Chapter 75 Non-accidental Head Trauma
Inflicted head injury is a form of traumatic brain injury resulting from assault of typically, but not exclusively, infants and young children of less than 3 years of age. Despite considerable prevention efforts, it remains a major cause of morbidity and mortality among children [Aldrich et al., 1992; Bonnier et al., 2003; Chiesa and Duhaime, 2009; Gilles and Nelson, 1998]. Infants with inflicted head injuries have the most severe illness, the highest mortality risk, and the worst outcome in comparison with other forms of childhood trauma [Vavilala et al., 2007; Irazuzta et al., 1997; Pfenninger and Santi, 2002; Rivara et al., 1996]. They also have the greatest utilization (and therefore cost) of health-care resources in children with traumatic brain injury [Libby et al., 2003].
The National Child Abuse and Neglect Data System reported 1760 child fatalities in 2007, a rate of 2.35 deaths/100,000 children. Of these, physical abuse or multiple forms of maltreatment constituted over 60 percent of fatalities. Infant boys less than 1 year of age had a death rate of 18.85 deaths per 100,000 same-aged infant boys, while infant girls had a rate of 15.39 deaths per 100,000 same-aged infant girls. Forty-two percent were infants younger than 1 year, while 75 percent were less than 4 years old [http://www.childwelfare.gov/pubs/factsheets/fatality.pdf; http://www.acf.hhs.gov/programs/cb/pubs/cm07/cm07.pdf]. In one study, the incidence of inflicted head injury among children younger than 2 years was 17 per 100,000 children [Keenan et al., 2003]. Other studies suggest that as many as 60 percent of deaths resulting from abuse or neglect are not recorded [Crume et al., 2002].
Historical Perspective
The acceptance of inflicted head injury as an age-related subtype of traumatic brain injury and a type of physical abuse is a relatively recent phenomenon, although descriptions of this disorder were made at least as early as the 19th century [Tardieu, 1860]. Tardieu was the first to document the range of injury patterns found in abused and neglected children, as distinct from those in adults, and to link them to caregiver actions. Starting in the 1920s, fractures, subdural hematomas, and retinal hemorrhages in infants were associated with trauma, but correlating these findings with caregiver actions was not done clearly until the late 1940s and 1950s [Caffey, 1946, 1972, 1974; Silverman, 1952; Guthkelch, 1971; Frauenberger and Lis, 1950; Kempe et al., 1962; Lindenberg and Freytag, 1969; Woolley and Evans, 1955].
It was not until the 1960s and 1970s that the terms “battered child syndrome” and “shaken-baby syndrome” were first employed, and additional correlation made with specific findings and etiology [Kempe et al., 1962; Lindenberg and Freytag, 1969; Guthkelch, 1971; Caffey, 1972; Caffey, 1974]. The term “battered child syndrome” was coined by John Kempe to describe severe physical abuse with fractures, bruises, failure to thrive, brain injury, and subdural hematomas [Kempe et al., 1962]. Subsequently, Guthkelch and Caffey independently hypothesized that head injury in the absence of obvious blunt force trauma was caused when a caregiver held the infant around the chest and “whiplashed” the head by shaking the baby (as some infants had associated rib fractures) [Guthkelch, 1971; Caffey, 1972, 1974]. Rotational movement of the head on the neck can, of course, be caused by a number of ways, not just shaking. Also in 1974, Caffey first used the term “shaken baby syndrome” for infants with severe head injuries but lacking signs of blunt force trauma on imaging studies. A number of pathologic studies have since confirmed that many infants without obvious signs of blunt force trauma antemortem frequently show evidence of impact trauma postmortem, and not infrequently with involvement at many impact sites [Duhaime et al., 1987; Hicks and Gaughan, 1995; Gill et al., 2009].
An important first step in accepting inflicted neurotrauma as a distinct form of trauma was recognizing that the history offered by the caregiver was biomechanically inconsistent with the severity of injury [Duhaime et al., 1987, 1992; Billmire and Myers, 1985; Goldstein et al., 1993; Weston, 1973]. Few authors have specifically addressed the discrepancies between initial historical data and subsequent caregiver admissions [Gilles and Nelson, 1998; Tardieu, 1860; Weston, 1973; Starling et al., 2004; Reece and Sege, 2000; Krugman, 1983].
Over the past three decades, our knowledge of inflicted childhood neurotrauma has increased significantly [Chiesa and Duhaime, 2009; Christian and Block, 2009]. It is important to realize that the majority of the relevant literature is observational and retrospective in nature. Many studies have failed to specify explicit inclusion or exclusion criteria, and have accepted, with minimal or no question, histories obtained from perpetrators. While prospective studies continue to be in the minority due to the inherent difficulties in defining abusive injury, both clinical and laboratory investigations increasingly are examining the unique nature of early life inflicted injury.
Terminology
It is recommended to use the terms inflicted, nonaccidental, or abusive head injury when referring to neurotrauma from assault in children [Christian and Block, 2009]. These are inclusive terms covering the range of craniospinal injuries sustained by infants and young children as a consequence of violent assault by parents or other caretakers. Unintentional, or accidental, head injury refers to injury sustained by chance and is unrelated to specific deliberate actions of caretakers.
There are a number of problems with using lay terminology, such as shaken baby syndrome and shaken-impact infant syndrome. These terms imply a uniform injury event sequence, mechanism of injury, and age at time of injury. The actual injury and postinjury event sequences are often much more complex [Gilles and Nelson, 1998; Duhaime et al., 1987; Alexander et al., 1990; Budenz et al., 1994; Pollanen et al., 2002].
Developmental Differences Predisposing the Immature Neuraxis to Injury
Structural and functional changes of central and peripheral nervous system organization and function are greatest during the first 2 years of life, when nonaccidental head injury occurs most frequently [Bauer and Fritz, 2004; Johnston, 1995; Robertson et al., 2006; Gilmore et al., 2007; Vexler et al., 2006; Law et al., 2003; Rice and Barone, 2000; Pouwels et al., 1999; Brody et al., 1987]. A number of biologic and mechanical factors predispose the infant and young child to injury of the craniospinal axis (Box 75-1). Of these, the most important are biomechanical factors and differences in postinjury response cascades.
Box 75-1 Biologic and Mechanical Features Predisposing the Infant to Head Injury
Mechanical Factors
The infant is more biomechanically vulnerable to significant applied forces than the older child and adult due to a larger head to body ratio and less developed neck and shoulder girdle musculature. The higher water content of the brain, coupled with incomplete myelination, contributes to distensibility and compliance of brain tissue during injury events and predisposes the infant to greater shear strains with angular and translational acceleration [Bauer and Fritz, 2004; Brody et al., 1987; Prange et al., 2003]. On the other hand, the relatively smooth inner cranial table and greater skull compliance decrease risk for contusion, as well as dispersing some force into the skull with impact [Lindenberg and Freytag, 1969; Carter and McCormick, 1983; Courville, 1965; Calder et al., 1984; Jaspan et al., 1992]. These features reinforce the observation that trivial trauma does not appear sufficient to cause the kinds of injuries seen in the context of abuse [Brody et al., 1987; Prange et al., 2003].
Biologic Factors
There are numerous age-related biochemical, microscopic, and macroscopic age-dependent differences in the immature versus mature central nervous system (CNS) [Bauer and Fritz, 2004; Pouwels et al., 1999]. Synaptic density in human infants is 50–60 percent above the adult mean [Novack et al., 1996; Teasdale and Graham, 1998]. Neuronal synapses and receptors differ in structure, number, and function [Azzarelli et al., 1996; Herlenius and Lagercrantz, 2004]. Among the many unique biochemical features of the developing brain are greater potential to excitability due to increased excitatory amino acids, and robust neuronal and synaptic networks [Herlenius and Lagercrantz, 2004; Johnston, 1996; Kubova and Moshe, 1994; McDonald et al., 1992; Wasterlain et al., 2002].
Responses to Injury
Injury and repair after a CNS insult are quite different in the immature versus the mature CNS. The biochemical and physiological responses to injury of the immature nervous system are unique and may render the infant more vulnerable to injury [Zupanc, 2009; Giza et al., 2007; Bauer and Fritz, 2004; Bittigau et al., 2003]. The immature CNS has decreased ischemic and seizure thresholds, especially after trauma and hypoxic-ischemic injury [Goldstein et al., 1993, 1998; Johnston, 1995; Kubova and Moshe, 1994; Adelson et al., 1997; Hagberg, 2004; Ruppel et al., 2002; Chiaretti et al., 2009; Lea and Faden, 2001; Satchell et al., 2005; Robertson et al., 2009; Duhaime et al., 2003; Raghupathi et al., 2004; Missios et al., 2009; Duhaime and Durham, 2007; Freeman et al., 2008; Wong et al., 2008; Pryds et al., 2005].
Other mediators of secondary injury include inflammatory and excitotoxic mechanisms, oxidative stress, and apoptosis [Ruppel et al., 2002; Chiaretti et al., 2009; Bittigau et al., 2003]. For instance, in the infant brain, neuronal apoptosis predominates as a cause of neuronal cell death, whereas in the adult brain, neuronal cell death is more associated with necrosis [Vexler et al., 2006; Lea and Faden, 2001]. Cytochrome C, a marker of apoptosis, has been shown to be increased in young children with inflicted head injury compared to a group of children with unintentional head injury [Satchell et al., 2005]. Mitochondrial dysfunction in the face of significant oxidative stress also plays a role [Robertson et al., 2009].
The immature gyrencephalic brain (having convolutions) is more resistant to direct cortical deformation and isolated subdural hematoma than the mature brain, but is more sensitive to white-matter strains [Duhaime et al., 2003; Raghupathi et al., 2004; Missios et al., 2009]. Specific vulnerability likely depends on maturational factors, as well as simultaneous physiologic stresses such as hypoventilation and seizures, and is the focus of on-going research [Duhaime and Durham, 2007].
On a more macroscopic level, age less than 4 years is a risk factor for development of impaired cerebral autoregulation, regardless of severity of traumatic brain injury in humans as autoregulation is not fully functional in premature infants and in animal studies [Freeman et al., 2008; Wong et al., 2008; Pryds et al., 2005; Tuor and Grewal, 1994; Zernikow et al., 1994]. Efficiency of the autoregulatory response improves with age [Tuor and Grewal, 1994]. Further, the more severe the abnormalities of cerebral autoregulation, the greater potential for reduced cerebral blood flow throughout the cortex and subcortical white matter in infants, and the worse the outcome, particularly with inflicted injury [Vavilala et al., 2004; Chaiwat et al., 2009].
Mechanisms of Injury
Basic principles of the mechanisms of head injury are the same for all ages and are reviewed in Chapter 74. As with other tissues, brain injuries occur when applied forces strain the tissue beyond its structural or functional tolerance. Differences exist in the specific patterns of response at different ages. When modeled biomechanically, significant forces are required to cause the type of injuries seen in children with severe inflicted trauma [Duhaime et al., 1987; Prange et al., 2003]. In general, greater applied forces result in more severe injuries, regardless of whether the injury was inflicted.
The exact type and the magnitude of applied forces determine the severity of injury. Most severe primary brain injuries in the clinical world result from head contact, because the contact, and the inertial forces generated when the head stops suddenly against a surface, are much higher than those produced by noncontact mechanisms, where the change in velocity occurs much more slowly and therefore the deceleration magnitude is much smaller. Rotational movement of the head from side to side (lateral movement) is associated with more brain damage than equivalent movements in the anterior–posterior direction, regardless of whether there is impact [Xiao-Sheng et al., 2000; Zhang et al., 2009].
Contribution of hypoxia-ischemia
Developmental differences alone fail to explain why children with inflicted head injury have more severe illness and a higher mortality risk than do children of the same age with unintentional head injury [Hymel et al., 2007; Case, 2008; Ichord et al., 2007; Vinchon et al., 2005]. There are numerous age-related differences in regional cerebral blood flow, autoregulatory mechanisms, and regional sensitivity to hypoxia, which all contribute to enhanced vulnerability to secondary ischemic brain damage initiated by mechanical brain injury.
Infant animals and human children less than 24 months of age seem particularly prone to secondary injury from hypoxia-ischemia after severe traumatic brain injury [Adelson et al., 1997; Ashwal et al., 2000]. In inflicted head injury, it appears to be the most significant factor contributing to the evolution of parenchymal injury and deleterious outcome [Aldrich et al., 1992; Ashwal et al., 1996; Biousse et al., 2002; Duhaime et al., 1993; Hymel et al., 2007; Duhaime and Durham, 2007; Pigula et al., 1993]. Immature medullary respiratory drive and lowered threshold to disruption of respiratory and cardiovascular drive may be the basis of the prolonged apnea and hypotension observed in severely injured young children [Aldrich et al., 1992; Goldstein et al., 1993; Bittigau et al., 2003; Adelson et al., 1997; Pigula et al., 1993; Goldstein et al., 1996].
Trauma-induced hypoxia and hypotension are strongly associated with adverse outcomes in adults and children and are an index of neurologic insult and shock [Gilles and Nelson, 1998; Hymel et al., 2007; Ichord et al., 2007; Kemp et al., 2003; Chesnut et al., 1993; Marton et al., 2007; Chiaretti et al., 2000]. Most infants with severe head injury, inflicted or not, experience apnea or altered respiration during or just after injury [Gilles and Nelson, 1998; Kemp et al., 2003; Johnson et al., 1995]. Apnea and hypotension can also be a response to smothering or to direct vessel compromise caused by strangulation [Shaikh et al., 2001; Ashwal et al., 1991].
Clinical Features
Acute Presentation
Regardless of age, there is a predictable pattern of brain response to mechanical trauma, whether blunt force, primarily angular acceleration-deceleration, or both. Studies have demonstrated repeatedly that severe primary brain injuries result in immediate decrease in the level of consciousness, apnea, and initial hypertension (from catecholamine surge), followed by hypotension [Atkinson, 2000; Denny-Brown and Russell, 1941; Ommaya and Gennarelli, 1974]. An apneustic response (abnormal breathing pattern with deep, gasping inspirations followed by brief insufficient expiration) is triggered, arising from disruption of the cerebral-brainstem reticular activating system and respiratory centers of the upper medulla/lower pons, or from loss of afferent vagal activity.
Symptoms of brain injury are nonspecific, and vary by the severity of injury. The classic presentation is that of a previously healthy infant who suddenly decompensates neurologically and develops severe respiratory distress and flaccidity, with or without seizures or posturing (usually extensor) in the absence of any trauma history [Talbert, 2008; Biron and Shelton, 2007; Arbogast et al., 2005; Gilles and Nelson, 1998; Kemp et al., 2003; Fujiwara et al., 2008]. Following initial loss of consciousness and apnea of variable duration, infants may remain apneic or develop other breathing patterns correlating with the severity of respiratory drive disruption. Less severely injured infants with concussive symptoms may exhibit a depressed level of consciousness, irritability, altered behavior, seizures, decreased feeding, vomiting, fever, and altered respiration. They may not appear sick enough for the perpetrator to seek medical attention. The accompanying caregiver may be unaware of the injury or may be the nondisclosing perpetrator.
Persistence of coma, apnea or respiratory distress, irritability, seizures, hypotonia, and vomiting reflects the severity of mechanical and physiologic disruptions and evolving secondary pathophysiologic cascades [Fujiwara et al., 2008; Gilles and Nelson, 1998; Goldstein et al., 1993; Johnson et al., 1995]. Infants who are severely injured cannot regulate behavior that requires higher cortical functions, such as coordinated action, eating, sitting, and walking. As Graham and colleagues noted, if an individual who sustains a head injury is able to talk, brain damage at the moment of injury cannot have been overwhelming [Graham et al., 1989].
Infants with increased intracranial pressure may have a bulging fontanel and diastatic sutures. Young children with inflicted brain injury develop the same patterns of brainstem abnormalities (pupils, eye movements, and breathing) as children and adults with severe unintentional brain injury [Gilles and Nelson, 1998; Johnson et al., 1995; Atkinson et al., 1998; Hahn et al., 1983].
Early Post-Traumatic Seizures
Early post-traumatic seizures (those occurring in the first 72–96 hours after trauma) are more common in infants and young children with inflicted head injury than in older children or adults with unintentional head injury or cerebral infarction from other etiologies [Chiaretti et al., 2000; Johnson et al., 1995; Barlow et al., 2000; Bechtel et al., 2004; Myhre et al., 2007]. The majority of early post-traumatic seizures start in the first 24 hours after trauma, and although seizures can accompany injury events, extensor posturing is more common after injury [Chiaretti et al., 2000; Johnson et al., 1995; Barlow et al., 2000; Bechtel et al., 2004; Myhre et al., 2007; Lewis et al., 1993]. Seizures are most commonly partial, partial complex, or partial, with or without secondary generalization. Clinical signs may be subtle or even absent. Although the onset of symptoms generally correlates with the timing of injury, clinically evident early post-traumatic seizures cannot and should not be used to time the injury events.
The frequency of seizures ranges from 30 to 80 percent in infants with nonaccidental injury and seems to vary in part as a function of the severity of injury, especially the severity of the degree of hypoxia-ischemia [Gilles and Nelson, 1998; Chiaretti et al., 2000; Johnson et al., 1995; Barlow et al., 2000; Bechtel et al., 2004; Myhre et al., 2007; Lewis et al., 1993]. Early post-traumatic seizures correlate with inflicted mechanism of injury, age younger than 18 months, loss of consciousness, low Glasgow Coma Scale score, and abnormal computed tomographic (CT) scan findings [Gilles and Nelson, 1998; Vinchon et al., 2005; Marton et al., 2007; Myhre et al., 2007; Lewis et al., 1993; Golden and Maliawan, 2005].
Early post-traumatic seizures potentiate postinjury ischemic cascades in the immature injured brain by compromising oxygen and substrate delivery to neurons and, if unrecognized, can be the source of severe secondary injury [Gilles and Nelson, 1998; Vinchon et al., 2005; Marton et al., 2007; Myhre et al., 2007; Lewis et al., 1993; Golden and Maliawan, 2005; Chiaretti et al., 2000; Diaz-Arrastia et al., 2009; Hossain, 2005]. The high incidence of early post-traumatic seizures and the difficulty encountered in their control support the early use of prophylactic antiepileptic medication in the child with severe inflicted closed head injury, particularly if a cerebral infarction syndrome is suspected. Only one study has examined this issue specifically, but as the majority did not sustain inflicted injury and did not have seizures, the efficacy of this intervention remains unproven [Young et al., 2004].
Post-traumatic epilepsy develops significantly more frequently in young children after inflicted head injury, compared with children with unintentional injury or adults after closed head injury [Gilles and Nelson, 1998; Diaz-Arrastia et al., 2009; Talvik et al., 2007; Ates et al., 2006]. The rate varies by study, but is generally in the 20–30 percent range, compared with less than 2 percent in adults [Gilles and Nelson, 1998; Barlow et al., 2000; Diaz-Arrastia et al., 2009; Talvik et al., 2007; Ates et al., 2006; Ghahreman et al., 2005; Singer, 2001]. Types of post-traumatic epilepsy syndromes are typically motor or partial complex. Of infants less than 6 months old at the time of injury who sustain severe hypoxic-ischemic injury, an occasional individual will develop infantile spasms 3–6 months after injury.
Sequelae of Inflicted Head Injury
Outcome data regarding inflicted childhood head injury have been accumulating since the 1950s. In contrast to the majority of children with severe unintentional head injury, who tend to be older and generally have better outcomes, infants have a higher mortality rate and significant sequelae [Bonnier et al., 2003; Gilles and Nelson, 1998; Duhaime et al., 1987; Goldstein et al., 1993; Kemp et al., 2003; Hymel et al., 2007; Keenan et al., 2006]. The majority of severely injured infants initially become macrocephalic, frequently with diastatic sutures and bulging fontanels. With time, cerebral atrophy and associated microcephaly develop, with enlargement of the cerebrospinal fluid spaces [Bonnier et al., 2003; Gilles and Nelson, 1998; Lo et al., 2003]. The development of posttraumatic hydrocephalus is less common.
Predictors of poor outcome are similar to those for other types of head injury. Clinically, these include low scores on the Glasgow Coma Scale or other infant coma indices, early hyperglycemia, extensor posturing, lack of brainstem reflexes on presentation, and coma duration of more than 7 days (Box 75-2) [Johnson et al., 1995; Chiaretti et al., 2002; Cochran et al., 2003; Durham et al., 2000; Prasad et al., 2002]. In inflicted head injury, age less than 4 years, type and repetitiveness of injury events, severity of brain swelling and subsequent brain atrophy, post-traumatic seizures, presence and number of focal lesions, and severe retinal hemorrhages are other important predictors. Cognitive and motor outcome correlates directly with severity of parenchymal injury [Bonnier et al., 2003; Prasad et al., 2002; Barlow and Minns, 1999].
There are an increasing number of markers of brain damage being studied as potential prognostic indicators after early-life traumatic brain injury. These include some that have been in use for a number of years, such as neuron-specific enolase and S100B [Chiaretti et al., 2009; Beers et al., 2007]. More recently, a number of other biomarkers, such as doublecortin, a neurotrophic factor, interleukin-6 (IL-6), IL-8, IL-10, soluble intracellular adhesion molecule (SICAM), L-selectin, and endothelin, have also been studied, with significant outcome predictability using paired biomarkers [Lo et al., 2009]. This was recently reviewed by Kochanek et al [Kochanek et al., 2008].
One of the most significant findings is the proportion of survivors developing microcephaly, a key indicator of the severity of brain injury in these young children. Up to 100 percent of severely injured children had evidence of cerebral atrophy on later imaging, typically evident within 1–2 months after admission [Gilles and Nelson, 1998; Lo et al., 2003; Perez-Arjona et al., 2003]. Visual impairment and behavioral, cognitive, and motor disabilities are common after inflicted head injury, and infants have an increased likelihood of developing post-traumatic epilepsy [Bonnier et al., 2003; Gilles and Nelson, 1998; Kemp et al., 2003; Duhaime et al., 1996; Ewing-Cobbs et al., 1999; Gilles et al., 2003; Haviland and Russell, 1997].
Cognitive and Executive Function
More than 50 percent of survivors have severe developmental disabilities (many vegetative and nonambulatory), with a sizeable proportion completely dependent for daily care [Hymel et al., 2007; Duhaime et al., 1996; Ewing-Cobbs et al., 1998, 1999; Barlow et al., 2005; Keenan et al., 2007]. Of these, 20–30 percent have post-traumatic epilepsy [Gilles and Nelson, 1998; Barlow et al., 2005]. Cognitive and executive functions are often severely impaired, with attention and emotional regulation frequently affected [Barlow et al., 2005; Keenan et al., 2007; Nadebaum et al., 2007]. Many survivors who are interactive develop pseudobulbar palsy.
Behavioral Sequelae
Similar to survivors of severe traumatic brain injury at other ages, behavioral problems are common, ranging from perseveration to oppositional behavior. Temper tantrums occur frequently and may begin to manifest as the child reaches a functional age of 2 years [Ewing-Cobbs et al., 1999; Barlow et al., 2005]. There are no systematic studies as to the most effective interventions for these behavioral disorders.
Visual Sequelae
Sequelae of ocular pathology are common, with 32–90 percent of children having visual impairments [Gilles et al., 2003; Barlow et al., 2005; Kivlin et al., 2008; McCabe and Donahue, 2000]. Cortical visual impairment contributes more to long-term visual deficits than do anterior visual defects, and is most frequently a homonymous hemianopia [Gilles et al., 2003; Kivlin et al., 2008]. Retinal hemorrhages generally resolve by 4 months after admission; those that are small resolve without compromising vision [McCabe and Donahue, 2000]. Larger hemorrhages, especially those near or obscuring the macula, may affect central vision permanently [McCabe and Donahue, 2000; Ehrenfest, 1922]. A few infants require vitrectomy for persistent vitreal hemorrhages.
Motor Sequelae
Motor deficits are exceedingly common and are found in over 50 percent of survivors of early-life abuse [Hymel et al., 2007; Barlow et al., 2005; Holloway et al., 1994]. Typical features are spastic hemiparesis, often superimposed on quadriparesis; spastic quadriparesis, often with truncal hypotonia; and, not infrequently, mixed tone, with elements of dystonia or choreoathetosis. Severe extensor posturing is not uncommon.
Neuroimaging
Findings of diffuse hypodensity and evidence of shear injury are predictive of worse outcome, regardless of imaging modality. T2-weighted and fluid-attenuated inversion-recovery imaging (FLAIR), diffusion abnormalities, and susceptibility images are most predictive of injury severity and outcome on magnetic resonance imaging (MRI) [Babikian et al., 2005; Foerster et al., 2009; Galloway et al., 2008; Sigmund et al., 2007; Suh et al., 2001; Tong et al., 2003]. Reduced N-acetylaspartate and elevated lactate levels on MR spectroscopy correlate with poor outcomes [Aaen et al., 2010; Ashwal et al., 2006; Babikian et al., 2006]. Long-term imaging anomalies include diffuse cortical atrophy, generalized white-matter attenuation, multicystic encephalomalacia, porencephaly, and ulegyria [Bonnier et al., 2003; Geddes et al., 2001; Marin-Padilla et al., 2002]. The presence and extent of parenchymal abnormalities on imaging at 3 months post injury is negatively predictive of neurodevelopmental outcome [Bonnier et al., 2003].
Pathologic Features
A broad range of physical findings is associated with inflicted head injury [Wygnanski-Jaffe et al., 2009; Tung et al., 2006]. Not all injuries have to be present to make the diagnosis, and no individual markers are pathognomonic of abusive injury. The major markers of injury are outlined in Box 75-3.
Extracranial Injuries
Scalp
The most common extracranial impact injuries in inflicted injury are scalp bruising, soft-tissue swelling, cephalohematoma, and subgaleal hematoma (Figure 75-1) [Duhaime et al., 1987; Case, 2008; Bechtel et al., 2004; Rubin et al., 2003]. Scalp bruising may be difficult to discern clinically [Atwal et al., 1998; Peters et al., 2008]. Skull fractures and subgaleal hematomas are more commonly associated with bruising than extremity fractures [Peters et al., 2008]. Atwal and associates found that facial and forehead bruising predominated in a series of fatal inflicted head injury, whereas limb and chest bruising were uncommon; this suggests that slapping or punching of the head, not gripping of the chest or arms and shaking, was more common in that series. Other authors have found a predominance of parieto-occipital sites for contact injuries. Subgaleal hematomas from abuse result from shearing injury to the scalp from impact or from a violent hair pulling [Anton et al., 1999; Hamlin, 1968; Greenes and Schutzman, 1998; Case et al., 2001; Ewing-Cobbs et al., 2000; Geddes et al., 2001; Shane and Fuchs, 1997; Kemp et al., 2008; Hobbs, 1984; Meservy et al., 1987; Arnholz et al., 1998; Banaszkiewicz et al., 2002; Jayakumar et al., 2010; Hiss and Kahana, 1995].
Skull Fractures
A variety of skull fractures have been reported after blunt trauma, regardless of etiology (see Figure 75-1). As such, they are not specific to either unintentional or inflicted trauma [Greenes and Schutzman, 1998]. Depending on the study, between 25 and 74 percent of infants and children with inflicted neurotrauma sustain skull fractures [Duhaime et al., 1992; Case et al., 2001; Ewing-Cobbs et al., 2000; Geddes et al., 2001; Shane and Fuchs, 1997; Kemp et al., 2008]. Simple linear fractures are the most common, and tend to be parietal or occipital in location [Rubin et al., 2003; Shane and Fuchs, 1997; Hobbs, 1984; Meservy et al., 1987].
In general, fractures that are complicated – i.e. bilateral, comminuted, multiple, or that cross suture lines – are more suggestive of being inflicted [Shane and Fuchs, 1997; Hobbs, 1984; Meservy et al., 1987; Arnholz et al., 1998; Banaszkiewicz et al., 2002; Jayakumar et al., 2010]. In one study of children with inflicted fractures, a skull fracture was most commonly associated with bruising or subgaleal hematoma at or near the fracture site [Peters et al., 2008]. Fractures in infants with open sutures generally do not usually cross suture lines. Bilateral or other multiple fractures usually indicate that there was more than one impact but have also been reported after simultaneous, severe head compression between two unyielding surfaces or from direct occipital impacts [Arnholz et al., 1998; Hiss and Kahana, 1995]. In rare cases, complicated fractures occur after falls.
Intracranial Injuries
In most severely injured children, neuroimaging reveals intracranial abnormalities, most often acute subdural hematoma, cerebral hypodensity, and intraparenchymal hemorrhage (Figure 75-2, 75-3, and 75-4; see also Figure 75-5A and 75-9) [Bonnier et al., 2003; Chiesa and Duhaime, 2009; Reece and Sege, 2000; Myhre et al., 2007; Datta et al., 2005].
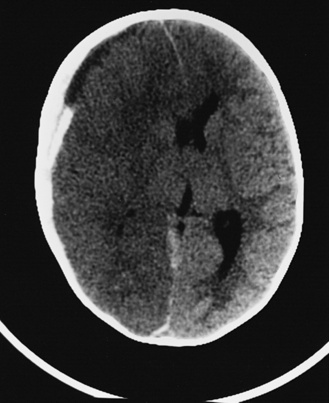
Fig. 75-4 Nonenhanced computed tomographic scan with an acute mixed-density right subdural hematoma.
Subdural Hematoma
Regardless of etiology, subdural hematomas are the most common intracranial pathological abnormality found in inflicted head injury. They are observed in up to 100 percent of infants and young children with inflicted neurotrauma [Foerster et al., 2009; Vinchon et al., 2005; Golden and Maliawan, 2005; Fernando et al., 2008; Vinchon et al., 2004; Kivlin, 2001; Duhaime et al., 1992; Feldman et al., 2001]. In fatal cases, some blood is almost always present in the subdural space.
Subdural hematomas associated with inflicted injuries in children are typically interhemispheric, unilateral, or bilateral over the cerebral convexities (see Figure 75-2, 75-3, 75-4, 75-5, and 75-9) [Biousse et al., 2002]. Interhemispheric or falcine subdural hematomas are particularly suspect for inflicted injury. Significant convexity or interhemispheric subdural hematomas with associated neurologic signs are extremely rare as a result of any etiology other than trauma. In adults, these are most often observed in the context of moderate to severe accidental traumatic head injury (see Figure 75-2 and 75-3) [Tzioumi and Oates, 1998].
Subdural hematomas develop from several mechanisms, alone or in combination, including the tearing of bridging veins extending from the cortex to the dural sinuses (particularly the superior sagittal sinus), injured surface vessels (about 10 percent), and dural lacerations [Case, 2007; Gennarelli et al., 1982; Matsuyama et al., 1997; Shenkin, 1982; Stålhammer, 1986]. Less commonly, they arise from the rupture of cortical arteries [Matsuyama et al., 1997]. Acute subdural hematomas typically liquefy and are resorbed, but may persist as chronic subdural effusions, and even become chronic and eventually calcify [Zouros et al., 2004; Tokmak et al., 2007].
Mixed-density or “hyperacute” subdural hematoma
Mixed-density subdural hematomas on CT scanning in an acutely injured individual are frequently misdiagnosed as “acute on chronic” subdural hematoma. Unlike the older individual with a chronic hematoma and a clearly delineated membrane, the mixed-density subdural collection in the acutely injured infant results from rapidly accumulating blood in the potential subdural space, and at surgery is typically found to be associated with fresh nonclotted blood with no evidence of an organized membrane [Zouros et al., 2004].
Other potential mechanisms for a mixed-density appearance are settling, washout, or compartmentalization of hemorrhage products (Figure 75-6; see also Figure 75-4 and 75-9) [Tung et al., 2006; Greenberg et al., 1985; Sargent et al., 1996]. Sometimes, cerebrospinal fluid mixed with blood in a subdural hematoma may also appear to be of mixed density (see Figure 75-4) [Zouros et al., 2004; Vinchon et al., 2009]. When seen in the context of an injured infant or young child, it is significantly more likely to be due to abuse [Tung et al., 2006]. Not infrequently, this finding is associated with abnormalities of coagulation [Greenberg et al., 1985].
Chronic subdural hematoma
Chronic subdural hematomas develop when an acute subdural hematoma fails to organize, either because of intrinsic abnormalities in coagulation within the clot, or more often because of rebleeding or exudation into the well-defined outer and inner membrane [Tokmak et al., 2007; Shim et al., 2007]. There is a predictable sequence in their growth, with the development of a membrane around the liquefying clot, and then neovascularization within, and finally ossification [Walter et al., 2009].
Chronic subdural effusions
Proteinaceous subdural collections frequently evolve from resolving acute subdural hematomas in young infants (Figure 75-7 and 75-8; see also Figure 75-6). In infants with traumatic subdural hematoma, the evolution to a chronic subdural collection (hygroma or effusion) may be associated with tears in the arachnoid and may not have the classic membrane structure [Zouros et al., 2004]. They may develop within days of the injury [Vinchon et al., 2009]. Sometimes referred to as subdural hygromas, they either resolve with time, or persist when there is diffuse brain injury with secondary atrophy [Hasegawa et al., 1992; Kaufman, 1993; Wetterling et al., 1988].
Subdural hygromas are defined as accumulations of fluid in the subdural space. The use of the term hygroma is controversial [Wetterling, 1988 #6429]. Like many terms, it came into use before more sophisticated imaging, such as MRI, was available to determine noninvasively the variability in the characteristics of extra-axial collections. Subdural hygromas are variably attributed to resolving subdural hematomas or to abnormal collections of cerebrospinal fluid, alone or mixed with blood, in the subdural space [Zouros et al., 2004]. Analysis of attenuation coefficients on CT scan is often adequate for making the diagnosis. If there is any question, MRI is the preferred technique, as it is accurate in determining whether extra-axial fluid is of cerebrospinal fluid intensity and whether fluid is in the subarachnoid or subdural space [Hasegawa et al., 1992; Wilms et al., 1993]. The majority of subdural collections resolve without intervention (see Figure 75-8).
Subarachnoid Hemorrhage
Subarachnoid hemorrhage develops after direct surface trauma to the brain, or from leakage of blood from injured cerebral vessels within the subarachnoid space. Subarachnoid hemorrhage is difficult to identify on CT scans and to differentiate from subdural hematoma, especially when located interhemispherically. In the setting of trauma, most patients with subdural hemorrhage also have subarachnoid hemorrhage, because of tearing of the arachnoid at the time of the injury. Abused infants and young children are no exception [Golden and Maliawan, 2005].
Epidural Hematoma
Arterial epidural hematoma is uncommon after trauma, especially after inflicted head injury [Gerlach et al., 2009; Schutzman et al., 1993; Shugerman et al., 1996]. It is discussed here to clarify its pathogenesis and clinical course. Both venous and arterial epidural hematomas can occur after relatively minor trauma [Schutzman et al., 1993]. The majority of arterial epidural hematomas develop adjacent to the middle meningeal artery or over the cerebral convexity, but they can also occur in the posterior fossa and are usually venous, rather than arterial, in this location. Venous epidural collections most often result from skull fractures, where the bone itself is the source of hemorrhage; these are most often low-pressure hemorrhages and usually are self-limited.
Arterial epidural hematomas typically accumulate more rapidly and result in larger and more clinically significant clots. Skull fracture is present in two-thirds of cases of arterial epidural hematomas. Epidural hematomas develop when the middle meningeal artery is torn as the result of temporal bone deformation. Fracture is not always a consequence, particularly in an infant, whose increased skull compliance allows increased skull deformation at the impact site [Shugerman et al., 1996]. In this situation, locally deforming forces do not usually injure brain parenchyma away from the impact site. Symptom progression varies from dramatic to more protracted, paralleling the rate of epidural blood accumulation.
Children with epidural hematomas are much more likely to have a lucid interval and do well clinically than are severely abused infants (see also “Timing” section) [Schutzman et al., 1993]. This finding is congruent with the biomechanics of injury because epidural hematomas result primarily from brief linear deceleration impacts, such as falls [Schutzman et al., 1993; Shugerman et al., 1996]. These children typically do well with prompt surgical intervention when needed and aggressive supportive care [Gerlach et al., 2009].
Brain Injuries
Brain swelling
Diffuse or focal brain hypodensity is the most common parenchymal pattern found on initial CT imaging [Duhaime and Durham, 2007; Kemp et al., 2003; Golden and Maliawan, 2005; Kazan et al., 1997; Rao et al., 1999]. Younger infants are more likely to present with diffuse hypodensity, with or without bilateral acute subdural hematomas, presumably reflecting the greater likelihood of diffuse hypoxic-ischemic insult (see Figure 75-5 and 75-8). Older infants and children more often present with focal or hemispheric brain swelling and ipsilateral acute subdural hematomas (Figure 75-9; see also Figure 75-4) [Gilles and Nelson, 1998; Duhaime et al., 1992; Kemp et al., 2003]. The particular pattern of severe bilateral hemispheric hypodensity is rare in adults, but not uncommon in infants after severe injury [Gilles and Nelson, 1998; Duhaime et al., 1992; Kemp et al., 2003]. The so-called “black brain” signifies profound parenchymal injury and secondary swelling [Duhaime and Durham, 2007]. Traumatic brainstem lesions are not common [Brennan et al., 2009; Eder et al., 2000].
Focal swelling has several patterns: hemispheric subjacent to an ipsilateral acute subdural hematoma, less often in a vascular distribution from an evolving infarction, or patchy hypodensity not in a vascular distribution. None of these patterns is specific for abuse [Lobato, 1993; Sarabia et al., 1988; Tomita et al., 1997]. Although there is a tendency to infer that focal brain swelling equates to localized brain injury, the finding of persistent coma in severely injured children in the absence of significant midline shift is supportive evidence that diffuse injury has also occurred.
Hemispheric swelling
Apnea or hypotension alone does not completely explain why one-third of abused infants and young children present with hypodensity affecting one hemisphere, in so far as these processes would be expected to be symmetric, so some synergy among pathophysiologic stressors appears to be at play [Gilles and Nelson, 1998; Duhaime and Durham, 2007; Duhaime et al., 1993]. In adults, asymmetric hemispheric swelling is associated with the highest mortality rate [Eisenberg et al., 1990; Lobato et al., 1988].
The etiology of hemispheric swelling and secondary necrosis subjacent to an acute subdural hematoma is not well understood. Putative mechanisms include the adverse effects of local pressure and midline shift (decreasing cerebral metabolic rate of oxygen), association with cortical ischemia, and decreased glucose metabolism [Miller et al., 1990; Valadka et al., 2000; Baechli et al., 2010; Schroder et al., 1994]. When hypoxia is added to the mix, it appears to result in further decreases in cerebral blood flow and severe brain swelling [Orlin et al., 1992; Sawauchi et al., 2004; Tomita et al., 1997].
Strangulation has been proposed as a causal mechanism of brain swelling, particularly hemispheric swelling, but corroborative clinical data are limited (Figure 75-10 and Figure 75-11) [Gilles and Nelson, 1998; Duhaime et al., 1996; Bird et al., 1987; Bonnier et al., 1995; Drack et al., 1999]. Circumferential strangulation in a young child can simultaneously compress carotid and vertebral arteries (the carotid arteries by anterior neck compression, and the vertebral arteries as they pass through the scalene muscles) [Rossen et al., 1943]. Traumatic vascular injury secondary to strangulation should be diagnosed only if there is unequivocal clinical, imaging, or postmortem examination evidence.
Cerebral infarction
Post-traumatic cerebral infarction develops after traumatic brain injury in both children and adults, predominantly but not exclusively as a result of vascular compression and herniation [Gilles and Nelson, 1998; Mirvis et al., 1990; Server et al., 2001; Steinborn et al., 2010]. Post-traumatic cerebral infarction after inflicted head injury has received relatively little attention [Gilles and Nelson, 1998; Bird et al., 1987; Ransom et al., 2003; Zimmerman et al., 1979]. Development of a post-traumatic infarction is more likely if the child is abused, but the actual frequency is unknown [Ransom et al., 2003]. In adult studies, the frequency ranges from 8 to 19 percent. Posterior cerebral artery and branch anterior cerebral artery distribution infarctions predominate (see Figure 75-7 and 75-9). Infarction in the lenticulostriate-thalamoperforator distribution leads to basal ganglia and thalamic injury. Hemispheric necrosis and border zone infarction are other prominent patterns (see Figure 75-9). The majority of infarctions in adults are also in vascular territories localizing to middle cerebral artery, lenticulostriate arteries, and posterior and anterior cerebral arteries, but border zone infarctions also occur [Marino et al., 2006]. In selected patients, evaluation for carotid arterial dissection needs to be considered.
The extent of infarction is correlated with poor outcome [Gilles and Nelson, 1998; Server et al., 2001]. Risk factors in adults include Glasgow Coma Scale score, hypotension, brain herniation and decompressive craniotomy, and blunt trauma to the neck [Steinborn et al., 2010; Marino et al., 2006; Tawil et al., 2008; Tian et al., 2008; Latronico and Marino, 2009]. Strangulation is not a common cause of cerebral infarction in abused infants [Gilles and Nelson, 1998; Drack et al., 1999].
Diffuse axonal injury
Diffuse axonal shear injury is an important determinant of clinical status and outcome from nonmissile head injury [Adams et al., 1982; Hammoud and Wasserman, 2002; Strich, 1961]. It is a common feature of severe traumatic brain injury in older children and adults; its frequency is not established in infants, and there is conflicting evidence about its importance in inflicted head injury [Tokutomi et al., 1997; Tong et al., 2004]. Diffuse axonal shear injury has been described in infants younger than 5 months of age, but it is not commonly diagnosed at necropsy in abused infants [Vowles et al., 1987].
Major angular deceleration forces are required. The most common accidental cause is a high-speed motor vehicle crash or a pedestrian struck by a high-velocity vehicle. It occurs at the moment of injury and, when severe, is associated with loss of consciousness at the moment of impact [Adams et al., 1977; Gennarelli, 1993]. Imaging findings of shear injuries include punctate hemorrhages, particularly at the gray–white matter, and tearing of the corpus callosum (see Figure 75-8) [Demaerel et al., 2002; Mendelsohn et al., 1992].
Cerebral contusions
An infant younger than 8 months is much less likely to develop cortical contusions in coup or contrecoup locations than are older children and adults [Lindenberg and Freytag, 1969]. The incidence of contrecoup injuries rises rapidly from later infancy to 3 years of age, when it begins to approximate that of the adults [Marin-Padilla et al., 2002; Digraham et al., 1989; McLaurine and Tutor, 1961].
White-Matter Contusional Tears
On occasion, young infants present with white-matter tears, also referred to as white-matter contusional tears or gliding contusions [Lindenberg and Freytag, 1969; Calder et al., 1984; Jaspan et al., 1992; Vowles et al., 1987]. Contusional tears occur where shear strain is greatest: namely, deep to the gray matter–white matter junction (Figure 75-12 and Figure 75-13) [Lindenberg and Freytag, 1969; Calder et al., 1984; Jaspan et al., 1992; Gentry et al., 1988]. From 1 to 3 cm in length, they are most often located in the frontal and temporal lobes, although they may be seen throughout the brain [Lindenberg and Freytag, 1969].
These tears are considerably larger than the tissue tear hemorrhages in the adult brain after closed head injury that are punctate and are found most often in the periventricular white matter, corpus callosum, and brainstem [Wilberger et al., 1990]. When white-matter tears are filled with fresh blood, they may be mistaken for parenchymal hemorrhages (rare in childhood inflicted or unintentional head injury). In an acutely swollen brain, white-matter contusional tears may be difficult to visualize on CT or MRI [Gentry et al., 1988]. They are most easily visualized on follow-up imaging studies, in which they appear as slit-like or oval lesions within the cerebral parenchyma (see Figure 75-12).
Ocular Pathology
Retinal Hemorrhages
A range of injuries to the globe, optic nerve, and surrounding tissues has been reported after inflicted injury, including retinoschisis, retinal folds, retinal detachment, periorbital edema and ecchymosis, subconjunctival hemorrhages, hyphema, and cataracts [Vinchon et al., 2009; Buys et al., 1992