Microbiological Laboratory Techniques
After reading this chapter, the student will be able to:
• Describe the general concept of aseptic techniques used in laboratory preparation and analysis
• Explain and differentiate between sterilization, disinfection, and sanitization
• Describe the different types of culture media and their possible physical state
• Discuss inoculation, incubation, and isolation
• Describe the various fixation and staining techniques to identify microbes with the light microscope
• Describe the fixation and staining methods used in electron microscopy
• Classify the bacteria according to their shape for morphological identification
• Describe the different culture characteristics of microbes used for the purpose of identification
• Discuss the physiological, biochemical, and genetic characteristics used for microbial identification purposes
Aseptic Technique in Laboratory Preparation and Analysis
Medical and clinical laboratories test biological specimens to determine the health status of a patient and to identify the disease-causing pathogen for the purpose of appropriate treatment. Research laboratories include basic, clinical, and pharmaceutical laboratories, each dealing with a specific aspect of microbiological research.
Microorganisms are everywhere in the environment. In order to selectively identify specific microbes they must be grown in controlled laboratory environments. Beginning with pure sterile cultures, the key is to control the factors to which the cultures are subjected. In other words, when working with microbial cultures, it is necessary to ensure that organisms are selectively introduced into the culture and that other environmental organisms do not contaminate it. Aseptic technique is a procedure that is performed under sterile conditions, a method that prevents the introduction of unwanted organisms or contaminants into an environment. This process is characterized by strict adherence to details. The use of aseptic technique controls, limits, or prevents contamination by fomites. A fomite is any inanimate object or substance capable of transporting pathogens from one medium or individual to another. Aseptic technique is essential in the microbiology laboratory to prevent any contamination of laboratory personnel (see Chapter 5, Safety Issues), cultures, supplies, and equipment.
Air currents must be controlled by closing laboratory doors and windows to prevent microbes on surfaces from becoming airborne and entering the cultures. When handling cultures to prepare slides or to transfer organisms to another medium, the transfer loops and needles need to be sterilized by flame or incinerator before and after use (Figure 4.1). Furthermore, culture plates are held in a position that minimizes exposure of the medium to the environment. When removing lids/stoppers from test tubes, the lids should remain held in the hand and not placed on other surfaces such as countertops during the transfer of materials from one tube to another. Flaming is one of the physical methods of sterilization and must always be applied to the lips of test tubes and also of flasks whenever culture liquid is poured from one container to another, as in the case of pouring culture plates (Figure 4.2).
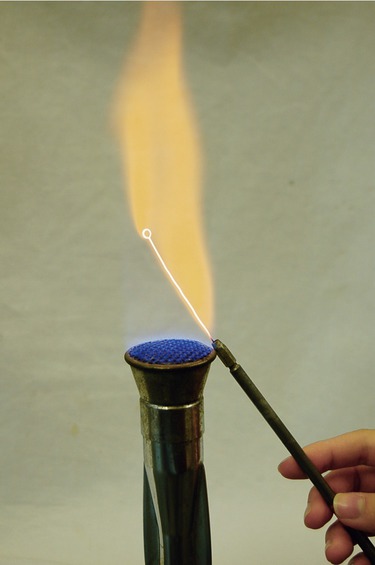
One of the primary inoculation tools in the microbiology laboratory is the loop. Metal loops must be sterilized in the flame of a Bunsen burner by heating the wire until it glows. Presterilized, disposable plastic loops are also available for inoculation.
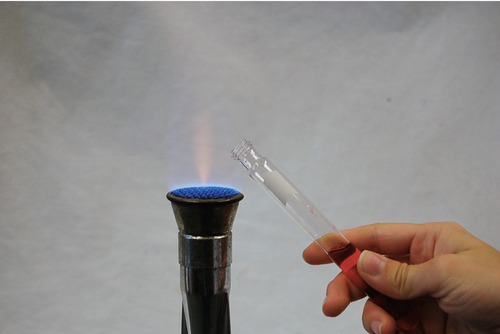
Bacteria and fungi are everywhere in the environment, including the air. The mouth of a tube or bottle opening is a potential point of contamination and flaming of the opening in direct flame immediately after opening and before closing is an effective technique to prevent potential contamination on this exposed surface.
Sterilization and disinfection procedures are daily routines in microbiological laboratories. They are essential to ensure that cultures, containers, media, and equipment are handled in such a way that only the desired organism will grow and others will be eliminated or excluded.
Sterilization
Sterilization is the destruction or removal of all microorganisms, including bacteria and their endospores, viruses, fungi, and prions. This can be accomplished by physical methods such as heat, radiation, and filtration, or by chemical methods. The wide application of sterilization processes makes it necessary to impose strict control measures to validate the results. When using dry heat or moist heat sterilization, physical, chemical, or biological indicators can be used to validate the desired results (Table 4.1). The general resistance of microbes to methods of sterilization ranges from bacterial endospores, with the highest resistance to sterilization, to vegetative cells, with moderate to least resistance.
TABLE 4.1
Methods for Validating Dry or Moist Heat Sterilization
Physical Methods | Chemical Methods | Biological Test Organism | |
Dry heat | Temperature-recording charts | Color change indicator | Bacillus subtilis var. niger |
Moist heat | Temperature-recording charts | Color change indicator | Bacillus stearothermophilus |
Physical and chemical methods of sterilization are applied in the microbiological laboratory to ensure that equipment and materials are free of microorganisms. Aseptic technique is the first and most important step in ensuring that manipulation of specimens during investigative procedures does not infect laboratory personnel or contaminate cultures or the laboratory environment (see Chapter 5, Safety Issues). Bacteria are found practically everywhere including fingertips and bench tops and therefore it is essential to minimize contact with these surfaces. Only sterile items are free of potentially contaminating microorganisms and once a sterile object comes in contact with a nonsterile surface, the object can no longer be considered sterile. The most commonly used instrument in the microbiology laboratory for the sterilization of media and glassware is the autoclave (Figure 4.3). A detailed discussion of the different types of physical and chemical methods of sterilization is provided in Chapter 19 (Physical and Chemical Methods of Control; for overviews see Tables 19.3 and 19.5).
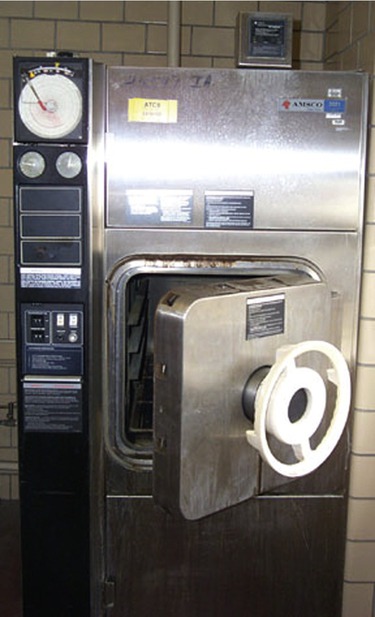
The autoclave is one of the primary tools for sterilization of equipment, containers, media, and biohazardous wastes. It is essentially a large pressure cooker that raises the temperature of steam to about 121° C under 15–20 psi pressure. The size and configuration of autoclaves vary but the basic operation is same.
Disinfection
Disinfectants are applied to inanimate surfaces, medical equipment, and other man-made objects whereas antiseptics are used to disinfect skin. The term disinfection refers to the use of a physical process or the use of a chemical agent to destroy vegetative microbes and viruses. This does not include bacterial endospores. The ideal disinfectant would result in complete sterilization without harming other forms of life. Unfortunately, ideal disinfectants as such do not exist and most of them only partially sterilize. In addition to the most resistant pathogens, endospores, other bacteria, and viruses are also highly resistant to many disinfectants.
Substances that kill bacteria are bactericidal and those that interfere with cell growth and reproduction are bacteriostatic. Disinfectants and antiseptics are bactericidal and bacteriostatic depending on the concentration applied. All disinfectants are by their nature potentially harmful, even toxic, to humans and animals. They should be handled with appropriate care to avoid harm to the handler or recipient. The type of disinfectant to be used depends on the surface or material to be disinfected. Specifics on the different types of disinfectants and their particular effectiveness are described in Chapter 19, with an overview in Table 19.5 (Chemicals Used in the Control of Microbes).
Sanitization
Several applications in everyday life and medicine do not require sterilization, disinfection, or antisepsis but need to reduce microorganisms in order to control possible infections or spoilage of substances. Sanitization achieves this by using any cleansing technique that mechanically removes microorganisms and other debris to reduce contamination to safe levels. Often the sanitizer used is a compound such as soap or detergent. Restaurants, dairies, breweries, and other food industries handle soiled utensils on a daily basis and must take appropriate measures to sanitize them for prevention of infection, spoilage, and contamination. This includes controlling microbes to a minimal level during preparation and processing.
Degermation is the process by which the numbers of microbes on the human skin are reduced by scrubbing, immersion in chemicals, or both. Some examples of degermation include the process of presurgical scrubbing of the hands with sterile brushes and germicidal soap before putting on sterile surgical gloves, the application of alcohol wipes to the skin, and the cleansing of a wound with germicidal soap and water.
Culture Techniques
Microbiologists use five basic procedures to examine and characterize microbes: Inoculation, Incubation, Isolation, Inspection (observation), and Identification—the five “I’s.” To culture a microorganism a small sample, the inoculum, is introduced into a culture medium usually with a platinum wire probe streaked across its surface. This process is called inoculation and the growth that appears on or in the medium is the culture. A culture can be pure—containing one type of organism, or mixed—containing two or more species.
Types of Culture Media
Nutritional requirements of particular microorganisms range from a few simple inorganic compounds to a complex list of specific inorganic and organic chemicals (Table 4.2). Access to carbon, the essential component required for molecular life, is obtained in different ways by microorganisms. Autotrophs acquire carbon from carbon dioxide in the atmosphere and heterotrophs obtain their carbon from organic compounds. This diversity is seen in the different types of media needed to ensure the growth of the organism for investigation. Media vary in nutrient content and consistency and can be classified according to their physical state, chemical composition, and functional type.
TABLE 4.2
Nutritional Requirements for Microorganisms*
Macronutrients | Growth Factors (Vitamins) | Micronutrients (Trace Elements) |
Carbon | p-Aminobenzoic acid | Boron |
Hydrogen | Folic acid | Chromium |
Oxygen | Biotin | Cobalt |
Nitrogen | Cobalamin (B12) | Copper |
Phosphorus | Lipoic acid | Iron |
Sulfur | Nicotinic acid (niacin) | Manganese |
Potassium | Pantothenic acid | Molybdenum |
Magnesium | Riboflavin | Nickel |
Sodium | Thiamine | Selenium |
Calcium | Vitamin B6 | Tungsten |
Iron | Vitamin K group | Vanadium |
Hydroxamates | Zinc |
*Not all microbes need all of the nutrients listed; therefore, for optimal growth environments media with specific nutrients are necessary for specific microorganisms.
Physical State of Media
Liquid media are water-based solutions that do not solidify at temperatures above freezing and flow freely in the containers when tilted. Most commonly, liquid media are supplied in tubes or bottles and are called broths, milks, or infusions. A common laboratory medium is nutrient broth, which contains beef extract and peptone (partially digested protein) dissolved in water. Methylene blue milk and litmus milk are opaque liquids prepared from skim milk powder and dyes. After inoculation, growth occurs throughout the container. Enriched broths are used to grow bacteria that are present in few numbers such as in small specimen samples obtained from patients.
Semisolid media contain a limited amount of a solidifying agent such as agar or gelatin, giving the medium a clotlike consistency. Semisolid media are often used to determine motility and growth patterns of bacteria.
Solid media are dispensed in Petri plates or slanted in tubes or bottles to provide firm and maximal surfaces for growing bacteria or fungi. By far the most widely used and effective of these media is agar, composed of a complex polysaccharide from the red alga Gelidium. Agar is solid at room temperature and liquefies at the boiling temperature of water. Once in liquid form it does not solidify until it cools to 42° C. It then can be inoculated and poured in liquid form at temperatures that will not harm the microbes or the handlers. Agar added to media simply gels them into a solid form. Any medium containing 1% to 5% agar usually has the agar in the name of the specific medium as, for example, nutrient agar, phenylethyl alcohol agar, blood agar, and others.
Chemical Classification of Media
Depending on their chemical content media can be classified as complex or nonsynthetic media and as chemically defined or synthetic media.
• Chemically defined media or synthetic media are media with a defined, exact chemical composition. They are prepared by means of an exact formula, adding precise amounts of inorganic and/or organic chemicals to distilled water. Some of these media contain minimal amounts of chemicals such as some salts and a source of carbon; others are special media containing a variety of precisely measured substances.
• Complex media or nonsynthetic media contain at least one component that cannot be chemically defined and thus the medium cannot be represented by an exact chemical formula. Complex media contain extracts from animals, plants, or yeast. They may include blood, serum, meat extracts, milk, yeast extracts, soybean digests, and peptone.
Functional Types of Media
General-purpose media are designed to grow a broad spectrum of microbes that do not have any special growth requirements. Other media are available for special growth conditions of selected organisms. These include enriched, selective, and differential media.
• Enriched media contain complex organic substances such as blood, serum, hemoglobin, or growth factors for the growth needs of specific species. An example is blood agar, made by adding sterile sheep, horse, or rabbit blood to a sterile agar base. It is widely used to grow certain streptococci and other pathogens. Another enriched medium is chocolate agar. Chocolate agar is enriched with heat-treated blood, which turns brown and gives the medium the color and thus its name.
• Selective media inhibit the growth of selected organisms while allowing the growth of others. These media are useful in isolating bacteria or fungi from specimens that contain several different organisms. For example, mannitol salt agar contains 7.5% NaCl, inhibitory to most human pathogens with the exception of the genus Staphylococcus, which thrives in mannitol salt agar and consequently its growth can be amplified in mixed samples.
• Differential media can grow several different organisms that show visible differences. These differences can be variations in colony size or color, a change in medium color, or the formation of gas bubbles and precipitates. Dyes can be used as differential agents because many of them are pH indicators that change color in response to acid or base production by a specific microbe. For example, MacConkey agar contains neutral red, which is a dye that is yellow when neutral and pink or red when acidic. Escherichia coli, a bacterium common to the intestinal tract, produces acid when it metabolizes the lactose in the medium and develops red or pink colonies. In contrast, Salmonella does not give off acid and therefore remains in a natural off-white color. A comparison of general, selective, and differential media is shown in Figure 4.4.
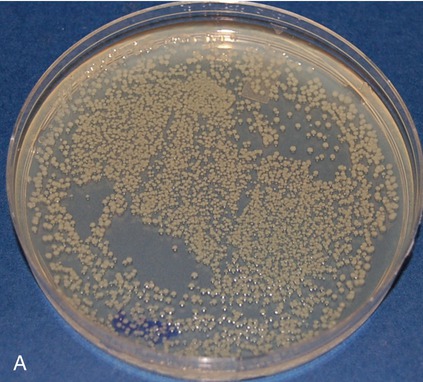
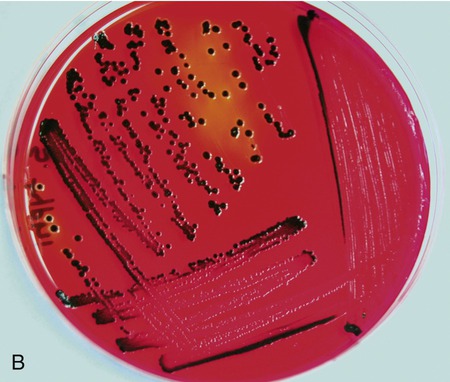
Pictured are (A) tryptic soy agar—a complex medium used as an all-purpose growth medium, and (B) xylose lysine deoxycholate agar—a chemically defined agar that is both selective and differential and used primarily in selecting for and differentiating gram-negative enteric bacilli, especially Shigella, Salmonella, and Providencia. Yellow indicates the organism is utilizing the carbohydrate xylose and the black colonies indicate the production of hydrogen sulfide.
HEALTHCARE APPLICATION
Growth Requirements of Selected Bacteria
Bacterium | Medium | Atmospheric Conditions |
Pseudomonas aeruginosa
Buy Membership for Basic Science Category to continue reading. Learn more here
|