemic corticosteroids are recommended to reduce inflammation and improve lung function. An early randomized, controlled trial of intravenous methylprednisolone for patients with acute exacerbations showed accelerated improvement in FEV1 within 72 hours.34 Larger, more recent trials have confirmed the benefits of systemic corticosteroids in acute exacerbations and have shown that short-term oral courses (i.e., approximately 2 weeks) are as effective as longer courses (i.e., 8 weeks) with fewer adverse steroid effects.35 For patients with acute exacerbations characterized by purulent phlegm, oral antibiotics (e.g., trimethoprim-sulfamethoxazole, amoxicillin, or doxycycline) administered for 7 to 10 days have produced accelerated improvement of peak flow rates compared with placebo recipients.20,36,37
Finally, intravenous methylxanthines offer little benefit in the setting of acute exacerbations of COPD and have fallen into disfavor.38,39 Taken together, important elements of managing an acute exacerbation of COPD caused by purulent bronchitis include supplemental oxygen (O2) to maintain arterial saturation at greater than 90%, inhaled bronchodilators, oral antibiotics, and a brief course of systemic corticosteroids.20
For patients with hypercapnia and acute respiratory acidemia, the clinician also must decide whether to institute ventilatory assistance. Although intubation and mechanical ventilation historically have been the preferred approach, more recent studies suggest that noninvasive positive pressure ventilation can be an appealing alternative for patients with acute exacerbations of COPD, especially with severe exacerbations characterized by pH less than 7.30.40 Specifically, based on available randomized, controlled clinical trials showing that noninvasive positive pressure ventilation can shorten intensive care unit (ICU) stay and avert the need for intubation, the American Association for Respiratory Care consensus conference and guidelines on noninvasive ventilation from other official societies have endorsed this approach.41,42 Criteria defining candidacy for noninvasive ventilation include acute respiratory acidosis (without frank respiratory arrest); hemodynamic stability; ability to tolerate the interface needed for noninvasive ventilation; ability to protect the airway; and lack of craniofacial trauma or burns, copious secretions, or massive obesity.41
Maximizing Functional Status
In symptomatic patients with stable COPD, maximizing their ability to perform daily activities is a priority (see Figure 23-4). Pharmacologic treatments to maximize functional status include administration of bronchodilators to enhance lung function as much as possible and consideration of methylxanthine therapy, based on data that such drugs can lessen dyspnea and improve functional status ratings even in the absence of improved airflow.32
Comprehensive pulmonary rehabilitation is a multidisciplinary intervention that consists of lower and upper extremity exercise conditioning, breathing retraining, education, and psychosocial support. Pulmonary rehabilitation is an additional important strategy for improving functional status.43 Randomized, controlled trials show that although pulmonary rehabilitation does not improve lung function or survival, this strategy results in decreased dyspnea perception, improved health-related quality of life, fewer days of hospitalization, and decreased health care usage.44,45
Finally, transcutaneous neuromuscular electrical stimulation is a new experimental therapy that has been successfully used to stimulate peripheral muscles in patients with COPD. Studies have shown significant improvements in quadriceps muscle function, exercise tolerance (including walk distance), and health status in patients with severe COPD.46
Preventing Progression of Chronic Obstructive Pulmonary Disease and Enhancing Survival
Follow-up data from the Lung Health Study9 confirm that a comprehensive smoking cessation program (including instruction, group counseling, and nicotine replacement therapy) can achieve sustained smoking cessation in 22% of participants and that the rate of annual FEV1 decline in these sustained nonsmokers was significantly less than it was for continuing smokers, even over 11 years of follow-up.10 Participation in aggressive smoking cessation can enhance survival rates in patients with COPD.10
Critical elements in achieving successful abstinence from smoking include identifying “teachable moments” (i.e., during episodes of illness where smoking can be identified as a contributing factor47), identifying the role of smoking in adverse health outcomes, negotiating a “quit date,” and providing frequent follow-up reminders from health care providers.48 A helpful strategy during counseling is to use the five A’s of smoking cessation2:
In this regard, the respiratory therapist (RT), who sees the patient frequently, has a special responsibility to provide frequent, constructive reminders about the advisability of smoking cessation.49
Among available treatments for COPD, supplemental oxygen is important because, similar to smoking cessation and lung volume reduction surgery in selected individuals (see later), it can prolong survival.50–53 Box 23-2 reviews the indications for supplemental O2, and Figure 23-5 shows the results of the American Nocturnal Oxygen Therapy Trial50 and the British Medical Research Council trial of domiciliary O2 (1980-1981).51,52 Survival was improved when eligible patients used supplemental O2 for as close to 24 hours as possible; survival improved less for patients using O2 only 15 hours per day. No survival advantage was observed when O2 use was confined to the sleeping hours. Patients should be assessed for supplemental O2 use only after receiving optimal bronchodilator therapy because one-third of potential O2 candidates can experience sufficient improvement with aggressive bronchodilation to avoid the need for long-term supplemental O2. Also, patients prescribed to receive supplemental O2 during acute exacerbations should be reassessed several months later to determine whether their candidacy and need for supplemental O2 continue.54 RTs can play a key role in ensuring compliance with this recommendation and optimizing O2 therapy.54,55
Finally, preventive strategies such as annual influenza and pneumococcal vaccinations are recommended for all patients with chronic debilitating conditions such as COPD.56 Specific indications for pneumococcal vaccination are presented in Box 23-3.
Additional Therapies
Additional therapies for individuals with end-stage COPD include lung transplantation57 and lung volume reduction surgery (LVRS),58–60 in which small portions of emphysematous lung are removed to reduce hyperinflation and improve lung mechanics of the remaining tissue. COPD is the most common current indication for lung transplantation. Lung transplantation is a consideration for patients with severe airflow obstruction (i.e., FEV1 < 20% predicted) who are younger than 65 years old, who lack major dysfunction of other organs, and who are psychologically and motivationally suitable. Given the scarcity of available lungs to transplant, single-lung transplantation usually is performed for individuals with COPD. Although lung transplantation may be associated with significantly improved quality of life and functional status, major risks include rejection (manifested as bronchiolitis obliterans and progressive, debilitating airflow obstruction), infection with unusual opportunistic organisms, and death from these and other complications. The 5-year actuarial survival rate after single-lung transplantation is approximately 54% (Figure 23-6).
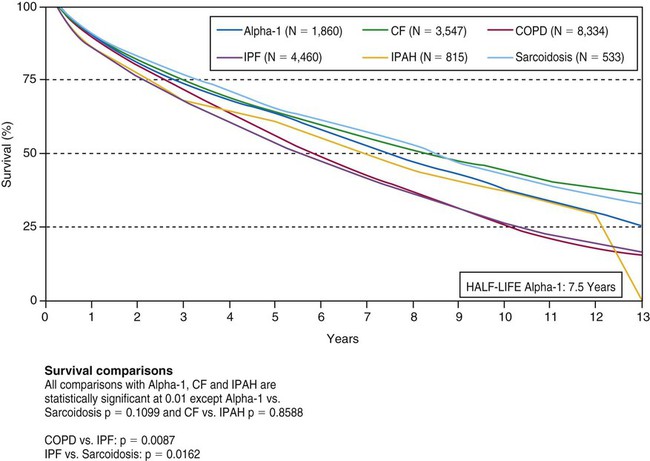
LVRS has regained popularity after initial experiences were reported in 1957.58 Results of randomized controlled trials of LVRS, including the large National Emphysema Treatment Trial, indicate that in selected subsets of patients with COPD (i.e., patients with heterogeneous emphysema that is upper lobe predominant and who have low exercise capacity after pulmonary rehabilitation), LVRS can prolong survival, improve quality of life, and increase exercise capacity.59,60 LVRS should not be considered in individuals with very severe COPD (i.e., characterized by FEV1 < 20% predicted with either a homogeneous pattern of emphysema or a diffusing capacity <20% predicted) because the mortality rate of LVRS is higher in such individuals than in medically treated patients.61
Given the positive results associated with LVRS in selected patients with COPD, nonsurgical bronchoscopic techniques have been developed in an attempt to reduce costs and expand treatment options for patients with high operative risk.62 With the use of the bronchoscope, deployment of unidirectional endobronchial valves into the airways results in collapse of the targeted lung parenchyma. Other techniques include application of biodegradable gel to induce lung collapse or application of bronchial stents to create fenestrations and allow gas escape from hyperinflated areas of the lung. None of the aforementioned devices is currently approved by the U.S. Food and Drug Administration (FDA) for treatment of emphysema in the United States.63
Finally, for patients with AAT deficiency and established COPD, so-called intravenous augmentation with a purified preparation of AAT from human blood donors is recommended.14 Although no definitive randomized, controlled trials are available,64,65 observational studies suggest that for individuals with severe AAT deficiency and moderate degrees of airflow obstruction (i.e., FEV1 35% to 60% predicted), weekly augmentation therapy may be associated with a slower rate of decline of lung function and improved survival. Difficulties with intravenous augmentation therapy include the substantial expense (approximately $100,000 per year); the inconvenience of frequent intravenous infusions for life; and the infusion itself, which confers a theoretical risk of transmitting a blood-borne infection. Despite these drawbacks, the facts that augmentation therapy can slow the rate of FEV1 decline, can possibly slow the rate of computed tomography (CT) density loss, and is currently the only specific therapy for AAT deficiency have led to its endorsement in official guidelines from the ATS, the ERS, and the Canadian Thoracic Society.14,48,66
Asthma
Definition
Asthma is a clinical syndrome characterized by airway obstruction, which is partially or completely reversible either spontaneously or with treatment; airway inflammation; and airway hyperresponsiveness (AHR) to various stimuli.67–69 Past definitions of asthma emphasized AHR and reversible obstruction; however, newer and more accurate definitions of asthma focus on asthma as a primary inflammatory disease of the airways, with clinical manifestations of increased airway hyperreactivity and airflow obstruction caused by the inflammation.
Incidence
Asthma is a chronic illness that has been increasing in prevalence in the United States since 1980. In 2007, asthma accounted for 3447 deaths, approximately 456,000 hospitalizations, and approximately 13.3 million emergency department or physician office visits among persons of all ages. Approximately 7.7% of U.S. adults, or 17.5 million Americans, have asthma currently.67–70
Etiology and Pathogenesis
In the genetically susceptible host, allergens, respiratory infections, certain occupational and environmental exposures, and many unknown hosts or environmental stimuli can produce the full spectrum of asthma, with persistent airway inflammation, bronchial hyperreactivity, and subsequent airflow obstruction. When inflammation and bronchial hyperreactivity are present, asthma can be triggered by additional factors, including exercise; inhalation of cold, dry air; hyperventilation; cigarette smoke; physical or emotional stress; inhalation of irritants; and pharmacologic agents, such as methacholine and histamine.71–73
When a patient with asthma inhales an allergen to which he or she is sensitized, the antigen cross-links to specific IgE molecules attached to the surface of mast cells in the bronchial mucosa and submucosa. The mast cells degranulate rapidly (within 30 minutes), releasing multiple mediators including leukotrienes (previously known as slow-reacting substance of anaphylaxis [SRS-A]), histamine, prostaglandins, platelet-activating factor, and other mediators. These mediators lead to smooth muscle contraction, vascular congestion, and leakage resulting in airflow obstruction, which can be assessed clinically as a decline in FEV1 or peak expiratory flow rate (PEFR) (Figure 23-7). This is the early (acute) asthmatic response, which is an immediate hypersensitivity reaction that usually subsides in about 30 to 60 minutes. In approximately 50% of asthmatic patients, however, airflow obstruction recurs in 3 to 8 hours.73 This late asthmatic response is usually more severe and lasts longer than the early asthmatic response (Figure 23-8).74 The late asthmatic response is characterized by increasing influx and activation of inflammatory cells such as mast cells, eosinophils, and lymphocytes.74,75
Clinical Presentation and Diagnosis
Confirmation of the diagnosis of asthma requires demonstration of reversible airflow obstruction. Pulmonary function tests may be normal in asymptomatic patients with asthma, but more commonly they reveal some degree of airway obstruction manifested by decreased FEV1 and FEV1/FVC ratio. By convention, improvement in the FEV1 by at least 12% and 200 ml after administration of a bronchodilator is considered evidence of reversibility. Spontaneous variation in self-recorded PEFR by 15% or more also can provide evidence of reversibility of airway obstruction (see Figure 23-7).
The most commonly used stimulus for bronchoprovocation is methacholine. The generally accepted criterion for hyperresponsiveness is a decrease in FEV1 by 20% or more below the baseline value after inhalation of methacholine (see Figure 23-7).
The methacholine provocation test has few false-negative results (<5%), but a false-positive result may be found in 7% to 8% of the average population and patients with other obstructive lung diseases. Elevated IgE levels and eosinophilia may be present in patients with asthma, but their presence is not specific, and their absence does not exclude asthma, rendering them not useful for the diagnosis.67–69 Although arterial blood gas analysis is not helpful or necessary in diagnosing asthma, it can be helpful in assessing the severity of an acute asthma attack.
Management
The goal of asthma management is to maintain a high quality of life for the patient, uninterrupted by asthma symptoms, side effects from medications, or limitations on the job or during exercise. This goal can be accomplished by preventing acute exacerbations, with their potential mortality and morbidity, or by returning the patient to a stable baseline when exacerbations occur. Asthma management relies on the following four integral components recommended by the National Asthma Education Program (NAEP) expert panel67:
Table 23-2 outlines the stepwise approach currently recommended for long-term management of asthma. This approach provides a framework for an individually tailored dose of medication based on the severity of asthma in any patient at a particular time. This approach acknowledges that asthma is a chronic and dynamic disease, which needs optimum control. Control of asthma is defined as minimal to no chronic diurnal or nocturnal symptoms, infrequent exacerbations, minimal to no need for beta-2 agonists, no limitation to exercise activity, PEFR or FEV1 greater than 80% predicted with less than 20% diurnal variation, and minimal to no adverse effects of medication.67–69
TABLE 23-2
Stepwise Approach to Long-Term Management of Asthma Based on Severity
Severity* | Clinical Features Before Treatment† | PEFR or FEV1 | Long-Term Preventive Medications | Quick-Relief Medications |
Step 4 | ||||
Severe persistent | Continuous symptoms | ≤60% predicted | Inhaled corticosteroids ≥800-2000 mcg/day | Inhaled beta-2 agonist as needed for symptoms |
Red zone | Frequent exacerbations | >30% variability | Long-acting bronchodilator‡ | |
Nocturnal symptoms | Oral corticosteroids | |||
Symptoms limit activity | ||||
Step 3 | ||||
Moderate persistent | Daily symptoms | >60%-<80% predicted | Inhaled corticosteroids ≥800-2000 mcg/day | Inhaled beta-2 agonist as needed for symptoms, not to exceed 3-4 times per day |
Yellow zone | Exacerbations affect activity and sleep | >30% variability | Long-acting bronchodilator,‡ especially for nocturnal symptoms | |
Nocturnal symptoms more than once per week | ||||
Daily use of short-acting beta-2 agonist | ||||
Step 2 | ||||
Mild persistent | Symptoms at least once per week but <1 time per day | ≥80% predicted | Inhaled corticosteroid, 200-500 mg/day, cromolyn, or nedocromil | Inhaled beta-2 agonist as needed for symptoms, not to exceed 3-4 times per day |
Yellow zone | Exacerbations may affect activity or sleep | 20%-30% variability | Long-acting bronchodilator‡ for nocturnal symptoms | |
Nocturnal symptoms more than twice per month | ||||
Step 1 | ||||
Intermittent | Intermittent symptoms less than once per week | ≥80% predicted | None needed | Inhaled beta-2 agonist needed for symptoms but less than once per week |
Green zone | Nocturnal symptoms not more than twice per month | <20% variability | Inhaled beta-2 agonist or cromolyn before exercise or exposure to allergen | |
Asymptomatic with normal lung function between exacerbations |
*Step-down: Review treatment every 3-6 months. If control is sustained for at least 3 months, consider a gradual stepwise reduction in treatment. Step-up: If control is not achieved, consider step-up, but first review patient medication technique, compliance, and environmental control.
†The presence of one of the features of severity is sufficient to place a patient in that category.
‡Long-acting beta-2 agonist or sustained-release theophylline.
Modified from Global Initiative for Asthma: Asthma management and prevention: a practical guide for public health officials and health care professionals, NIH publication no. 96-3659A, Bethesda, MD, 1995, National Institutes of Health, National Heart, Lung, and Blood Institute, and World Health Organization.
Objective Measurement and Monitoring
When patients learn how to take PEFR measurements at home, the clinician is better able to recommend effective treatment. Daily monitoring of PEFR helps detect early stages of airway obstruction. All PEFR measurements are compared with the patient’s personal best value, which can be established during a 2- to 3-week asymptomatic period when the patient is being treated optimally.67–69
To help patients understand home PEFR monitoring, a zonal system corresponding to the traffic light system may be helpful (see Table 23-2). A PEFR measurement of 80% to 100% of the personal best is considered to be in the green zone. No asthma symptoms are present, and maintenance medications can be continued or tapered. A PEFR in the 60% to 80% range of the personal best is in the yellow zone and may indicate an acute exacerbation and requires a temporary step-up in treatment. A PEFR less than 60% of the personal best is in the red zone and signals a medical alert, requiring immediate medical attention if the patient does not return to the yellow zone or green zone with bronchodilator use.69
Pharmacotherapy
Pharmacotherapy for asthma reflects the basic understanding that asthma is a chronic inflammatory airway disease that requires long-term antiinflammatory therapy for adequate control.67–76 Antiinflammatory agents, such as corticosteroids and cromolyn, suppress the primary disease process and its resultant airway hyperreactivity. Bronchodilators, such as beta-2-adrenergic agonists, anticholinergics, and theophylline, relieve asthma symptoms. Because asthma is a disease of the airways, inhalation therapy is preferred to oral or other systemic therapy. Inhaled therapy using metered dose inhalers or powders allows high concentration of the medication to be delivered directly to the airways, resulting in fewer systemic side effects. Spacer devices can be used to improve delivery of inhaled medication, but training and coordination are still required for patients using metered dose inhalers. Table 23-3 lists commonly used medications in the treatment of asthma.
TABLE 23-3
Medications Commonly Used in the Treatment of Asthma and COPD
Medication | Trade Names | Available Preparations | Usual Dosage |
Inhaled Corticosteroids | |||
Beclomethasone | Beclovent, Vanceril | MDI 42 mcg/puff, 200 puffs/canister | 2 puffs tid-qid, maximum 20 puffs/day |
Triamcinolone | Azmacort | MDI 100 mcg/puff, 240 puffs/canister | 2-4 puffs qid, maximum 16 puffs/day |
Flunisolide | AeroBid | MDI 250 mg/puff, 100 puffs/canister | 2 puffs bid, maximum 8 puffs/day |
Fluticasone | Flovent | MDI 44, 110, 220 mcg/puff | 88-880 mcg/day |
Mometasone | Asmanex | DPI 220 mcg/spray | 1-2 sprays qd-bid, maximum 4 puffs/day |
Budesonide | Pulmicort | DPI 90, 180 mcg/puff | 360-720 mcg/day |
Systemic Corticosteroids | |||
Prednisone | Many | Tablets 1, 5, 20, 50 mg | 5-50 mg/day |
Methylprednisolone | Medrol | Tablets 2, 4, 8, 16, 24, 32 mg | 4-48 mg/day |
Solu-Medrol | IV 40, 125, 500, 1000 mg | 1-2 mg/kg q4-6h | |
Hydrocortisone | Solu-Cortef | IV 100, 250, 500, 1000 mg | 4 mg/kg q4-6h |
Beta-2 Agonists | |||
Albuterol | Proventil | MDI 90 mcg/puff, 200 puffs/canister | 2-4 puffs q4-6h, maximum 20 puffs/day |
Ventolin | Solution for nebulizer 0.083% and 0.5% | 2.5-10 mg q6-8h | |
Tablets 2, 4 mg | 2-4 mg q6-8h | ||
Volmax | Sustained-release tablets 4, 8 mg | 4-8 mg q12h | |
Metaproterenol | Alupent | MDI 650 mcg/puff, 200 puffs/canister | 2-3 puffs q3-4h, maximum 12 puffs/day |
Metaprel | Solution 0.5% | 2.5-10 mg q4-6h | |
Tablets 10, 20 mg | 10 mg q6-8h | ||
Pirbuterol | Maxair | MDI 200 mcg/puff, 300 puffs/canister | 1-2 puffs q4-6h, maximum 12 puffs/day |
Terbutaline | Breathaire | MDI 200 mcg/puff, 300 puffs/canister 1-2 puffs q4-6h | |
Bricanyl | Tablets 2.5, 5 mg | 2.5-5 mg tid, maximum 15 mg/day | |
Solution 1 mg/ml | 0.25 mg SC q15-30min | ||
Salmeterol | Serevent | MDI 50 mcg/puff | 2 puffs q12h |
Formoterol | Foradil | DPI 12 mcg/capsule | 1 capsule inhaled q12h |
Arformoterol | Brovana | Solution 15 mcg/ml | 15 mcg inhaled bid |
Anticholinergics | |||
Ipratropium bromide | Atrovent | MDI 18 mcg/puff, 200 puffs/canister | 2-4 puffs q6h |
Solution for nebulizer 0.02% | |||
0.5 mg/2.5 ml vial, 0.5 mg qid | |||
Tiotropium | Spiriva | DPI 18 mcg/capsule | 1 capsule inhaled/day |
Methylxanthines | |||
Aminophylline | IV | Load 5-6 mg/kg, maintenance | |
Tablets or capsules | 0.5-0.9 mg/kg/hr | ||
Theophylline | Theo-Dur | 300-1200 mg/day divided q6-8h | |
Slo-bid | for immediate and q12-24h | ||
Theovent | for sustained | ||
Uniphyl | |||
(immediate or sustained release) | |||
Leukotriene Inhibitors | |||
Zafirlukast | Accolate | Tablets 20 mg | 20 mg bid |
Zileuton | Zyflo | Tablets 600 mg | 600 mg qid |
Montelukast | Singulair | Tablets 10 mg | 10 mg qd |
Other | |||
Cromolyn | Intal | MDI 800 mcg/puff, 112 puffs/canister | 2 puffs qid |
Spinhaler 20 mg/capsule | 20 mg qid | ||
Nedocromil | Tilade | MDI 1.75 mg/puff, 112 puffs/canister | 2 puffs bid-qid |
DPI, Dry powder inhaler; IV, intravenous; MDI, metered dose inhaler; SC, subcutaneous.
Corticosteroids
Corticosteroids are the most effective medication currently available for the treatment of asthma. Although their mode of action is still uncertain, corticosteroids probably act on various components of the inflammatory response in asthma.76 Inhaled corticosteroids are effective locally, and regular use suppresses inflammation in the airways, decreases bronchial hyperreactivity and airflow obstruction, and reduces the symptoms of and mortality from asthma. Long-term, high-dose inhaled corticosteroids have far fewer side effects than oral corticosteroids. Side effects such as oropharyngeal candidiasis and dysphonia are controllable with spacer use and by rinsing the mouth after each treatment.
Oral corticosteroids are effective for treating asthma, but the potential for devastating side effects during long-term use restricts their use to patients not responding to other forms of asthma therapy. Short-term, high-dose (0.5 to 1.0 mg/kg/day) oral corticosteroid therapy during exacerbation reduces the severity and duration, decreases the need for emergency department visits and hospitalization, and reduces mortality.76
Cromolyn
Cromolyn sodium is a noncorticosteroid antiinflammatory medication. Its mechanism of action is not completely understood, but it has a protective effect against provocative stimuli such as allergens, cold air, and exercise. The drug is most effective when administered prophylactically. Cromolyn does not dilate smooth muscle and is not useful for treating the symptoms of an acute asthma attack. Cromolyn is useful in cough-variant and exercise-induced asthma but otherwise has only limited usefulness in the treatment of adult asthmatics. However, many experts believe that cromolyn is the drug of choice for atopic children with asthma.72
Nedocromil
In 1993, nedocromil was approved in the United States for use in metered dose inhalers. It is structurally different from cromolyn but has similar pharmacologic activity and is 4 to 10 times more potent in preventing acute allergic bronchospasm. Nedocromil can be used as an alternative to cromolyn, but neither nedocromil nor cromolyn has a clear advantage over inhaled corticosteroids.72
Leukotriene Inhibitors
Leukotrienes are mediators of inflammation and bronchoconstriction and are thought to play a role in the pathogenesis of asthma. Three leukotriene antagonists are currently available for the treatment of asthma. Montelukast (Singulair; Merck, Whitehouse Station, NJ) and zafirlukast (Accolate; Astra Zeneca, London, United Kingdom) are leukotriene receptor antagonists, and zileuton (Zyflo; Abbott Laboratories, Chicago, IL) is a leukotriene synthesis inhibitor. These agents all are modestly effective for maintenance of mild to moderate asthma, but their exact role in asthma therapy remains to be determined. Inhaled steroids remain the antiinflammatory drugs of choice for the treatment of asthma.77
Beta-2-Adrenergic Agonists
Although there is sufficient concern regarding fenoterol to justify avoiding its use, it remains unclear whether the association between excessive beta-2 agonist use and death from asthma is a genuine or spurious association.77 It is clear, however, that excessive beta-2 agonist use by asthmatics indicates an increased risk of death from asthma and indicates the need for more effective antiinflammatory therapy. There is currently little definitive evidence to establish that treatment with conventional doses of the short-acting beta-2 agonists available in the United States is harmful to asthmatic patients.75,76,78 The NAEP guidelines recommend that inhaled beta-2 agonists be used as needed. If a patient needs more than 3 or 4 puffs a day of a beta-2 agonist, additional antiinflammatory therapy should be considered.77
Newer, longer acting (12 to 24 hours) beta-2 agonists, such as salmeterol and formoterol, are available in the United States. Their mechanism of action is different from the shorter acting beta-2 agonists discussed earlier. Long-acting beta-2 agonists have use in treating nocturnal asthma.67–69,78
Monotherapy with beta agonists has been shown to be inferior to use of inhaled corticosteroids, which are recommended as first-line treatment for asthma. When added to inhaled corticosteroids, long-acting beta agonists have been found to improve asthma control, symptoms, and exacerbations. However, a meta-analysis of randomized controlled clinical trials examining outcomes for asthma control found that long-acting beta agonists increase the risk for hospitalization from asthma, life-threatening asthma attacks, and asthma-related deaths compared with placebo.79 Several mechanisms need to be considered to explain such reactions, including paradoxical bronchospasm, increased bronchial responsiveness, and tolerance. Racial and genetic factors may also influence these outcomes.
The FDA requested manufacturers of Serevent Diskus (salmeterol xinafoate inhalation powder), Advair Diskus (fluticasone propionate and salmeterol inhalation powder), and Foradil Aerolizer (formoterol fumarate inhalation powder) to update their existing product labels with new warnings and a medication guide for patients to alert health care professionals and patients that these medicines may increase the chance of severe asthma episodes and death when such episodes occur.80 Inhaled corticosteroids remain the first choice of therapy in asthma, and careful monitoring is required when long-acting beta agonists are used for asthma control.
Methylxanthines
Several factors affect the plasma levels of theophylline by increasing or decreasing hepatic metabolism of the drug. Conditions that tend to increase plasma concentrations include acute viral infections, cardiac failure, hepatic disease, and concomitant use of certain medications such as erythromycin or cimetidine. In these cases, the maintenance dose should be halved, and the theophylline blood levels should be monitored. Conditions that tend to decrease plasma levels of theophylline include cigarette smoking and use of medications that increase hepatic clearance, such as phenobarbital.67–69,72
Anticholinergics
Inhaled anticholinergic agents, such as ipratropium bromide, are effective dilators of airway smooth muscles. Although the regular use of these agents seems to be effective in patients with COPD, their benefit in the day-to-day management of asthma has not been established. Ipratropium produces bronchodilation by reduction of intrinsic vagal tone and by blocking vagal reflex bronchospasm. However, ipratropium does not stabilize mast cells or prevent mediator release and is a less potent bronchodilator than beta-2 agonists. Ipratropium has few side effects, is safe because it is poorly absorbed, adds a bronchodilator effect to beta-2 agonists, and is useful for treating cough-variant asthma. Ipratropium also can be used in treating acute asthma when first-line bronchodilators are ineffective. The long-acting anticholinergic agent, tiotropium, has been shown to enhance asthma control (e.g., improved peak expiratory flow, increased FEV1, and improved symptoms) when added to an inhaled corticosteroid compared with doubling the inhaled steroid dose.81
Anti-IgE Therapy
IgE plays a key role in the pathogenesis of asthma, and many asthmatic patients have elevated levels of IgE.82 Corticosteroids do not inhibit synthesis of IgE by activated lymphocytes. Omalizumab, an antibody that binds IgE and blocks its biologic effects, has been approved by the FDA for patients with a history of allergy and with moderate to severe asthma that is poorly controlled with inhaled corticosteroids.83 Studies have shown that treatment with omalizumab results in a reduction in the dose of inhaled glucocorticoids required to control symptoms and a reduction in the number of asthma exacerbation episodes.84 For this reason, the NAEP asthma guidelines recommended that omalizumab should be considered as adjunctive therapy for patients with severe persistent asthma.67
Emergency Department and Hospital Management
Emergency management of acute asthma should include early and frequent administration of aerosolized beta-2 agonists and therapy with systemic corticosteroids. Frequent assessment for response with PEFR should be performed. The NAEP guidelines recommend that only selective beta-2 agonists (i.e., albuterol, levalbuterol, pirbuterol) should be used in high doses to avoid cardiotoxicity.67 It is unclear which subset of patients may benefit from continuous aerosolized beta-2 agonists.
Patients with severe asthma and respiratory failure (hypoxemia, hypercapnia, increased work of breathing) need ventilatory support and present special challenges. Mortality rates for these patients can reach 22%, and complications are common, especially barotrauma. These complications can be minimized by limiting peak inspiratory pressure to less than 50 cm H2O and by the use of small tidal volumes, allowing “permissive hypercapnia” if necessary. When asthma control is achieved, hospital discharge criteria include being off O2, with PaO2 greater than 60 mm Hg; stable PEFR or FEV1, with values close to the patient’s best or greater than 70% of predicted; asthma symptoms returning to preadmission levels; no nocturnal symptoms; and 12- to 24-hour stability on discharge medications.67–69
Bronchial Thermoplasty
Bronchial thermoplasty is a recently approved addition to treatment options for adults whose asthma remains uncontrolled despite use of inhaled steroids and long-acting beta agonists.85 Bronchial thermoplasty is a procedure in which a probe is introduced into the central airways through a bronchoscope and heat is applied (through radiofrequency waves) to airways of 3 to 10 mm diameter with the goal to reduce the airway smooth muscle mass, reducing the ability of the airways to constrict. Studies have shown that bronchial thermoplasty improves asthma-specific quality of life and reduces the number of severe asthma exacerbation episodes and emergency department visits.86,87 Although bronchial thermoplasty is a promising new treatment for patients with difficult to control asthma, the long-term side effects of this procedure have not been well studied, and more studies are needed before it can be recommended to all patients with poorly controlled asthma.
Immunotherapy
Immunotherapy is based on the theoretical rationale that part of the immunologic response to an administered allergen is the production of IgG-specific antibody to the allergen injected. This newly generated IgG does not affix to most cells but can react with the allergen diffusing into the tissues and “neutralize” it. Although immunotherapy is acceptable in the treatment of allergic rhinitis, its use in the treatment of asthma is not standardized and remains controversial. However, a meta-analysis of 88 randomized controlled trials of injection allergen immunotherapy for asthma reported that immunotherapy is effective, with evidence of significant reductions in asthma medications and symptoms and a reduction in the degree of bronchial hyperreactivity.88
Environmental Control
Additional helpful control measures include reducing the indoor humidity to less than 50%, removing carpets from the bedroom, and using chemical agents to kill mites. Indoor air-cleaning devices, especially high-efficiency particulate air/aerosol filters, may be useful, but they cannot substitute for controlling the allergen source. Humidifiers are potentially harmful because they can harbor and aerosolize mold spores, and the increased humidity they generate may encourage production of both mold and house-dust mites.67–69
Patient Education
Much of the day-to-day responsibility for managing asthma falls on the patient and the patient’s family. Patient education is a powerful motivational tool, helping patients attain the skills and gain the confidence to control their asthma. Patient education involves helping patients understand asthma and learning and practicing the skills necessary to manage it. Patient education includes providing information; developing a partnership with the patient; involving the patient in decision making; and demonstrating and observing asthma management practices such as the proper use of inhalers, nebulizers, and peak flowmeters.78
Special Considerations in Asthma Management
Exercise-Induced Asthma
Exercise-induced asthma is common in asthmatics, especially after participation in outdoor activities in cold weather. The causes are not fully understood, but heat loss from the airways seems to be one of the causes.74 Treatment consists of prophylactic inhalation of a beta-2 agonist or cromolyn before exercise. Leukotriene inhibitors also may have a role in treatment of exercise-induced asthma.67,68
Occupational Asthma
An estimated 2% to 5% of all asthma episodes may be caused by exposure to a specific sensitizing agent in the workplace. Occupational asthma is the most common form of occupational lung disease in many industrialized countries. In an attempt to distinguish occupational from preexisting asthma, occupational asthma is defined as a disease characterized by a variable airflow limitation or AHR secondary to causes and conditions attributable to a particular working environment and not to stimuli encountered outside the workplace. Toluene diisocyanate is the most common cause of occupational asthma and is the best studied. Other causes of occupational asthma are listed in Table 23-4.
TABLE 23-4
Occupation or Industry | Agent |
Laboratory animal workers, veterinarians | Animals (dander, urine protein) |
Food processing | Shellfish, egg proteins, pancreatic enzymes |
Dairy farming | Storage mites |
Poultry farming | Poultry mites, droppings, feathers |
Detergent manufacturing | Bacillus subtilis enzymes |
Baking | Flour |
Sawmill workers, carpentry | Wood dust (western red cedar, oak, mahogany, zebrawood, redwood) |
Nursing | Psyllium |
Refining | Platinum salts |
Plating | Nickel salts |
Stainless steel welding | Chromium salts |
Cosmetology | Persulfate |
Refinery workers | Vanadium |
Rubber processing | Formaldehyde, ethylenediamine |
Plastics industry | Toluene diisocyanate, trimellitic anhydride |
Generally, the treatment of occupational asthma is identical to treatment of other types of asthma. However, early diagnosis is important, and emphasis is placed on environmental control, in particular, cessation of exposure. Complete cessation of exposure is usually necessary because once sensitization has occurred, bronchoconstriction can be triggered by minimal subsequent exposure.68,75
Nocturnal Asthma
After ensuring adequate antiinflammatory therapy, medications for nocturnal asthma should be focused toward the night and especially the early morning hours, when the airway tone is lowest. Sustained-release theophylline and newer long-acting beta-2 agonists such as salmeterol are particularly helpful for controlling nocturnal asthma symptoms.68,75 Addition of a proton pump inhibitor such as esomeprazole has not been shown to enhance asthma control.89
Aspirin Sensitivity
At least 5% of adults with asthma experience severe and even fatal exacerbation of asthma after taking aspirin or other nonsteroidal antiinflammatory drugs (NSAIDs). Many of these patients have nasal polyps, although the relationship is not causal. The presumed mechanism is the inhibition of the cyclooxygenase pathway by aspirin and NSAIDs, with subsequent shunting of all arachidonic acid into the 5-lipoxygenase pathway, causing overproduction of bronchoconstrictor leukotrienes. Individuals with asthma should avoid aspirin and NSAIDs and instead use alternatives such as acetaminophen (e.g., Tylenol). Patients should be informed that many over-the-counter medications contain aspirin and should be avoided as well.68,75
Gastroesophageal Reflux
The relationship between asthma and gastroesophageal reflux is controversial, although gastroesophageal reflux is nearly three times more prevalent in patients with asthma than in persons without asthma. Presumably, acid reflux into the esophagus causes vagal stimulation, resulting in a reflex increase in bronchial tone in patients with asthma. However, addition of a proton pump inhibitor to an asthma regimen has not been shown to enhance asthma control significantly.89
Asthma During Pregnancy
During pregnancy, one-third of patients have worse control of their asthma, one-third have better control of asthma, and one-third have asthma that is unchanged. The potential threat of adverse effects from asthma medications is far outweighed by the danger of uncontrolled asthma to the fetus and mother. Poorly controlled asthma during pregnancy can cause increased perinatal mortality, increased prematurity, and low birth weight. Theophyllines, beta-2 agonists, inhaled or oral corticosteroids, or cromolyn can be used during pregnancy without significant risk of fetal abnormalities.90
Sinusitis
Acute sinusitis and chronic sinusitis have been related to exacerbations and poor control of asthma by causing postnasal drip and interfering with nasal patency. A limited CT scan of the sinuses should be obtained for patients with uncontrolled asthma. If sinusitis is present, therapy with antibiotics for 2 to 3 weeks, nasal decongestants, and nasal corticosteroid inhalers may help improve asthma control.67,68
Surgery
Patients with asthma are predisposed to respiratory complications after surgery, including respiratory arrest during induction of anesthesia, hypoxemia and possible hypercapnia, impaired effectiveness of cough, atelectasis, and respiratory infection. The likelihood of these complications depends on the severity of the patient’s AHR, the degree of airflow obstruction, and the amount of excess airway secretions at the time of surgery. Optimizing the patient’s lung function before surgery, including the administration of perioperative corticosteroids, is an important strategy for minimizing perioperative complications.67,68
Bronchiectasis
Clinical Presentation
Bronchiectasis refers to the abnormal, irreversible dilation of the bronchi caused by destructive and inflammatory changes in the airway walls. Bronchiectasis has the following three major anatomic patterns91:
1. Cylindrical bronchiectasis: Airway wall is regularly and uniformly dilated
2. Varicose bronchiectasis: Irregular pattern, with alternating areas of constriction and dilation
3. Cystic bronchiectasis: Progressive, distal enlargement of the airways, resulting in saclike dilations
Bronchiectasis is thought to result from damage to the bronchial wall by chronic inflammation. Predisposing conditions are listed in Box 23-4.
Evaluation
The hallmark of bronchiectasis is the chronic production of large quantities of purulent sputum. Dyspnea is variable and depends on the extent of involvement and the underlying disease. Hemoptysis occurs frequently and is usually mild, but severe hemoptysis can be seen. Radiographic studies confirm the diagnosis by showing airway dilation. A chest radiograph may show cystic spaces and tram tracks (thin parallel lines representing the airway walls). CT is the diagnostic standard; the diagnosis of bronchiectasis is established when the diameter of the bronchus exceeds the diameter of the adjacent pulmonary artery branch.92 Because reversible airway changes consistent with bronchiectasis can follow pneumonia, CT should be deferred for 6 to 8 weeks after pneumonia resolves, when a diagnosis of bronchiectasis can be made.
Management
Antibiotics and bronchopulmonary hygiene are the mainstays of bronchiectasis management. Antibiotics can be given as needed or following a regularly scheduled regimen. Sputum cultures may be helpful in guiding antibiotic choice. Inhaled aminoglycosides may be a useful option for patients with chronic colonization by Pseudomonas aeruginosa. Infection by P. aeruginosa in bronchiectasis patients is a marker of severity but is not linked to accelerated decline in pulmonary function.93 Secretions can be cleared by chest physiotherapy with postural drainage, cough maneuvers, and humidification. Inhaled bronchodilators may be helpful in some patients because accompanying airflow obstruction is common.91 Inhaled hyperosmolar substances may be helpful in clearing secretion in patients with bronchiectasis. A Cochrane review concluded that dry powder mannitol improves tracheobronchial clearance in patients with bronchiectasis, patients with cystic fibrosis patients, asthmatics, and normal subjects.94 Hypertonic saline has not been specifically tested in bronchiectasis, but it improves clearance in these other conditions and in chronic bronchitis. In cases that are complicated by massive hemoptysis, embolization of the bleeding bronchial artery may be helpful. Surgical resection should be reserved for patients with localized disease who develop massive hemoptysis or who are severely symptomatic despite appropriate medical therapy.95–97