Neonatal and Pediatric Respiratory Care
After reading this chapter you will be able to:
Describe the correct approach to assessment of the fetus and newborn infant.
Discuss the use of oxygen therapy, bronchial hygiene therapy, aerosol drug therapy, airway management, and resuscitation approaches in the care of infants and children.
Describe the correct approach to assessment of the pediatric patient.
Discuss the use of continuous positive airway pressure and the basics of mechanical ventilation including high-frequency ventilation in the care of infants and children.
List clinical situations where nitric oxide and extracorporeal life support are used, and discuss the basic application of each.
Caring for infants and children is one of the most challenging and rewarding aspects of respiratory care. Competent clinical practice in this area requires knowledge of the many pathophysiologic differences among infants, children, and adults. Understanding the unique pathophysiology involved in neonatal and pediatric respiratory disorders (see Chapter 31) can assist the respiratory therapist (RT) in providing quality care to infants and children. A thorough understanding of how the respiratory system develops in the fetus is the first step toward acquiring the specialized knowledge needed to practice neonatal respiratory care (see Chapter 8). This chapter begins with an overview of neonatal and pediatric patient assessment and then describes respiratory care modalities used to treat these patients.
Assessment of the Newborn
Maternal Factors
Maternal risk factors include many medical, physical, and social conditions. Maternal health and individual physiology, pregnancy complications, and maternal behaviors affect the health of the fetus. Any condition that causes an interference with placental blood flow or the transfer of oxygen (O2) to the fetus can result in an adverse outcome. The clinician must be prepared for the possibility of resuscitation at delivery. This possibility is best anticipated by identifying risk factors that relate to neonatal compromise. Table 48-1 lists maternal risks and related outcomes of which the team preparing to receive the infant should be aware when the infant is delivered.
TABLE 48-1
Maternal Condition and Neonatal Outcomes
Maternal Condition | Fetal or Neonatal Outcome |
Previous pregnancy complication | Same outcome as previous fetus |
Diabetes mellitus | LGA, congenital malformations, RDS, hypoglycemia |
Pregnancy-induced hypertension | Prematurity, SGA (preeclampsia) |
Maternal age <17 years | Low birth weight, prematurity |
Maternal age >35 years | Prematurity, chromosomal defects |
Placenta previa | Prematurity, bleeding, SGA |
Abruptio placentae | Fetal asphyxia, bleeding |
Alcohol consumption | SGA, CNS dysfunction, mental retardation, facial dysmorphology |
Smoking | SGA, prematurity, mental retardation, SIDS |
Drug use | Placental abruption, IUGR, prematurity, CNS abnormalities, withdrawal disorders |
Fetal Assessment
Amniocentesis involves direct sampling and quantitative assessment of amniotic fluid. Amniotic fluid may be inspected for meconium (fetal bowel contents) or blood. In addition, sloughed fetal cells can be analyzed for genetic normality. Lung maturation can be assessed with amniocentesis. The lecithin-to-sphingomyelin ratio (L : S ratio) involves measurement of two phospholipids, lethicin and sphingomyelin, synthesized by the fetus in utero. As shown in Figure 48-1, the L : S ratio increases with increasing gestational age. At approximately 34 to 35 weeks’ gestation, this ratio abruptly increases to greater than 2 : 1. An L : S ratio greater than 2 : 1 indicates stable surfactant production and mature lungs. Phosphatidylglycerol is another lipid found in the amniotic fluid that is used to assess fetal lung maturity. Phosphatidylglycerol first appears at approximately 35 to 36 weeks’ gestation. If phosphatidylglycerol is more than 1% of the total phospholipids, the risk of respiratory distress syndrome is less than 1%.
Figure 48-2 illustrates the three common patterns of early decelerations, late decelerations, and variable decelerations. Early decelerations occur when the fetal heart rate decreases in the beginning of a contraction. This type of deceleration is benign and in most cases is caused by a vagal response related to compression of the fetal head in the birth canal. A late deceleration occurs when the heart rate decreases 10 to 30 seconds after the onset of contractions. A late deceleration pattern indicates impaired maternal-placental blood flow, or uteroplacental insufficiency. With variable decelerations, there is no clear relationship between contractions and heart rate. This pattern is the most common of the three and probably related to umbilical cord compression. Short periods of cord compression are generally benign, but prolonged periods of compression result in impaired umbilical blood flow and can lead to fetal distress. Fetal heart rate variability is the beat-to-beat variation in rate that occurs because of normal sympathetic or parasympathetic influences. A completely monotonous heart rate tracing may be indicative of fetal asphyxia. Fetal heart rate reactivity is the ability of the fetal heart rate to increase in response to movement or external stimuli. A healthy fetus has two accelerations within a 20-minute period.
Evaluation of the Newborn
Meconium is the medical term for the infant’s first stools. It is a sticky green-black substance that if inhaled by the infant can cause significant respiratory problems. It is most likely present in a term or postterm newborn. Term infants delivered without evidence of meconium who are crying or breathing and have good tone should not routinely be separated from the mother. They should be dried, covered, and given to the mother and observed for breathing, activity, and color. If meconium is present and the infant is vigorous, pharyngeal suctioning with a bulb suction is appropriate. Simultaneously the infant should be dried and placed under a warmer and assessed for signs of respiratory distress. See later section on Respiratory Assessment of the Infant. If meconium is present in a nonvigorous infant, stimulation should be avoided.
The most important and effective action in neonatal resuscitation is effective ventilation. Recommendations from the American Academy of Pediatrics are to attach a pulse oximeter to the infant, begin resuscitation efforts using room air, and assess carefully the amount of O2 needed.1 Effective PPV usually results in rapid improvement of heart rate. Initial ventilating pressures of 30 to 40 cm H2O may be necessary to achieve noticeable chest movement, particularly in a preterm newborn with surfactant deficiency. Continuous assessment of the lowest pressure needed to observe the chest rise is essential throughout the resuscitation. After application of PPV for 30 seconds, the heart rate is reassessed. If the heart rate is less than 60 beats/min, chest compressions are begun, and PPV is maintained. If the heart rate remains less than 60 beats/min after adequate ventilation with 100% O2 and chest compressions for 30 seconds, appropriate medications are given. As soon as the heart rate is noted to be greater than 100 beats/min, compressions are discontinued. If spontaneous breathing is present, PPV may be gradually reduced and then discontinued. If spontaneous breathing remains inadequate or if heart rate remains less than 100 beats/min, assisted ventilation is continued via bag-mask or endotracheal tube. Figure 48-3 outlines a newborn resuscitation algorithm and includes the targeted saturation levels for the first 10 minutes of life.
Apgar Score
An Apgar score is assigned at 1 minute and 5 minutes of life. The Apgar score is an objective scoring system used to evaluate a newborn rapidly. As shown in Table 48-2, the score has five components: heart rate, respiratory effort, muscle tone, reflex irritability, and skin color. Each component is rated according to standard definitions, resulting in a composite assessment score. Generally, infants scoring 7 or higher at 1 minute are responding normally. An infant with a score of 7 may require supportive care, such as O2 or stimulation to breathe. Infants with a 1-minute Apgar score of 6 or lower may require more aggressive support.
TABLE 48-2
Apgar Scoring System for Newborn Assessment
Score | |||
Sign | 0 | 1 | 2 |
Heart rate | Absent | <100/min | >100/min |
Respirations | Absent | Slow, irregular | Good, crying |
Muscle tone | Limp | Some flexion | Active motion |
Reflex irritability (catheter in nares, tactile stimulation) | No response | Grimace | Cough, sneeze, cry |
Color | Blue or pale | Pink body with completely blue extremities | Pink |
From Koff PB, Eitzman DV, Neu J: Neonatal and pediatric respiratory care, ed 2, St Louis, 1993, Mosby.
Assessment of Gestational Age
Gestational age assessment and assessment of relationship of weight to gestational age are performed shortly after delivery. Determination of gestational age involves assessment of multiple physical characteristics and neurologic signs. Two common systems are used to determine gestational age: the Dubowitz scales and the Ballard scales. The Dubowitz scales involve assessment of 11 physical and 10 neurologic signs.2 Physical criteria include assessment of skin texture, skin color, and genitalia. Neurologic criteria include posture and arm and leg recoil. The Ballard scales are a simplified version of the Dubowitz scales and include six physical and six neurologic signs as illustrated in Figure 48-4. Soon after delivery, the newborn is stabilized and weighed, followed by determination of gestational age. Infants born between 38 weeks and 42 weeks are considered term gestation. Infants born before 38 weeks are preterm. Infants born after 42 weeks are postterm.
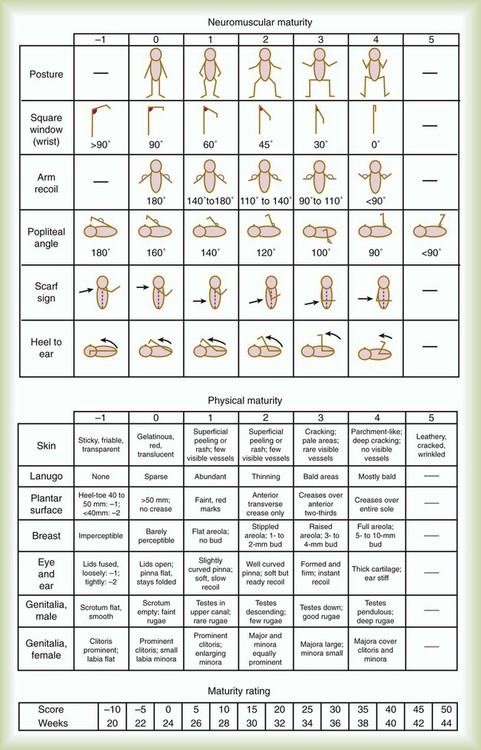
All newborns weighing less than 2500 g are considered low birth weight. Newborns weighing less than 1500 g are considered very low birth weight (VLBW). Newborns weighing less than 1000 g are considered extremely low birth weight (ELBW). A newborn with a weight that is either too large or too small or who has been born preterm or postterm has a higher risk of morbidity and mortality. As shown in Figure 48-5, by plotting the infant’s gestational age against weight, the newborn’s relative developmental status can be classified. Infants whose weight falls between the 10th and 90th percentiles are appropriate for gestational age (AGA). Infants whose weight is above the 90th percentile are large for gestational age (LGA). Infants whose weight is below the 10th percentile are small for gestational age (SGA).
Respiratory Assessment of the Infant
Physical Assessment
Normal infant heart rates range from 100 to 160 beats/min. Heart rate can be assessed by auscultation of the apical pulse, normally located at the fifth intercostal space, midclavicular line. Alternatively, the brachial and femoral pulses may be used. Weak pulses indicate hypotension, shock, or vasoconstriction. Bounding peripheral pulses occur with major left-to-right shunting through a patent ductus arteriosus (PDA).3A strong brachial pulse in the presence of a weak femoral pulse suggests either PDA or coarctation of the aorta. Table 48-3 lists normal ranges of blood pressure for neonates of different sizes.
TABLE 48-3
Normal Neonatal Blood Pressures
Weight (g) | Systolic (mm Hg) | Diastolic (mm Hg) |
750 | 35-45 | 14-34 |
1000 | 39-59 | 16-36 |
1500 | 40-61 | 19-39 |
3000 | 51-72 | 27-46 |
From Whitaker K: Comprehensive perinatal and pediatric respiratory care, ed 3, Albany, NY, 2001, Delmar.
Chest examination in an infant is more difficult to perform and interpret than in an adult because of the small chest size and the ease of sound transmission through the infant chest. Thorough observation of the infant greatly enhances the assessment data obtained. Infants in respiratory distress typically exhibit one or more key physical signs: nasal flaring, cyanosis, expiratory grunting, tachypnea, retractions, and paradoxical breathing. Nasal flaring is seen as dilation of the ala nasi on inspiration. The extent of flaring varies according to facial structure of the infant. Nasal flaring coincides with an increase in work of breathing. In concept, nasal flaring decreases the resistance to airflow. It also may help stabilize the upper airway by minimizing negative pharyngeal pressure during inspiration.4 Cyanosis may be absent in infants with anemia, even when arterial partial pressure of oxygen (PaO2) levels are decreased. In addition, infants with elevated fetal hemoglobin levels may not become cyanotic until PaO2 decreases to less than 30 mm Hg. Hyperbilirubinemia, common among newborns, may mask cyanosis. Grunting occurs when infants exhale against a partially closed glottis. By increasing airway pressure during expiration, grunting helps prevent airway closure and alveolar collapse. Grunting is most common in infants with respiratory distress syndrome, but it is also seen in other respiratory disorders associated with alveolar collapse. Figure 48-6 illustrates the Silverman score, which is a system of grading severity of lung disease.
Surfactant
Surfactant production begins around the 24th week of gestation and continues through gestation. Surfactant contributes to the stability of the alveolar sacs by reducing the surface tension of the fluids that coat the alveoli. Surfactant deficiency places an infant at increased risk for respiratory distress. By about 34 weeks’ gestation, most infants have produced enough surfactant to keep the alveoli from collapsing. There are two specific approaches to preventing and treating surfactant deficiency. Surfactant deficiency is due to lung immaturity. When a premature delivery is anticipated, steroids are given to the mother to help promote lung maturation. In addition, infants born before 35 weeks’ gestation, especially infants born very prematurely (<30 weeks), should be assessed for the need to receive exogenous surfactant. The need for surfactant is determined by assessing the infant’s lung volume on chest x-ray, evaluating the inspired O2 concentration to maintain O2 saturations greater than approximately 88%, and clinically assessing the infant’s work of breathing. Once surfactant deficiency is determined, administering exogenous surfactant as soon as possible has been found to be most beneficial.5
Blood Gas and Pulse Oximetry Analysis
Blood gas analysis is helpful in assessing respiratory distress in an infant. Many noninvasive techniques, such as transcutaneous partial pressure of oxygen (PtcO2), transcutaneous partial pressure of carbon dioxide (PtcCO2), end tidal carbon dioxide (CO2), and pulse oximetry (SpO2), are used to obtain comparable data, although blood gas analysis is more precise when results are critical. An infant blood gas sample can be obtained from an artery or capillary. Chapter 18 summarizes the advantages, disadvantages, and complications of these sampling methods. Care must be taken in assessing the results of capillary sampling. Capillary blood gases provide only information regarding ventilation and acid-base status, and accuracy is highly dependent on technique.6 Normal values for infant blood gases are listed in Table 48-4.
TABLE 48-4
Age-Related Values Commonly Reported for Normal Blood Gases
Normal Preterm Infants (at 1-5 Hours) | Normal Term Infants (at 5 Hours) | Normal Preterm Infants (at 5 Days) | Children, Adolescents, and Adults | |
pH (range) | 7.33 (7.29-7.37) | 7.34 (7.31-7.37) | 7.38 (7.34-7.42) | 7.40 (7.35-7.45) |
PCO2 (range) | 47 (39-56) | 35 (32-39) | 36 (32-41) | 40 (35-45) |
PO2 (range) | 60 (52-68) | 74 (62-86) | 76 (62-92) | 95 (85-100) |
HCO3− range | 25 (22-23) | 19 (18-21) | 21 (19-23) | 24 (22-26) |
BE range | −4 (−5 to −2.2) | −5 9 (−6 to −2) | −3 (−5.8 to −1.2) | 0 (−2 to +2) |
BE, Base excess; HCO3−, bicarbonate.
Modified from Orzalesi MM, Mendicini M, Bucci G, et al: Arterial oxygen studies in premature newborns with and without mild respiratory disorders. Arch Dis Child 42:174, 1967. From Koff PB, Eitzman DV, Neu J: Neonatal and pediatric respiratory care, ed 2, St Louis, 1993, Mosby.
Respiratory Assessment of the Pediatric Patient
Normal breathing in children is evidenced by quiet inspiration and passive expiration at an age-appropriate rate. Respiratory rates are rapid in neonates and decrease in toddlers and older children. Table 48-5 lists normal respiratory rates. The initial assessment of a pediatric patient starts with evaluating airway patency. Normal heart rates are higher in younger children and decrease with age. In assessing a pediatric patient, establishing if the airway is patent or has any obstructive component is essential. Signs that suggest upper airway obstruction include increased inspiratory effort with retractions or inspiratory efforts with no airway or breath sounds.
TABLE 48-5
Normal Respiratory and Heart Rates by Age
Age | Breaths/Minute | Heart Rates |
Infants (<1 yr) | 30-60 | 90-120 |
Toddler (1-3 yr) | 24-40 | 80-100 |
Preschooler (4-5 yr) | 22-34 | 70-90 |
School age (6-12 yr) | 18-30 | 70-90 |
Adolescent (13-18 yr) | 16-22 | 60-80 |
Respiratory Care
Oxygen Therapy
Goals and Indications
Lower saturation levels are targeted in infants less than 32 weeks’ gestation. There is evidence that exposure to supplemental O2 in a premature infant is a risk factor for the development of retinopathy of prematurity (ROP). ROP is caused by an abnormal vascularization of the retina, which in the most severe cases leads to retinal detachment. Preterm neonates weighing less than 1500 g are most susceptible. Hyperoxia is not the only factor associated with ROP, but close monitoring and adjusting of O2 therapy to avoid hyperoxia is crucial to decrease the risk of ROP. Specific saturation goals for this age group should be established, and O2 should be adjusted to maintain the intended target. Avoiding very high or very low saturation levels is critical. Adjusting the delivered O2 concentration by small increments avoids large swings in saturation levels.7–11
Methods of Administration
The effectiveness of O2 devices depends on the performance characteristics of the device (delivered FiO2, flow rate, relative humidity), the interface of the device, and the tolerance of the patient for using the device. Children are often frightened and combative, making it impractical to use some O2 administration devices. Selection of an O2 device must be based on the degree of hypoxemia and the emotional and physical needs of the child and family. O2 can be administered to infants and children by mask, cannula, high-flow nasal cannulas, or oxyhood. Table 48-6 compares the advantages and disadvantages of standard O2 delivery methods.
TABLE 48-6
Device | Age | FDO2 | Advantages | Disadvantages |
Air entrainment mask | ≥3 yr | High flow; 0.24-1 | Precise FiO2; good for transport; ease of application | Low relative humidity; pressure necrosis to face; difficult to fit and maintain on active child, not recommended for infants; risk of aspiration |
Nasal cannula | Premature infants to adult | Low flow; 25 ml/min–6.0 L/min | Tolerated well by all ages | Inaccurate FiO2; low relative humidity; excessive flows may cause inadvertent CPAP in infants; precise FiO2 may be achieved with O2 blender |
Incubator | Newborns ≤28 days | <0.40 FiO2, combine use with cannula or hood for precise FiO2 | Low FiO2 for stable infants; neutral thermal environment for premature infants | Varying FiO2; long stabilization time; limits access to child for patient care |
Oxyhood | Premature infants to ≤6 mo | 0.21-1 FiO2 with O2 blender maintained at 30° C to 34° C | Warmed and humidified gas at stable FiO2 during routine patient care | Overheating may cause apnea and dehydration; underheating may cause O2 consumption; inadequate flow causes CO2 buildup; noise produced by humidification device may cause hearing loss |
Mist tent | Infants to toddlers | High flow; 0.21-0.40 FiO2 | Allows child movement, high humidity, cool temperatures | Isolation of child from family; wet bedding and clothes; difficult to maintain stable FiO2; risk of cross-contamination; limits patient care |
Secretion Clearance Techniques
Secretion clearance techniques that can be applied to infants and children include chest physiotherapy, positive expiratory pressure therapy, autogenic drainage, flutter therapy, and mechanical insufflation-exsufflation.12,13 Secretion clearance techniques are considered when accumulated secretions impair pulmonary function and an infiltrate is visible on a chest radiograph. Secretion retention is common in children who have pneumonia, bronchopulmonary dysplasia, cystic fibrosis, bronchiectasis, and some neuromuscular diseases. Figure 48-7 shows postural drainage and percussion positions for infants and children.
Methods
Infants and young children cannot cough on command. For this reason, secretions often must be removed by suctioning. For older children with excessive secretions, directed deep breathing and coughing may help improve pulmonary clearance. The use of mechanical insufflation-exsufflation in children with neuromuscular disease can be helpful in clearing secretions. Adjunctive therapy devices such as positive expiratory pressure, flutter, or intermittent percussive ventilation therapy have been effective in secretion clearance in patients with cystic fibrosis.14
Humidity and Aerosol Therapy
Humidity Therapy
In children with an intact upper airway, O2 therapy devices, such as low-flow nasal cannulas, do not routinely need to be humidified When the upper airway is bypassed by intubation, supplemental humidification must be provided using a heated humidifier. Humidification of inspired gases for infants and children receiving mechanical ventilation is commonly provided by a servo-controlled humidifier. Ideal features for these systems include the following: (1) low internal volume and constant water level to minimize compressed volume loss; (2) closed, continuous feed water supply to avoid contamination; (3) distal airway temperature sensor and high/low alarms. Common problems with humidifier systems include condensation in the tubing, inadequate humidification, and hazards associated with the heating coil.15,16 Using heated wire circuits can also reduce condensation in the circuit. Frequent evaluation of the humidification system is necessary to increase the potential of adequate humidity delivered to the airway. Inadequate humidification occurs in nonheated circuits when the humidifier temperature probe is placed too far upstream from the airway connector. Variable humidification problems occur when ventilator circuits pass through an environment and then into a warmed enclosure, such as an incubator or radiant warmer.
Aerosol Drug Therapy
Drug action in infants and children differs significantly from drug action in adults because of differences in physiology, which may include immature enzyme systems, immature receptors, and variable gastrointestinal absorption. Dosing may be imprecise, and systemic effects may be hard to predict. Table 48-7 lists aerosolized medications commonly used in children.
TABLE 48-7
Commonly Used Aerosolized Medications
Medication Name | Dosage Form | Usual Child Dose | Comments |
Bronchodilators | |||
Beta-2 Agonists | |||
Albuterol (Proventil, Ventolin) | MDI (90 mcg/puff) | 1-2 puffs MDI q 15 min to q 6 hr ± PRN | May be used 15 min before exercise to prevent exercise-induced bronchospasm; should be used as a rescue medication |
Nebs (0.5%, 5 mg/ml) | 0.01-0.05 ml/kg/dose (maximum 1 ml/dose) neb q 15 min to q 6 hr ± PRN | ||
Rotohaler (200 mcg caps) | 1-2 caps inhaled q 15 min to q 6 hr ± PRN | ||
Levalbuterol (Xopenex) | Nebs (0.63 mg/3 ml, 1.25 mg/3 ml) | 0.32-1.25 mg neb q 6-8 hr ± PRN | May still cause extrapulmonary side effects including tachycardia and hypokalemia |
Salmeterol (Serevent) | MDI (21 mcg/puff) | 2 puffs inhalation q 12 hr | Not to be used as a rescue medication; long-acting beta-2 agonist; QTC prolongation has occurred in overdose |
DPI-Diskus (50 mcg/inhalation) | 1 inhalation q 12 hr | ||
Nonselective Bronchodilator | |||
Racemic epinephrine (Vaponefrin) | Nebs (2.25%) | 0.25-0.5 ml neb q 1-4 hr ± PRN | If shortage occurs, may use L-epinephrine (1 : 1000) 2.5-5 ml neb q 1-4 hr ± PRN |
Anticholinergic | |||
Ipratropium (Atrovent) | MDI (18 mcg/puff) | 2 puffs inhalation q 4-6 hr ± PRN | MDI is contraindicated in patients with peanut allergy; for neonates, use 25 mcg/kg/dose neb tid; may cause mydriasis if aerosolized drug gets into the eye |
Nebs (0.02%) | 0.25-0.5 mg neb q 4-6 hr ± PRN | ||
Antiinflammatory Agents | |||
Corticosteroids | |||
Beclomethasone (Beclovent, Vanceril) | MDI (42 mcg/puff) | 1-2 puffs inhalation qid or 2-4 puffs inhalation bid | Start at lower end of dosing range if patient not previously on steroids; titrate to lowest dose that is effective; always rinse mouth after each treatment |
MDI double strength (84 mcg/puff) | 2 puffs inhalation bid | ||
Budesonide (Pulmicort) | DPI-Turbuhaler (200 mcg/inhalation) | 1-2 puffs inhalation bid | May take several weeks to see benefit; not to be used as a rescue medication |
Nebs-Respules (0.25 mg/2 ml, 0.5 mg/2 ml) | 0.25-0.5 mg neb bid or 0.5-1 mg neb qd | ||
Flunisolide (Aerobid, Aerobid-M) | MDI (250 mcg/puff) | 2-3 puffs inhalation bid | |
Fluticasone (Flovent) | MDI (44 mcg/puff, 110 mcg/puff, 220 mcg/puff) | 2 puffs inhalation bid (maximum 880 mcg/day) | |
Rotadisk (50 mcg/blister) | 50-100 mcg inhalation bid | ||
Triamcinolone (Azmacort) | MDI (100 mcg/puff) | 1-2 puffs inhal qid | |
Mast Cell Stabilizers | |||
Cromolyn (Intal) | MDI (800 mcg/puff) | 2 puffs inhalation qid | May take several weeks to see benefit; not to be used as a rescue medication |
Nebs (20 mg/2 ml) | 20 mg neb qid | ||
Nedocromil (Tilade) | MDI (1.75 mg/puff) | 2 puffs inhalation qid | |
Mucolytics | |||
N-acetylcysteine (Mucomyst) | Nebs (20%, 200 mg/ml) | 3-5 ml neb qid | Consider pretreatment with albuterol 15 min before N-acetylcysteine secondary to bronchospasm |
Dornase alfa (Pulmozyme) | Nebs (2.5 mg/2.5 ml) | 2.5 mg neb qid-bid | May cause hemoptysis |
Antiinfectives | |||
Pentamidine (Pentam) | Nebs (300 mg) | 8 mg/kg/dose (maximum 300 mg/dose) neb q month | Used for PCP prophylaxis |
Ribavirin (Virazole) | Powder (6 g vial) | 2 g over 2 hr neb q 8 hr × 3-7 days or 6 g over 12-18 hr neb q 24 hr × 3-7 days | Used for RSV treatment; mutagenic, teratogenic |
Tobramycin (TOBI) | Nebs (300 mg/5 ml) | 300 mg neb q 12 hr | Used for pseudomonal infection of the lungs |
PCP, Pneumocystis jiroveci pneumonia; RSV, respiratory syncytial virus.
Small volume nebulizers (SVNs), MDIs, and dry powder inhalers (DPIs) can be used to deliver aerosolized drugs via mouthpiece or face mask to infants and children.17 Continuous aerosol drug therapy is also used for patients unresponsive to intermittent SVN treatments. Aerosol drug administration to intubated infants and children is challenging because of the decreased deposition from baffling of small endotracheal tubes in these patients, which prevents approximately 90% of the drug from entering the lungs, regardless of delivery system. In addition, careful adjustments must be made to the ventilator so that nebulizer flows do not alter delivered VT and inspiratory pressure and interfere with triggering efforts.18
Airway Management
Airway management methods in infants and children are unique because of the anatomic differences between neonates and adults. Specifically, equipment and technique must be tailored to each child according to his or her size, weight, and postpartum age. Masks, oral airways, suction catheters, laryngoscope blades, and endotracheal tubes in a wide selection of infant and child sizes are needed to account for variations in patient age and weight. Table 48-8 provides recommendations regarding endotracheal tube and suction catheter sizes for infants and children.
TABLE 48-8
Endotracheal Tube and Suction Catheter Sizes for Infants and Children
Age or Weight | Endotracheal Tube ID (mm) | Oral Tube Length (cm) | Nasal Tube Length (cm) | Suction Catheter (F) |
Newborn | ||||
<1000 g | 2.5 | 9-11 | 11-12 | 6 |
1000-2000 g | 3 | 9-11 | 11-12 | 6 |
2000-3000 g | 3.5 | 10-12 | 12-14 | 6 |
>3000 g | 4 | 11-12 | 13-14 | 8 |
Children | ||||
6 mo | 3-4 | 11-12 | 12-14 | 6-8 |
18 mo | 3.5-4.5 | 11-13 | 13-15 | 8 |
2 yr | 4-5 | 12-14 | 14-16 | 8-10 |
3-5 yr | 4.5-5.5 | 12-15 | 14-17 | 8-10 |
6 yr | 5.5-6 | 14-16 | 16-18 | 10 |
8 yr | 6-6.5 | 15-17 | 17-19 | 10-12 |
12 yr | 6-7 | 17-19 | 19-21 | 10-12 |
16 yr | 6.5-7.5 | 19-21 | 21-23 | 10-12 |
Estimating formula for tube internal diameter (ID) in mm:
Intubation
Endotracheal intubation is a generally safe method of airway management in infants and children, even when used for extended periods.19,20 Complications and hazards associated with intubation in these age groups are listed in Box 48-1. The infant’s age or weight can be used to estimate proper endotracheal tube size and depth of insertion. If the tube is too small in diameter, a leak may result, decreasing delivered minute ventilation. Small endotracheal tubes have high inspiratory resistance, increasing the spontaneous work of breathing for the child. An inappropriately large endotracheal tube can cause mucosal and laryngeal damage that is evident after extubation, resulting in upper airway obstruction.21
Most neonatal and pediatric endotracheal tubes are uncuffed. The narrowest point of the airway in an infant and small child is the cricoid cartilage. When an appropriately sized uncuffed tube is positioned in the airway, the fit of the tube in the airway “seals” the airway enough so that adequate ventilation can usually be maintained. Cuffed endotracheal tubes are an option if a large leak persists around the tube and stable ventilation cannot be maintained. Similar to with adults when a cuffed tube is used, careful attention to the pressure of the cuff on the tracheal wall is essential. Because the tongue is large and the epiglottis is anatomically high in infants and small children, practitioners generally find the Miller (straight) laryngoscope blade best for intubation. Infant endotracheal tubes are small and can be easily kinked or obstructed. In addition, slight changes in the position of the endotracheal tube in movement can result in bronchial intubation.22
Once a tube is inserted, immediate securing of the tube to the infant’s face and ongoing evaluation of the security of the tube are essential. Proper head positioning and avoidance of cumbersome connecting apparatus help reduce the potential of accidental extubation. Estimates of the distance the tube should be inserted into the airway based on patient weight are provided in Table 48-9. Further confirmation of correct tube position should be evaluated with a chest x-ray. Noting the infant’s head position when the chest x-ray is obtained is helpful in assessing appropriate tube position in the airway. Slight changes in head position can result in the tube position sitting too high or too low in the airway. In very small infants, light flexion of the head can move the tube into the right main stem bronchus.
TABLE 48-9
Approximate Distance from Infant’s Lip to End of Inserted Oral Endotracheal Tube
Weight (kg) | Mark at Lip (cm) |
<1 | 6.5 |
1 | 7 |
2 | 8 |
3 | 9 |
4 | 10 |
Breath sounds may be of limited value in infants and small children for evaluation of tube position. Portable end tidal CO2 monitoring devices may be used to help assess the tube in the airway, although these should be used as only additional assessment tools with recognition of their limitations. Factors associated with accidental extubation of infants include tension on the tube from the ventilator circuit, patient agitation, suctioning, head turning, chest physiotherapy, too short a tube distance between lip and adapter, moving the patient during procedures, and inadequately taped endotracheal tube.23
Laryngeal mask airways (LMAs) are available as an alternative to intubation. LMAs are typically used in children during periods when a short-term airway is indicated, such as during some surgical procedures or when endotracheal intubation cannot be accomplished and an airway needs to be established. Table 48-10 outlines appropriate sizes and maximum cuff volumes of LMAs for varying weights.
TABLE 48-10
Appropriate Sizes and Maximum Cuff Volumes of Laryngeal Mask Airways for Varying Weights
LMA Size | Patient Weight (kg) | Maximum Cuff Volume |
1 | 1-5 | 4 |
1.5 | 5-10 | 7 |
2 | 10-20 | 10 |
2.5 | 20-30 | 14 |
3 | 30-40 | 20 |
Suctioning Intubated Pediatric Patients
The goal of suctioning is to remove secretions from large airways and stimulate a cough. Although suctioning can be beneficial, significant risks are associated with the procedure, including lung derecruitment, hypoxia, hypertension, increased intracranial pressure, tracheal trauma, and infection. Absolute and relative indications for suctioning are listed in Box 48-2.
Suctioning
Nasopharyngeal and tracheal suctioning helps minimize aspiration, prevents endotracheal tube occlusion, and reduces airway resistance in infants and children.24 Suctioning is a hazardous procedure, and complications can occur. Box 48-3 lists the common complications and hazards associated with tracheal suctioning of infants and children. Tracheal suctioning of preterm infants and neonates should be performed only when clinical signs indicate a need.25,26
Oral and pharyngeal suctioning of infants can be done with a bulb syringe. A DeLee trap or a mechanical vacuum source with catheter may be used for nasopharyngeal and nasotracheal suctioning of neonates. Equipment for suctioning larger infants and children is similar to the equipment used with adults with modifications in vacuum pressure and catheter size. Recommended suction pressures for neonates range from approximately −60 to −80 mm Hg. With large infants and children, pressures in the range of −80 to −100 mm Hg are generally safe and effective. Catheter sizes are chosen according to the age of the patient and the size of the tracheal airway (see Table 48-8). Other techniques for averting hypoxemia include use of endotracheal tube adapters that allow preoxygenation and suctioning without disconnection of the ventilator and use of closed tracheal suction systems.27,28
Continuous Positive Airway Pressure
Spontaneous breathing can be supported with continuous positive airway pressure (CPAP), a breathing mode that maintains a constant pressure above baseline throughout inspiration and expiration. CPAP maintains inspiratory and expiratory pressures above ambient, which improves functional residual capacity (FRC) and static lung compliance.29 It is essential that the patient is able to maintain adequate minute volume while breathing spontaneously because ventilatory support is not provided.
CPAP is indicated when arterial oxygenation is inadequate despite elevated FiO2. This condition is usually accompanied by certain signs of respiratory distress. CPAP is commonly used when PaO2 is less than 50 mm Hg while the infant is breathing FiO2 of 0.60 or greater, provided that the PaCO2 is less than or equal to 50 mm Hg and the pH is greater than 7.25. The indications for CPAP are described in Box 48-4.
Methods of Administration
The application of CPAP is most commonly accomplished noninvasively. In preterm and term neonates, nasal prongs or nasopharyngeal tubes were traditionally used. However, the more recent introduction of improved interface devices has led to more consistent CPAP delivery and better patient comfort (Figure 48-8). These interfaces include nasal masks and soft, pliable nasal cannulas that provide a comfortable interface without applying excessive pressure to maintain a tight fitting seal. The American Association for Respiratory Care (AARC) has published Clinical Practice Guideline: Application of CPAP to Neonates via Nasal Prongs, Nasopharyngeal Tube, or Nasal Mask. Excerpts from this guideline appear in Clinical Practice Guideline 48-1.
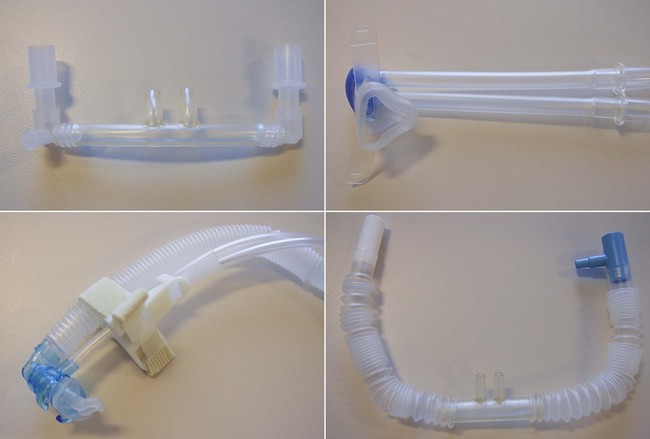
High-Flow Nasal Cannula
Supplemental O2 administration by nasal cannula is the most comfortable and simplest means of providing O2 for infants and children. Evidence in preterm and term neonates indicates that using a nasal cannula at flow rates of 2 to 8 L/min may be as effective as and is easier to apply than a nasal CPAP system.30,31
Specially designed humidification systems have been developed and allow the use of nasal cannulas at flow rates of 2 to 30 L/min.32 These devices maximize humidification and minimize condensation accumulating in the small diameter supply tubing. High-flow nasal cannula systems have been used successfully in neonates for the same indications that CPAP has been used. Instead of titrating levels of CPAP, the flow rate is incrementally adjusted. However, the amount of positive pressure that the high-flow nasal cannula potentially produces cannot be measured, and inadvertent high levels may occur, particularly if the nasal cannula fits snugly in the nares.33–35
Mechanical Ventilation
Early attempts to provide assisted ventilation to infants and children were largely derived from the experiences gained in adults, including the type of ventilators used and the associated techniques. Recognition of the physiologic differences of neonates and children led to further advances in ventilator design and modes and a wider range of capabilities. Although the classic “infant ventilator” is still widely used, modern microprocessor ventilators offer an ever-evolving array of options capable of supporting the full range of patient sizes and physiologic conditions.36 RTs caring for infants and children need to be familiar with their physiologic differences to select and modify the appropriate ventilator strategy.37–39
Goals of Mechanical Ventilation
The basic goals of mechanical ventilation are to improve O2 delivery to meet metabolic demand and eliminate CO2, while reducing the work of breathing.38,39 The basic aim of assisted ventilation is to meet the goals while minimizing the associated deleterious effects. One approach to mechanical ventilation begins with the selection of an appropriate breath type, either pressure-controlled or volume-controlled, and a mode that best meets the physiologic needs of the patient’s condition.40 Box 48-5 lists the indications for mechanical ventilation in infants and children.
Modes of Ventilation and Breath Delivery Types
Historically, the most common mode of ventilation used in neonates and children was intermittent mandatory ventilation. Because early infant ventilators were unable to respond to the small triggering efforts of these patients, mandatory timed breaths were superimposed over a continuous flow of gas. These asynchronous, mandatory breaths provided most of the ventilation, while the patient was allowed to breathe spontaneously from the continuous gas source. Eventually, technologic improvements resulted in triggering devices that provided synchronization of the mandatory breaths with patient effort (synchronized intermittent mandatory ventilation [SIMV]) followed by the ability to provide assist control (A/C) and pressure support ventilation (PSV). Despite evidence that SIMV is more likely to result in patient-ventilator asynchrony,41,42 most neonatal and pediatric patients are managed by using one of these three modes or a combination (i.e., SIMV + PSV). The most common triggering device for infant ventilators is a pneumotachygraph placed in the ventilator circuit, often proximal to the airway, which in many cases also serves as a monitoring device. The pneumotachygraph allows for the integration of a flow signal, which can be displayed as inhaled and exhaled VT and minute ventilation. Figure 48-9 displays graphic representations of A/C, SIMV, and PSV.
In almost all cases of neonatal ventilation, the mechanical breaths delivered during SIMV and A/C are time-cycled, pressure-limited breaths.43 Inspiration is initiated by patient effort or as a result of the set respiratory rate (whichever comes first). Based on the available flow—continuous, demand, or both—the set inspiratory pressure is reached early in the inspiratory phase and maintained throughout the remainder of the inspiratory time, after which the ventilator cycles to expiration. Most current-generation ventilators are capable of providing volume-targeted, pressure-limited ventilation, often referred to as pressure-regulated volume control or volume guarantee. In this dual mode of ventilation, the inspiratory VT is compared with a preset target VT, and the inspiratory pressure on the next breath is adjusted up or down in an attempt to meet the target volume. True volume-controlled breaths are rarely used for ventilating neonatal patients. (See Chapter 42 for details.)
Ventilator Settings and Parameters
Tidal Volume
If the clinician chooses to deliver volume-controlled breaths, VT is set as a control variable. Every mechanical breath delivers an identical VT at either a preset inspiratory time or a preset flow rate. Set VT, inspiratory time, and flow all are interrelated. If VT is set at 300 ml, and flow rate is 30 L/min (0.5 L/sec), the inspiratory time is 0.6 second. See formulas in Box 48-6.
Regardless of whether the clinician chooses volume-controlled or pressure-controlled breaths, he or she must recognize that some of the VT is compressed in the circuit and not delivered to the patient; this is referred to as compressible volume loss. Most current-generation ventilators automatically compensate for compressible volume loss and adjust the delivered and displayed (monitored) VT accordingly. With older ventilators, during volume-controlled breaths, the clinician must calculate the compressible volume loss and increase the set VT to deliver the desired volume to the patient. During volume-controlled and pressure-controlled breaths, the calculated compressible volume loss must be subtracted from the ventilator displayed exhaled VT. See Box 48-7 for calculation of compressible volume loss.
To guide practitioners in providing quality care, the AARC has published Clinical Practice Guideline: Neonatal Time-Triggered, Pressure-Limited, Time-Cycled Mechanical Ventilation. Excerpts from this guideline appear in Clinical Practice Guideline 48-2.
Oxygen Concentration
The O2 concentration or FiO2 is kept as low as possible to avoid the risk of O2 toxicity. Although the precise mechanisms are not understood, the best approach is to maintain the lowest FiO2 possible. The immature lung is particularly susceptible to O2 toxicity, which can result in the development of bronchopulmonary dysplasia. In a preterm infant, FiO2 is titrated to a narrow SpO2 range (e.g., 88% to 94%) so that retinal damage (ROP), which is caused by elevated PO2, does not develop.11
Noninvasive Ventilation
Noninvasive ventilation (NIV), also known as noninvasive positive pressure ventilation, has become more popular recently in neonatal patients. In the past, use of NIV was limited by the lack of available interfaces; however, these are becoming more readily available. Figure 48-8 shows various interfaces that may be used for NIV (or CPAP) in neonates. More recent evidence supports the use of both synchronized and nonsynchronized NIV in the neonatal intensive care unit (ICU).46 It is hoped that future research will clarify which patients are most likely to benefit from this form of support.
NIV has been used extensively in patients of all ages, including pediatric patients.47–50 Indications may include short-term support of hypoxemic respiratory failure, such as that seen with pulmonary edema associated with left-sided heart failure; prevention of intubation; postextubation support; and long-term support of patients with neuromuscular disease. Some limitation of available interfaces persists, particularly in smaller patients; however, most patients can be fitted without too much difficulty. As with CPAP and other noninvasive interface devices, care must be taken to prevent or minimize patient injury owing to iatrogenic pressure ulcers from a tight or poorly fitted device.
NIV may be provided with simple, single-limb devices such as bilevel positive airway pressure generators, or sophisticated ICU ventilators. Care must be taken whenever a single-limb circuit is employed to provide sufficient PEEP in the system to prevent rebreathing of gases (see Chapter 46).
Monitoring Mechanical Ventilation
The RT should develop a systematic approach to monitoring the effects of mechanical ventilation. Components of a ventilator assessment should include an evaluation of the artificial airway, physical examination, assessment of patient-ventilator interaction,51 analysis of laboratory and radiographic data, adjunct ventilator monitoring, and a systematic ventilator safety assessment including alarm function and assessment of humidification. Alarms should be connected to a central monitoring system to alert appropriately clinicians away from the bedside of a change the patient’s condition.
Patient-Ventilator Interaction
The patient-ventilator interaction is the assessment used to determine the ease with which the patient can trigger the ventilator and is made by simultaneously observing the trigger indicator and the patient. Refinements in the trigger threshold may need to be made if there is a leak present or the work to trigger or initiate a breath is too great. The manner in which the breath is terminated is also assessed. Together, patient synchrony and comfort are determined. Patient-ventilator asynchrony occurs when the patient’s efforts to breathe are unmatched with the preselected ventilator support. Airway graphics are also helpful in identifying nuances and refining ventilator settings.52 Airway graphics routinely displayed are scalar waveforms of flow, airway pressure, and volume. Additionally, each of these parameters can be plotted against each other. Pressure-volume and flow-volume loops can be particularly helpful in assessing alterations in work of breathing, overdistention of the lung, and compliance.
Patient-Ventilator Periodic Assessment
A systematic patient-ventilator assessment should be conducted periodically.53 Prescribed ventilator settings are confirmed and documented along with verification of ventilator outputs. Measurements of mandatory and spontaneous VT values are made and expressed per the patient’s weight to determine if targets are being achieved. Alarms are set and tested and should minimally detect loss of pressure, high pressure, and patient disconnection.
Weaning from Mechanical Ventilation
Weaning or, more appropriately, liberation from mechanical ventilation is a topic that until more recently has received little attention in pediatric and neonatal patients. Clear guidelines for “assessment of readiness to extubate” and “spontaneous breathing trials” are standard practice in adults. However, this assessment has not yet become standard practice in pediatric and neonatal patients even though there are resources available to guide the pediatric/neonatal clinician in this area.54 Nevertheless, these patients should be assessed daily to determine their readiness for liberation from mechanical ventilation. Some general considerations for extubation are presented in Box 48-8; however, distinct differences exist among these various patients, and it is reasonable to develop an age-specific, multidisciplinary approach to this task. One alternative would be to begin with existing adult guidelines and modify them to meet the needs of pediatric and neonatal patients. Two sets of guidelines, one for pediatric patients and one for neonates, are presented in Boxes 48-9 and 48-10. (See Chapter 47 for details on weaning.)
High-Frequency Ventilation
High-frequency ventilation (HFV) is a form of invasive mechanical ventilation that uses small VT values (less than dead space) at rapid frequencies, sometimes greater than 900 breaths/min (15 Hz). The primary goal of HFV is to provide adequate ventilation and oxygenation, while limiting the incidence of lung injury. HFV has been used as a primary mode of ventilation and a rescue therapy for patients determined to be failing conventional mechanical ventilation. Although early studies showed a beneficial effect compared with conventional ventilation, there has been no demonstrated improvement in outcome compared with current lung protective strategies.55–61 HFV remains an acceptable mode of ventilation for patients of any age but should be used only by clinicians expert in its clinical application and knowledgeable about its physiologic effects.
Specialty Gases
Inhaled Nitric Oxide
Inhaled nitric oxide (INO) is a selective pulmonary vasodilator used to treat newborns who require mechanical ventilation for hypoxic respiratory failure.62,63 INO improves oxygenation and reduces the need for extracorporeal membrane oxygenation (ECMO), the more invasive and complication-prone alternative. The approved indications for INO are listed in Box 48-12. INO has also been studied in preterm infants with the aim to reduce the incidence of chronic lung disease. These clinical investigations showed a modest improvement in pulmonary outcomes, but other problems associated with prematurity, such as intracranial hemorrhage, were unchanged. At the present time, INO is not routinely used in the management of respiratory failure associated with prematurity.
INO is administered in conjunction with mechanical ventilation via a specially designed delivery and monitoring system that provides precision drug dosing and safety features (Figure 48-10). The recommended INO dose is 20 parts per million (ppm) with an optimal response achieved when lung inflation is maximized.64,65 When a response has been achieved and sustained, the INO dose is gradually reduced, typically by 50% each step, to a final dose of 1 ppm, at which point the drug is discontinued. During withdrawal of INO, FiO2 is increased to minimize any recurrence of pulmonary hypertension.66
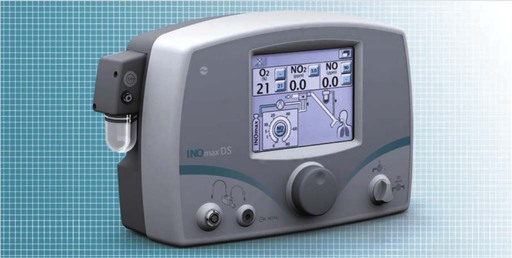
INO should be available in any hospital that has a level III intensive care nursery. INO should be an integral part of any high-risk transport team, and it is important that non-ECMO centers have a plan for treatment failure that takes into account the distance to an ECMO center.67 INO therapy has also been used for diagnosing and treating certain congenital heart diseases; although used in the management of ARDS, it seems to have less of a sustained effect in this setting.68 The AARC has published a clinical practice guideline on INO therapy. Excerpts from this guideline appear in Clinical Practice Guideline 48-3.69
Extracorporeal Membrane Oxygenation
Extracorporeal membrane oxygenation (ECMO) is a modified form of cardiopulmonary bypass used to provide relatively long-term pulmonary or cardiopulmonary life support when maximum medical interventions have failed.70 There are two types of ECMO support: venoarterial (VA), in which both heart and lung function is supported, and venovenous (VV), in which only the lungs are supported.
During VA ECMO, a cannula is inserted into the right internal jugular vein and advanced to the right atrium. Blood is drained from the right heart to a circuit where it is pumped through an artificial lung. The blood is oxygenated, and CO2 is removed by the artificial lung and returned to the patient through a cannula inserted most often into the carotid artery. The blood is warmed to body temperature before reinfusion to the patient (Figure 48-11). VV ECMO differs technically in that blood is drained and reinfused to the right side of the heart through a specially designed double-lumen cannula, one larger lumen for draining blood and a smaller lumen for reinfusion, or through two cannulas each inserted into a vein. The veins commonly cannulated are the right internal jugular vein and the right femoral vein. The blood traverses the same circuitry as with VA ECMO, but the arterial circulation is not invaded. When heart function is adequate, VV support can accomplish the goal of providing adequate oxygenation, while reducing the risk of lung injury from the ventilator. Box 48-13 outlines the advantages and disadvantages of VA and VV support. Arterial and venous access may also be achieved transthoracically (e.g., postoperative cardiac patients) or through femoral vessels (e.g., pediatric applications).
ECMO has been shown to improve survival in newborns with hypoxic respiratory failure associated with PPHN, meconium aspiration syndrome, sepsis, and, to a lesser extent, congenital diaphragmatic hernia. However, advances in newborn medicine, such as surfactant replacement therapy, approaches to mechanical ventilation, and INO, have greatly reduced the need for ECMO in this population. ECMO has become an important adjunct in the management of patients with cardiac failure as a bridge to heart transplantation, during resuscitative efforts, and in perioperative management of patients with complex congenital heart disease. ECMO has also been used to support pediatric patients with severe respiratory failure. Although the criteria for neonatal ECMO are well established, the criteria for pediatric patients are not well defined. Box 48-14 outlines criteria for newborns. ECMO is highly invasive and associated with numerous complications. Bleeding and clot formation are two major concerns. As blood circulates through the ECMO circuit, it comes in contact with a foreign surface. The normal response to this contact is for blood to form clots. To minimize clot formation, the patient receives significant doses of anticoagulant and is at risk for bleeding. Frequent monitoring of the patient’s coagulation status is essential. Mechanical failures of the system are also risks associated with ECMO support. Successful outcome is related to the reversibility of the patient’s underlying condition and minimizing the complications during the ECMO course.
The AARC has published a clinical practice guideline on surfactant replacement therapy. Excerpts from this guideline appear in Clinical Practice Guideline 48-4.
Neonatal and Pediatric Transport
Treatment of a critically ill infant or child is usually provided at a tertiary care facility. Many of these facilities have established transport teams and go to the referring facility, initiate ICU-type support, and transport the patient back to the tertiary care center. The composition of transport teams varies from one institution to another; however, typical team members include some combination of registered nurse, RT, paramedic, nurse practitioner, and physician. Regardless of the composition of the team, there are some characteristics that all transport teams should have in common.71 All members should have exquisite assessment and critical thinking skills. They should be technically adept and have good communication skills. Each team develops minimum criteria that a team member must possess. Many teams cross-train in multiple disciplines to perform certain technical tasks. Establishing proficiency and maintaining proficiency with all skills is a must for team members.
The team essentially functions as an extension of the ICU. To do this, much of the same equipment used in the ICU is taken to the referring hospital. Establishing responsibility for assessing function and maintaining appropriate inventory is essential. Many centers use elaborate checklists to be certain not to be without necessary equipment, disposables, or medications. Teams generally prepare for the worst. Many times when the team arrives at the referring facility, the patient’s condition is not the same as when the initial call for help was made. Being prepared for the worst helps in stabilizing the patient for transport. The American Academy of Pediatrics has guidelines for all ages and common conditions requiring transport to a tertiary facility. Box 48-15 lists the basic equipment and supplies needed to provide respiratory care during neonatal and pediatric transport.