Chapter 5 Muscular Tone and Gait Disturbances
Pathology
The central and peripheral nervous systems modify tone, but intrinsic physical characteristics of the tendons, joints, and muscles and the anatomic interrelationships of these structures also contribute significantly to tone. In childhood central nervous system (CNS) dysfunction, upper motor neuron (unit) disease may cause increased or decreased muscle tone [Teddy et al., 1984]. Disease involving the lower motor neuron (unit) results in hypotonia and weakness.
The final common pathway of upper or lower motor unit modification of tone is through the gamma loop (fusimotor) system [Gordon and Ghez, 1991; Granit, 1975]. Intimately involved with monitoring and effecting tone are the two stretch-sensitive muscle receptors – the muscle spindles and the Golgi tendon organs (Figure 5-1). It also has become evident that nonreflex, mechanical mechanisms are involved in the maintenance of resting muscle tone. Spinal cord reflex responses depend on ongoing activity in interneurons [Davidoff, 1992].
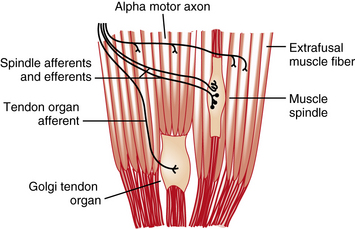
Fig. 5-1 Muscle spindles and Golgi tendon organs are encapsulated structures found in skeletal muscle.
(Adapted from Houck JC, Crago PE, Rymer WZ. Functional properties of the Golgi tendon organs. In: Desmedt JE, ed. Spinal and supraspinal mechanisms of voluntary motor control and locomotion. Progress in Clinical Neurophysiology, vol. 8. Basel: Karger, 1980:33.)
Stationed in all areas of the skeletal muscle is the muscle spindle, a fusiform-shaped receptor structure (Figure 5-2). The spindle is composed of contractile fibers at each end and a capsule covering a central fluid-filled dilatation. Sensory endings wrap around the central sections of the intrafusal fibers and monitor the stretch of these fibers; they communicate through the afferent axons that are described later in this chapter. Through efferent axons, gamma neurons within the anterior horn of the spinal cord innervate the contractile muscle portions on each end of the intrafusal fiber and enhance the sensitivity of the sensory endings to stretch [Gordon and Ghez, 1991]. Gamma motor neurons that innervate muscle spindles comprise the fusimotor system.
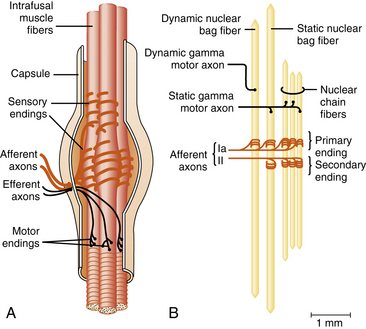
Fig. 5-2 The main components of the muscle spindle are intrafusal fibers, sensory endings, and motor axons.
(A, Adapted from Hullinger M. The mammalian muscle spindle and its central control. Rev Physiol Biochem Pharmacol 1984;101:1; B, Adapted from Boyd IA. The isolated mammalian muscle spindle. Trends Neurosci 1980;3:258. Reproduced with permission from Elsevier.)
The intrafusal muscle fibers are divided into three types: nuclear chain fibers, dynamic nuclear bag fibers, and static nuclear bag fibers. These fibers derive their names from the configuration of their nuclei in the fiber center. Chain fibers have nuclei arranged in a single column, whereas bag fibers have nuclei aligned in rows of two or three. A solitary Ia afferent fiber provides primary sensory innervation for all three types of intrafusal fibers. A group II afferent fiber innervates chain and static bag fibers providing secondary sensory endings. The various sensory endings on the different types of intrafusal fibers have different sensitivities to rate of change of length. Dynamic gamma motor axons innervate the contractile portions of dynamic nuclear bag fibers, and static gamma motor axons innervate the contractile portions of the static bag fibers [Gordon and Ghez, 1991]. This intricate system of muscle spindle innervation allows the muscle stretch receptors to monitor muscle tension, length, and velocity of stretch, and provide input for maintenance of tone [Carew, 1985].
It is through their effect on the gamma motor neuron that portions of the CNS (i.e., motor cortex, thalamus, basal ganglia, vestibular nuclei, reticular formation, and cerebellum) modify tone, with ensuing hypotonia or hypertonia (i.e., spasticity) [Alexander and Delong, 1985; Brooks and Stoney, 1971; Carew, 1985; Ghez, 1985].
The Golgi tendon organs, unlike the muscle spindles, are found in series with the skeletal muscle fibers (Figure 5-3), and are attached at one end to the muscle and at the other to the tendon. A number of individual skeletal muscle fibers enter a Golgi tendon organ through a constricted collar. The muscle fibers are attached to collagen fibers within the Golgi tendon organ. A single Ib axon enters each capsule and forms branches that are interlaced among the collagen fibers. The afferent axon branches are compressed when muscle contraction occurs and impulses are transmitted. Tendon organs are much more sensitive to muscle contraction than muscle spindles. Conversely, tendon organs are much less sensitive to stretch than muscle spindles. Each of these relative sensitivities plays a specific role during the performance of various motor tasks [Gordon and Ghez, 1991].
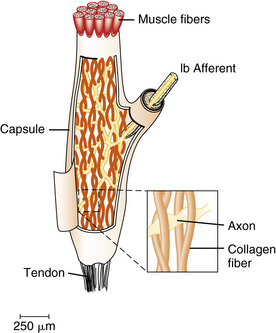
Fig. 5-3 Golgi tendon organs are specialized structures found at the junctions between muscle and tendon.
(Adapted from Schmidt RF. Motor systems. In: Schmidt RF, Thews G, eds. Human physiology [Biederman-Thorson MA, translator]. Berlin: Springer, 1983:81; inset, adapted from Swett JE, Schoultz TW. Mechanical transduction in the Golgi tendon organ: a hypothesis. Arch Ital Biol 1975;113:374.)
Evaluation of the Patient
History
The age at which hypotonia is first evident may be diagnostically crucial. The presence of hypotonia at birth or shortly thereafter can help differentiate among a number of conditions [Brooke et al., 1979; Dubowitz, 1985]. The presence or absence of weak fetal movements or the change from apparently normal fetal movements to those of decreased amplitude and vigor should be determined. Hypotonia in the setting of polyhydramnios signals prenatal interference with swallowing.
A careful genetic history must be sought because several conditions characterized by hypotonia are hereditary. In a retrospective review of hypotonic infants, Birdi et al. reported a family history of neuromuscular or neurologic disorders in almost half [Birdi et al., 2005].
Examination
Tone should be assessed both in the active state and when the neonate is at rest; active tone of the extremities is normally higher than passive tone. Passive pronation, supination, flexion, and extension of the limbs and gently shaking the hands and feet are the best ways to assess tone. The hands move over a large amplitude when the arms are shaken gently at the wrists. Often, in the hypotonic infant, the elbows can be extended beyond their normal range. The scarf sign involves wrapping the infant’s arm across the chest toward the neck on the contralateral side and is positive when the elbow can be readily moved beyond the midline. While abnormal in the term infant, it can normally be seen in preterm infants. The traction maneuver is one of the best means to evaluate tone, since it allows simultaneous evaluation of head control, flexion of elbows during infant participation, and general body and back posture (see Figure 3-7). The hypotonic infant’s foot can be brought to the opposite ear, and extreme passive foot dorsiflexion may be possible when hypotonia is profound.
The pectus excavatum deformity and a bell-shaped chest indicate relative weakness of intercostal muscles compared to better-preserved strength of the diaphragm during respiratory efforts. Skeletal deformities and fixed contractures are often present in congenital myotonic dystrophy and some congenital myopathies. Fixed contractures of the limbs may signal the presence of arthrogryposis multiplex congenita, which may result from dysfunction at a number of lower motor neuron unit sites [Lebenthal et al., 1970; Yuill and Lynch, 1974] (see Chapter 88).
Acute onset of progressive, profound weakness and hypotonia in previously normal infants suggests the possibility of infantile botulism [Infant botulism, 2003; Kao et al., 1976; Pickett et al., 1976; Ravid et al., 2000; Thompson et al., 1980]. There is almost always accompanying constipation, poor feeding, and bulbar involvement.
Weakness is often readily diagnosed in the infant and younger child by observation; in the older child, more formal and discrete muscle testing is possible, as described in Chapter 2. Examination also includes deep tendon reflexes, plantar reflexes, myotonic response to percussion and scrutiny of muscles for evidence of fasciculations.
When the lower motor unit is involved, the deep tendon reflexes range from hypoactive to absent. The reflexes are uniformly absent in infantile spinal muscular atrophy [Bundey and Lovelace, 1975; Smith and Swaiman, 1983]. Reflexes tend to be increased or normal when the upper motor unit is involved, but may be decreased in the case of acute injury or concomitant involvement of the basal ganglia or their output tracts.
Further neurologic examination is necessary and should include the search for fasciculations, ptosis, squint, myotonia, and extensor plantar reflexes. The presence of squint or ptosis suggests the possibility of congenital myopathies [Clancy et al., 1980; McComb et al., 1979; Riggs et al., 2003], myotonic dystrophy, myasthenia gravis [Holmes et al., 1980; Namba et al., 1970], or mitochondrial myopathies (see Chapters 37 and 93). Knowledge about the congenital myopathies has grown considerably during the past decade, and the clinical and genetic complexities have become increasingly evident [Bruno and Minetti, 2004; Kirschner and Bonnemann, 2004].
Diagnosis
It is important to distinguish whether hypotonia is derived from a central or peripheral etiology. While there can be difficulty in distinguishing the localization, one study examined sensitivity and specificity of findings predictive of primary neuromuscular disorders. These included a history of reduced fetal movements with polyhydramnios, significant impairment or absence of antigravity movements, and presence of contractures [Vasta et al., 2005]. Congenital hypotonia may be extremely difficult to categorize; however, certain characteristics may prove helpful in diagnosis (Table 5-1) [Harris, 2008].
Table 5-1 Differentiation of Central versus Peripheral Causes of Congenital Hypotonia
Characteristic | Central | Peripheral |
---|---|---|
Weakness | Mild to moderate | Significant (“paralytic”) |
Deep tendon reflexes | Decreased or increased | Absent |
Placing reaction | Sluggish | Absent |
Motor delays | Yes | Yes |
Antigravity movements in prone and supine | Some (less than normal) | Often absent |
Pull to sit | Head lag (more than normal) | Marked head lag |
Cognition/affect | Delayed | Typical |
Ability to “build up” tone, e.g., tapping under knees with infant in supine to assist him/her in holding hips in adduction | Yes | No |
(From Harris S. Congenital hypotonia. Dev Med Child Neurol 2008; 50:889.)
The presence of hypothyroidism is suggested by decreased length and weight, large tongue, and developmental delay. Some conditions associated with hypotonia are listed in Box 5-1 and are discussed in detail elsewhere in this book.