Chapter 19 Injury to the Developing Preterm Brain
Intraventricular Hemorrhage and White Matter Injury
Introduction
As newborn intensive care approaches its sixth decade, preterm birth has emerged as a major pediatric public health problem [Behrman and Stith Butler, 2007]. There were almost 129 million births worldwide in 2005, and 9.6 percent of them were premature [Beck et al., 2010]. Survival is increasing for neonates of all gestational ages, and the prevalence of children with cognitive impairments at school age continues to rise [Allen, 2008; Behrman and Stith Butler, 2007; Hack et al., 2009; Robertson et al., 2009]. At age 8 years, more than 50 percent of infants with birth weights < 1000 g require special classroom assistance in the classroom, 20 percent are in special education, and 15 percent have repeated at least one school grade [Allen, 2008; Aylward, 2005; Bhutta et al., 2002; Larroque et al., 2008; Neubauer et al., 2008; Saigal and Doyle, 2008; Voss et al., 2007]. In addition, 10–20 percent have cerebral palsy [Larroque et al., 2008].
Brain injury is common in preterm neonates and may perturb the genetically prescribed program of cerebral development in the prematurely born [Volpe, 2009a]. Magnetic resonance imaging (MRI) has permitted noninvasive high-resolution evaluation of the developing brain, and numerous investigators have reported that very low birth weight (VLBW) preterm infants experience both macrostructural alterations in postnatal brain development, as well as in microstructural connectivity when compared to term neonates [De Vries et al., 1999; Hagmann et al., 2009; Huppi et al., 1996; Maalouf et al., 1999; Mercuri et al., 1996; Woodward et al., 2006]. To date, however, both the pathophysiology of these changes and the relationship of MRI studies to neurodevelopmental outcome data remain poorly understood [Allin et al., 2004; Boardman et al., 2007; Huppi et al., 1998; Inder et al., 1999, 2005; Mewes et al., 2006; Peterson et al., 2000]. These data suggest that strategies for identifying and preventing causes of disability in this patient population are critically important to both physicians and parents.
The neuropathologic processes that most commonly affect the prematurely born neonate include intraventricular hemorrhage (IVH) and white matter injury, or the periventricular leukomalacia complex [Volpe, 2009a]. IVH, or hemorrhage into the germinal matrix tissues of the developing brain, is detected in approximately 10–25 percent of VLBW neonates [Fanaroff et al., 2007; O’Shea et al., 2008], while white matter injury (WMI) and the global neuronal and axonal deficits that may accompany it are found in 50 percent or more of this vulnerable population [Dyet et al., 2006; Maalouf et al., 1998; Woodward et al., 2006]. Because both IVH and WMI are unique to the preterm population and represent a significant burden of disease, the pathophysiology, outcome, and prevention of these two injuries will be reviewed separately.
Intraventricular Hemorrhage
Although IVH occurs in 10–25 percent of all VLBW neonates, it is most significantly a problem for those infants at the lowest limits of viability [Fanaroff et al., 2007]. Both the incidence and the severity of IVH are inversely related to birth weight and gestational age, and almost 25 percent of neonates of 501–750 g birth weight experience the highest grades of hemorrhage [Fanaroff et al., 2003, 2007]. High-grade IVH is more common in male infants than females [Hintz et al., 2006; Synnes et al., 2001; Tioseco et al., 2006], and although the risk period for IVH is in the first 4–5 postnatal days, data from several centers indicate that approximately half of all hemorrhages occur within the first 6–8 postnatal hours [Dolfin et al., 1983; Ment et al., 1993; Perlman and Volpe, 1986; Perlman and Rollins, 2000; Shaver et al., 1992] – a time of intense medical instability for those neonates with the lowest gestational ages.
Many neonatal intensive care units perform routine head ultrasound screening of preterm neonates using the system developed by Papile for grading IVH, as shown in Table 19-1 [O’Shea et al., 2008; Papile et al., 1978]. Employing this nomenclature, grade 1, or germinal matrix hemorrhage (GMH), describes blood in the germinal matrix only. Grade 2 is blood filling the lateral ventricles without distension, grade 3 is blood filling and acutely distending the ventricular system, and grade 4 describes hemorrhages with parenchymal involvement of hemorrhage. When associated with grades 1–3 IVH, unilateral grade 4 hemorrhages are considered in most cases to represent venous infarction of the periventricular white matter [de Vries et al., 2001], and the nomenclature of Volpe [Volpe, 2001], also shown in Table 19-1, provides a more pathophysiologic approach to parenchymal events.
In the newborn period, 5–10 percent of preterm infants with IVH suffer seizures and as many as 50 percent of infants with grade 4 IVH experience posthemorrhagic hydrocephalus [Volpe, 2009a]; mortality is also higher in those infants with IVH. Finally, although the long-term neurodevelopmental outcome for infants with lower grades of hemorrhage remains uncertain at this time, most observers agree that infants with parenchymal hemorrhage are at high risk for neurodevelopmental handicap (Figure 19-1) [Luu et al., 2009a; Saigal et al., 2003; Sherlock et al., 2005, 2008].
Pathophysiology
Intraventricular Hemorrhage is a Complex Disorder
IVH has been attributed to alterations in cerebral blood flow to the immature germinal matrix microvascular network [Shalak and Perlman, 2002; Whitelaw, 2001], as shown in Figure 19-2, and studies addressing the etiology of IVH have identified numerous environmental and medical risk factors (Box 19-1). These include low gestational age, absence of antenatal steroid exposure, maternal antenatal hemorrhage, chorioamnionitis/infection/inflammation and/or fertility treatment, neonatal transport to a tertiary care center following birth, maternal sepsis, seizures, hypotension requiring medical therapy, hypoxemia, hypercapnia, and acidosis [du Plessis, 2008; Fabres et al., 2007; Limperopoulos et al., 2008; McCrea and Ment, 2008; O’Leary et al., 2009]. As this lesion is unique to preterm infants, examination of those developmental, environmental, and genetic events that result in IVH is critical. These include anatomic, physiologic, and genetic factors.
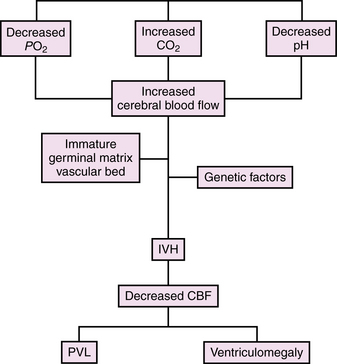
Fig. 19-2 Model for the development of intraventricular hemorrhage in the preterm infant.
CBF, cerebral blood flow; IVH, intraventricular hemorrhage; PVL, periventricular leukomalacia.
Anatomic Factors are Permissive for Hemorrhage
During the late second and third trimesters, the developing brain almost triples in volume, and there are multiple developmental events that occur during this time interval. The germinal matrix and the adjacent subventricular zone (SVZ) are the sites of proliferation of both glial and neuronal precursors. The SVZ generates predominately GABAergic cortical interneurons, although recent data suggest that the germinal matrix also produces GABAergic neurons that migrate to the dorsal thalamus during the risk period for IVH. (For review, please see Volpe [2009a].)
By 24 weeks of gestation, almost but not all of the cortical neurons have migrated from the germinal zones and axonal ingrowth begins [Kostovic, 1990; Kostovic and Rakic, 1990; Kostovic et al., 2002, 1989]. The elaboration of dendritic arbors is at an active stage and synaptic contacts are beginning to form [Huttenlocher and de Courten, 1987; Huttenlocher and Dabholkar, 1997; Huttenlocher et al., 1982]. The germinal matrix remains relatively robust through 32–34 weeks of gestation but almost completely involutes by term [Donat et al., 1978; Rorke, 1982; Whitelaw, 2001].
During this period of rapid cell genesis, the metabolically active germinal matrix requires a rich blood supply, as shown in Figure 19-3, yet its vessels are neuropathologically immature and present numerous risk factors for hemorrhage (Table 19-2). The developing blood–brain barrier is characterized by basement membrane proteins, endothelial tight junctions, capillary pericytes, and astrocytic endfeet [Ballabh et al., 2004]. Immunohistologic and electron microscopic examinations comparing germinal matrix microvessels with those in the periventricular white matter and cortical mantle demonstrate a paucity of fibronectin, fewer pericytes, and decreased perivascular coverage by glial fibrillary acidic protein (GFAP)-positive astrocytic endfeet [Ballabh et al., 2005; Braun et al., 2007; El-Khoury et al., 2006; Wei et al., 2000; Xu et al., 2008]. Since GFAP provides both shape and mechanical strength to the endfeet of astrocytes, its decrease, in combination with alterations in basement membrane proteins and pericyte investiture, suggests a structural weakening of the germinal matrix blood–brain barrier. In contrast, studies of fetuses and preterm neonates during the second and third trimesters of gestation have demonstrated no difference in the primary endothelial tight junction molecules, claudin-5, occludin, and JAM-1, in the germinal matrix compared to cortical gray and white matter regions [Ballabh et al., 2005; Xu et al., 2008].
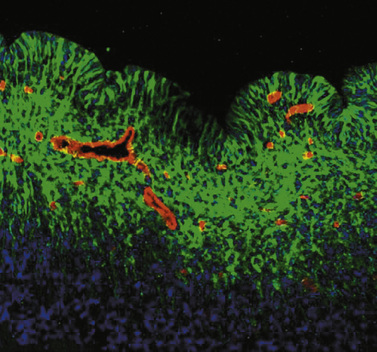
Fig. 19-3 Morphology of the germinal matrix.
(Courtesy of Praveen Ballabh, M.D., Department of Pediatrics, Anatomy, and Cell Biology, New York Medical College-Westchester Medical Center, Valhalla, NY.)
Table 19-2 Germinal Matrix Risk Factors for Intraventricular Hemorrhage
Factor | Developmental Regulation in GM | Changes in GM |
---|---|---|
Vascular density and area | Increase with GA | GM > cortex, WM |
Blood–brain barrier | ||
Tight junction proteins Claudin-5 Occludin Junction adhesion molecule 1 |
No change No change No change |
No differential expression No differential expression No differential expression |
Basement membrane proteins Laminin Collagen IV Fibronectin |
No change Increase with GA No change |
GM > cortex, WM No change GM < cortex, WM |
Glial endfeet Glial fibrillary acidic protein |
Increase with GA | GM < cortex, WM |
Pericytes Vascular coverage |
Increase with GA | GM < cortex, WM |
Angiogenic factors VEGF Angiopoietin-2 |
N/a N/a |
GM > cortex, WM GM > cortex, WM |
GA, gestational age; GM, germinal matrix; N/a, not available; VEGF, vascular endothelial growth factor; WM, white matter.
The germinal matrix has a greater vascular density than other developing brain regions, and recent reports suggest rapid angiogenesis in the germinal matrix, perhaps to support its greater metabolic rate and oxygen requirement [Ballabh et al., 2004]. This angiogenesis is induced by the high levels of vascular endothelial growth factor (VEGF) and angiopoietin (ANGPT)-2 found selectively in the germinal matrix compared to both the cortex and periventricular white matter [Carmeliet, 2003; Ferrara et al., 2003]. Since preclinical studies demonstrate that an increase in the expression of ANGPT-2 in the presence of VEGF promotes the sprouting of immature vessels lacking both basement membrane proteins and pericyte investiture [Carmeliet, 2003; Yancopoulos et al., 2000], the increased presence of these factors in the highly vascular germinal matrix provides further evidence for microvessels lacking a competent blood–brain barrier [Ballabh et al., 2007].
Finally, the venous circulation of the preterm brain may also contribute to the susceptibility to intraparenchymal hemorrhage. Venous blood from the periventricular white matter flows through a fan-shaped array of both short and long medullary veins into the veins of the germinal matrix, and subsequently into the terminal veins found inferior to this highly proliferative zone. The somewhat tortuous anatomy of these veins permits venous stasis, with subsequent hemorrhagic venous infarction of the periventricular white matter [Gould et al., 1987; Takashima et al., 1986; Taylor, 1995].
Alterations in Cerebral Blood Flow Contribute to IVH
Both experimental animal models (i.e., “preclinical data”) and clinical data suggest that alterations in cerebral blood flow may play an important role in the pathophysiology of IVH. (For review see du Plessis [2008].) Cerebral blood flow was reported to be pressure-passive in the asphyxiated preterm infant over four decades ago [Lou et al., 1979], yet the factors that contribute to cerebral autoregulatory systems in the prematurely born are just beginning to be explained. Further, the definition of the cerebral pressure-flow autoregulatory plateau, or that range of cerebral perfusion pressures (mean arterial pressure − cerebral venous pressure) over which cerebral blood flow is normally maintained, is poorly defined for the critically ill, extremely low birth weight preterm neonate. None the less, experimental animal data suggest that at perfusion pressures above this reportedly relatively narrow plateau, cerebral blood flow becomes pressure-passive.
Changes in perfusion pressure, alterations in circulating oxygen and carbon dioxide levels, and the magnitude of these respiratory fluctuations are perhaps the most important regulators of cerebral blood flow in the preterm neonate. In response to these physiologic alterations, cerebral blood flow rises, hemorrhage begins within the germinal matrix, and blood may rupture into the ventricular system. After ventricular distension by an acute hemorrhage event, cerebral blood flow falls. Venous stasis within the periventricular white matter ensues, and parenchymal venous infarction may soon develop [McCrea and Ment, 2008; Volpe, 2001].
Prostaglandins, and particularly the cyclo-oxygenase 2 (COX-2) system, are important modulators of cerebral blood flow in the developing brain [Leffler et al., 1985, 1986]. Hypoxia, hypotension, growth factors, including transforming growth factor-β and epidermal growth factor, and inflammatory modulators, such as interleukin (IL)-6, IL-1β, tumor necrosis factor α (TNFα), and nuclear factor κβ, may all induce COX-2 [Baier, 2006; Dammann and Leviton, 2004; Heep et al., 2003, 2005; Ribeiro et al., 2004]. The resultant increase in prostanoids results in the release of VEGF, the potent angiogenic factor associated in preclinical and clinical studies with IVH.
Following hemorrhage, these same triggers set into motion a cascade of events leading to disruption of tight junction proteins, increased blood–brain barrier permeability, and microglial activation within the developing white matter. The damaged endothelium releases IL-1β and TNFα, and these cytokines promote transmigration of leukocytes across the emerging blood–brain barrier. The activated microglia release reactive oxygen species (ROS), which in turn promote endothelial damage, alter hemostasis, and increase metabolism. (For review, please see du Plessis [2008] and Chua et al. [2009].) The preterm brain is very sensitive to ROS because of the immaturity of those enzyme systems designed to detoxify them, and experimental animal studies suggest that ROS play a significant role in the generation of periventricular parenchymal infarction [Zia et al., 2009].
Clinical events believed to be associated with increases in cerebral blood flow in the preterm infant and the presumed postnatal onset of IVH include endogenous and exogenous events. Vigorous resuscitation, rapid volume re-expansion, endotracheal tube repositioning, recurrent suctioning, complex nursing care procedures, and the administration of sodium bicarbonate, as well as respiratory distress syndrome, hypoxemia, extremes of PCO2 levels (i.e., both hypo- and hypercapnia), and acidosis have all been associated with IVH [Ancel et al., 2005; Hall et al., 2005; Kaiser et al., 2006; Kluckow, 2005; Kuint et al., 2009; Linder et al., 2003; O’Leary et al., 2009; Osborn and Evans, 2004; Synnes et al., 2001; Whitelaw, 2001] (Box 19-2). Routine screening by bedside cranial ultrasonography has demonstrated IVH after seizures and pneumothoraces in infants who were previously known to have no evidence of hemorrhage [Cooke, 1981; Hill and Volpe, 1981; Hill et al., 1982]. Finally, cerebrovasoactive cytokines are released in association with sepsis and may contribute to IVH.
Cerebral blood flow is markedly diminished after hemorrhage [Del Toro et al., 1991; Volpe, 1997]. Both xenon-133 inhalation and positron emission tomography (PET) have demonstrated significant hypoperfusion for longer than the first postnatal week in preterm infants with IVH [Ment et al., 1984; Volpe et al., 1983]. The cerebral hypoxemia and ischemia that accompany this depression in flow may result in damage not only to the periventricular white matter but also to those neural and glial precursors still residing in the germinal matrix and SVZ. Oligodendroglia and their precursors are among the cells in the developing cortex most sensitive to hypoxia, and their loss is postulated to result in secondary, gestational age-dependent alterations in connectivity in the developing brain [Gimenez et al., 2006; Miller et al., 2002; Nosarti et al., 2009]. Consistent with this hypothesis are reports of significantly decreased cortical gray matter volumes at term equivalent in VLBW preterm infants with grades 1–3 IVH compared to those with no IVH [Vasileiadis et al., 2004], and of decreases in cortical gray and white matter volumes, as well as callosal areas, in preterm subjects at 8–15 years of age [Kesler et al., 2004; Nosarti et al., 2008].
Candidate Genes for IVH
Although the environmental risk factors for IVH have been well studied, not all infants of a given birth weight range or those who suffer a known hypoxemic event experience IVH. Further, the incidence of high-grade IVH has not changed during the past 10 years despite improvements in care (Table 19-3) [Fanaroff et al., 2003, 2007]. Finally, twin analyses show that intraventricular hemorrhage is familial in origin, and over 40 percent of the variance in liability for IVH can be accounted for by shared genetic and environmental factors [Bhandari et al., 2006]. For these reasons, several investigators have tested the hypothesis that the etiology of IVH is multifactorial, involving both environmental and genetic events.
Table 19-3 Selected Perinatal Information for Infants Born with Birth Weights 500–1000 g in the National Institute of Child Health and Human Development (NICHD) Neonatal Research Network in Three Epochs
The description of the thrombophilias associated with the factor V Leiden and prothrombin G2021A mutations, and the implication of both abnormalities in fetal and neonatal stroke, suggested to several investigators that these might also be candidate genes for IVH [Debus et al., 1998; Gopel et al., 2001, 2002; Petaja et al., 2001]. Examination of other target genes in the presumptive pathway to IVH shortly followed. As shown in Table 19-4, these include genes contributing to hemostasis, vascular stability, and inflammation [Baier, 2006].
Gene | Allele | Effect |
---|---|---|
Thrombophilia and Coagulation Genes | ||
Factor V Leiden | Gln506-FV | Decreased inactivation of factor V Increased thrombin generation Candidate gene for perinatal stroke and IVH |
Prothrombin | G20210A | Increased thrombin Candidate gene for perinatal stroke and IVH |
Factor XIII | 34 Leu allele | High fibrinolytic activity in germinal matrix Associated with hemorrhagic events in adults Candidate gene for IVH |
MTHFRC mutations | Result in increased plasma homocysteine Increased thrombosis Candidate gene for IVH |
|
Vascular Stability Genes | ||
COL4A1 | Decreased vascular stability Candidate gene for fetal stroke and IVH |
|
Proinflammatory Cytokines | ||
TNF-α | Biallelic G to A at -308 | A allele confers high gene transcription A allele is candidate gene for IVH |
IL-1β | -511 CT | Increased production T allele is a candidate gene for IVH |
IL-6 | 174 G or C | CC genotype increases production CC genotype is candidate gene for IVH |
Thrombophilia and coagulation genes
Numerous investigators have examined the role of factor V Leiden (FVL) mutation, polymorphisms in those genes responsible for prothrombin, factor XIII, MTHFRC, and total homocysteine in the genesis of IVH [Debus et al., 1998; Gopel et al., 2001, 2002; Komlosi et al., 2005; Petaja et al., 2001].
A point mutation in the factor V gene resulting in the arginine 506 to glutamine substitution in factor V (Gln506-FV) is known as the FVL mutation. FVL is common in the Caucasian population and causes decreased inactivation of factor V, leading to markedly increased thrombin generation [Bertina et al., 1994]. Importantly, FVL has been associated with thrombotic conditions in both adult and pediatric populations.
The G20210A polymorphism in the prothrombin gene results in a G to A substitution at nucleotide 20210; this mutation increases thrombin and thus also increases the risk for thrombosis. FVL and prothrombin G20210A have been reported in children and infants with ischemic stroke, porencephaly, and other thromboembolic events. Gopel hypothesized that relative hypercoagulability would protect preterm neonates from the extension of grade 1 IVH to higher grades of hemorrhage [Gopel et al., 2001]. In this study of 305 infants with birth weights <1500 g, the FVL mutation was protective for grades 2–4 IVH. In contrast, Petaja hypothesized that the hypercoagulable state associated with FVL would promote periventricular venous infarction and render the infant susceptible to parenchymal involvement of IVH; this study of 51 infants of <32 weeks gestational age suggested that FVL was associated with grade 4 IVH (odds ratio 5.9) [Petaja et al., 2001]. Aronis evaluated 55 preterm infants of <32 weeks gestational age for FVL and prothrombin mutations, and noted a “trend” for thrombophilias among infants with the latter mutation [Aronis et al., 2002].
Factor XIII levels are low in the preterm neonate, and this finding may result in high fibrinolytic activity in the germinal matrix. The factor XIII 34 Leu allele has been reported to cause hemorrhage events in adults and increased IVH in preterm neonates [Gopel et al., 2002].
Finally, mutations in the 5,10 methylenetetrahydrofolate reductase gene (MTHFRC) lead to increased plasma homocysteine levels, a well-recognized risk factor for increased thrombosis. None the less, several authors have reported no association with either these mutations or total homocysteine levels at birth in preterm neonates with IVH [Aronis et al., 2002; Gopel et al., 2001].
Mutations in vascular stability genes
Numerous vascular, astrocytic, and pericytic proteins contribute to the maturation of the developing blood–brain barrier, and allelic alterations in these genes and their impact on IVH are just beginning to be described. Recent data suggest that mutations in collagen IVA1 result in IVH in neonatal mice, and fetal stroke and porencephalies in human infants [de Vries et al., 2009; Gould et al., 2005, 2006]. More recently, a mutation in this important vascular structural protein has been reported in preterm twins with grade 4 IVH [Bilguvar et al., 2009].
Mutations in proinflammatory cytokines
Proinflammatory cytokines are believed to represent important mediators of perinatal brain injury, and TNFα is known to be a central mediator in the inflammatory cascade. The degree of TNFα production may in part be genetically driven. A biallelic G to A polymorphism at position −308 in the TNFα promoter region confers either high (A) or low (G) gene transcription [Kroeger et al., 1997]. Hypoxia and ischemia, both of which are known to contribute to the pathogenesis of IVH, are associated with the upregulation of TNFα. Baier examined the role of this mutation in ventilated VLBW preterm neonates and found that the TNFα-308A allele was associated with an increased risk of IVH (40 percent for infants with an A allele compared to 24 percent with the GG genotype) [Adcock et al., 2003].
Polymorphisms in interleukins may also be possible genetic modifiers for IVH, although the results require further validation. These include mutations in IL-1β, IL-6, and IL-4. The IL-1β-511 CT mutation results in increased production of this proinflammatory cytokine and has been reported to increase the risk of IVH in ventilated preterm neonates with the T allele (33 percent vs. 14 percent for neonates with the C allele) [Baier, 2006]. Similarly, IL-6 mutations are believed to be strong candidate genes for IVH. Position 174 can be either a G or a C, and IL-6 production is greater in neonates with the CC genotype, but studies investigating IVH rates and/or and neurodevelopmental outcome in this subject population are contradictory [Gopel et al., 2006; Harding et al., 2004]. Similarly, a polymorphism at position 472 of the IL-6 gene has been shown in a small study to be associated with alterations in neurodevelopmental outcome in preterm infants at 2 years of age, although the study infants did not appear to have an increased incidence of IVH [Harding et al., 2005]. Finally, in a single study, mutations in IL-4 have been associated with a decreased incidence of IVH in African-American neonates [Baier, 2006].
Neuropathology
Over 90 percent of all IVH in preterm infants originates in the germinal matrix tissues located between the caudate nucleus and the thalamus at the level of, or slightly posterior to, the foramen of Monro in the developing brain [Donat et al., 1978; Volpe, 1997]. A small percentage of hemorrhages in preterm neonates may also originate from the choroid plexus, which is the most common site of IVH in term infants [Rorke, 1982].
Although it is often difficult to differentiate the neuropathologic findings that are directly attributable to IVH from those that frequently accompany it, most authors would agree that germinal matrix destruction, periventricular hemorrhagic infarction, porencephaly, and posthemorrhagic hydrocephalus (PHH) are the direct neuropathologic consequences of IVH [Shalak and Perlman, 2002; Whitelaw, 2001]. In contrast, periventricular leukomalacia and ventriculomegaly, to be described later in this chapter, are frequently detected in infants with IVH but are multifactorial in etiology.
Germinal matrix hemorrhage (GMH), solely confined to the germinal layer, occurs equally in both hemispheres and is found to be bilateral in approximately half of cases [Leech and Kohnen, 1974]. Not infrequently, there are multiple hemorrhages over one caudate nucleus, and injection techniques have demonstrated that all of the major vessels supplying the germinal matrix, including the artery of Heubner, the anterior choroidal artery, the lateral striate arteries, and the terminal vein, remain intact after an initial GMH [Pape and Wigglesworth, 1979]. Such a hemorrhage is associated with destruction of the capillary bed, and secondary germinal matrix cyst formation is common [Hambleton and Wigglesworth, 1976].
After rupture of a GMH, the spread of blood throughout the ventricular system is variable. Sonograms may demonstrate clots attached not only to the site of rupture but also to the choroid plexus, and variable spread of blood throughout the entire ventricular system. A characteristic pathologic finding is a mass of clot within the subarachnoid spaces at the base of the brain (Figure 19-4), and extension of blood through the subarachnoid pathways into the sylvian fissure and over the convexities of the developing hemispheres.
Serial cranial sonograms suggest that as many as 10–20 percent of preterm infants with GMH develop intraparenchymal abnormalities [Fanaroff et al., 2003]. The neuropathologic correlates of these abnormalities are most easily understood as arising not only from the acute increase in intracranial pressure attributable to the GMH itself, but also from the profound decreases in regional blood flow that may follow it. In 10–20 percent of infants with extensive GMH, the branches of the terminal vein that typically drain through the area of hemorrhage become grossly congested, and venous hemorrhagic infarction of the periventricular white matter and even cortical tissues may occur during the first several days after IVH [Pape and Wigglesworth, 1979]. These periventricular white matter infarctions are frequently termed intraparenchymal echodensities (IPE) when examined by cranial ultrasonography or MRI. In addition, some investigators believe that the parenchymal involvement of IVH readily visible by cranial ultrasonography represents a direct pressure-mediated extension of hemorrhage from either a massive GMH or blood within the ventricular system, but the close clinical association of ischemia and hemorrhage and the timing of the development of intraparenchymal echodense lesions on ultrasonography suggest that these lesions represent venous infarction of the periventricular white matter. These lesions are difficult to distinguish at the time of postmortem examination and have been variously named hemorrhagic intracerebral involvement, parenchymal involvement of hemorrhage, or grade 4 IVH. As proposed by Volpe, while GMH may result in the destruction of the germinal matrix and loss of neuronal and glial cells residing there, extension of hemorrhage into the SVZ and periventricular white matter is associated with not only the loss of additional precursor cells but also axonal and glial necrosis [Volpe, 2009a].
PHH represents a not uncommon sequela of IVH [Whitelaw, 2001; Whitelaw et al., 2004]. The meninges are thickened and show an infiltration by hemosiderin-laden macrophages [Deonna et al., 1975; Larroche, 1972]. These findings result in occlusion of the arachnoid villi, obstruction to flow of cerebrospinal fluid (CSF) through the foramina of Luschka and Magendie, and impairment of flow through the tentorial notch (see Figure 19-4). Occasionally, aqueductal obstruction is caused by an acute blood clot, ependymal disruption, or reactive gliosis [Cherian et al., 2004; Whitelaw, 2001].
Neuroimaging
Intraventricular Hemorrhage
Routine cranial ultrasonography via the anterior fontanel is performed in neonatal intensive and special care units around the world, and this modality represents the method of choice for the diagnosis and monitoring of IVH and its complications in preterm infants. Most nurseries use the standard grading system found in Table 19-1, which was originally developed for computed tomography (CT) scanning but fairly quickly applied to ultrasonography (Figure 19-5) [Papile et al., 1978]. Although grade 4 hemorrhages (shown in Figure 19-6) have been noted within the first 6 postnatal hours, most infants initially experience low-grade hemorrhage, which can be seen to progress over the course of time by ultrasonography. The most common sites for parenchymal hemorrhage are the frontal and parietal regions; approximately 50 percent of such hemorrhages occur bilaterally [Shalak and Perlman, 2002].
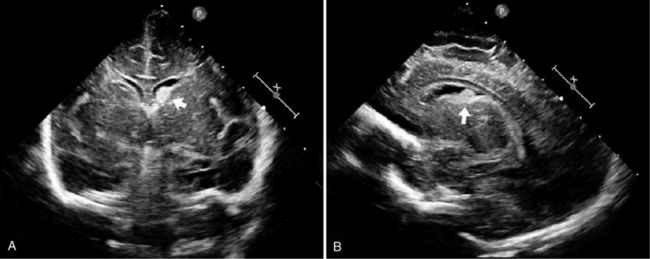
Fig. 19-5 Germinal matrix hemorrhage (GMH).
(Courtesy of Walter C Allan, M.D., Maine Medical Center, Portland, ME.)
Intraparenchymal Echodensities
Cranial ultrasonography and MRI are also used for the diagnoses of IPE and porencephaly. IPE has been described as an asymmetric white matter lesion that may accompany the onset of IVH or follow it. Rarely found without GMH or IVH, IPE is believed to represent hemorrhagic venous infarction of the periventricular white matter and generally occurs in infants of the youngest gestational ages during the first postnatal week [de Vries et al., 2001]. The ultrasound appearance, found in Figure 19-7, is an echogenic juxtaventricular white matter lesion superior and lateral to the lateral ventricle and extending toward the cortex in a flare pattern without evidence for mass effect on the surrounding brain. Most often, IPE eventually cavitates or atrophies to form either a porencephaly or adjacent ex vacuo ventriculomegaly [De Vries et al., 2004].
Improvements in MRI technology and the use of special MRI-compatible incubators and ventilators have permitted MR imaging of hemorrhage in the developing brain. In the newborn period, MR studies demonstrate a single hemorrhagic lesion adjacent to or communicating with the lateral ventricle in addition to IVH [Dyet et al., 2006] (Figure 19-8).
Porencephaly
Porencephalies (Greek for “holes in the brain”) are hemispheric cavitary lesions. In neonates, porencephalies follow grade 4 hemorrhages, IPEs, or periventricular venous infarctions. Although most porencephalies are freely communicating with the ventricular system, a porencephaly may present as a fluid-filled cyst that causes increased intracranial pressure in the preterm infant. A typical porencephaly is shown by ultrasound and MRI in Figure 19-9.
Clinical Findings
Incidence
While the incidence of IVH depends on a number of factors previously discussed, most centers report that 20–25 percent of VLBW neonates experience IVH, and 6 to more than 20 percent experience grades 3–4 IVH [Fanaroff et al., 2007; Zeitlin et al., 2009]. The incidence of IVH is inversely related to birth weight and gestational age [Sarkar et al., 2009], and data for 18,153 neonates of 501–1500 g birth weight from the National Institute of Child Health and Human Development (NICHD) Neonatal Network between 1 January 1997 and 12 December 2002 suggest that 24 percent of infants of 501–750 g birth weight, 14 percent of infants of 751–1000 g birth weight, 9 percent of those with 1001–1250 g birth weight, and 5 percent of infants with birth weights between 1251 and 1500 g experienced grades 3–4 IVH [Fanaroff et al., 2003, 2007]. Grades 3–4 IVH are more common in male neonates [Leijser et al., 2009]; when Synnes evaluated 3772 neonates of <33 weeks gestational age, 317 (8.3 percent) were found to have grade 3–4 IVH. In this cohort, male gender was an independent and important predictor of IVH (OR = 1.5, 95 percent CI = 1.1–1.9) [Synnes et al., 2006].
Timing of IVH
The risk period for IVH for preterm infants is the first 4–5 postnatal days [Ment et al., 2002; Perlman and Rollins, 2000]. Over 50 percent of all hemorrhages are detectable within the first 6 postnatal hours, and the work of Shaver suggests that almost all of these early hemorrhages are in fact detectable within the first postnatal hour [Shaver et al., 1992]. Less than 5 percent of hemorrhages occur after the fourth or fifth postnatal day (see Figure 19-9). The risk period for IVH is independent of gestational age, and between 10 and 65 percent of infants experience progression, or extension, of their hemorrhages over the first several postnatal days [Whitelaw, 2001]. Extension of IVH and the development of high-grade IVH are twice as common in those infants with the earliest onset of IVH. This progression of hemorrhage has been linked to known clinical events, such as pneumothoraces and seizures, which have been demonstrated to increase cerebral blood flow [du Plessis, 2008].
Clinical Manifestations
The clinical manifestations of IVH are variable, and in one large series of postmortem examinations, over 75 percent of hemorrhages were clinically unrecognized [Allan and Sobel, 2004; Perlman and Rollins, 2000; Volpe, 1997]. None the less, as shown in Table 19-5, infants with large hemorrhages generally are noted to suffer a profound decrease in peripheral hematocrit and may experience coma, seizures, abnormal eye findings, including dilated and nonreactive pupils and loss of eye movements, and changes in tone and reflexes [Dubowitz et al., 1998]. Apneic and bradycardic spells may be attributable to either increased intracranial pressure or changes in cerebral blood flow to brainstem respiratory control centers. Of note, infants with even low-grade IVH may have significantly elevated serum glucose values and evidence for inappropriate secretion of antidiuretic hormone [Moylan et al., 1978; Shalak and Perlman, 2002]. Finally, persistent, unremitting metabolic acidosis unresponsive to alkali therapy or pressor agents is the hallmark of high-grade hemorrhage.
Table 19-5 Clinical Symptoms of High-Grade Intraventricular Hemorrhage
Symptom | Incidence |
---|---|
Decrease in hematocrit | 75% |
Seizures | 10–15% |
Changes in tone | 75–90% |
Abnormal eye signs | 33–95% |
Apnea/bradycardia | 50–75% |
Hyperglycemia | >50% |
Hyponatremia | >50% |
Metabolic acidosis | >75% |
Ment unpublished data, NS27116 Randomized Indomethacin IVH Prevention Trial, 1997.
Neonatal Outcome
In the neonatal period, infants with IVH are at risk for the development of seizures and PHH [Allan and Sobel, 2004; Whitelaw et al., 2004]. Depending on the birth weights and gestational ages of the cohorts studied and the investigator’s method for determining seizures, the incidence of seizures in preterm infants with IVH has been reported to range from 5 to 45 percent. Of note, a recent continuous amplitude-integrated EEG (aEEG) study of preterm neonates suggests that the mean age of seizure detection is 28 hours in infants with IVH, and only 2 of 8 neonates with IVH and seizures detected by aEEG had clinically recognizable events [Shah et al., 2010].
Posthemorrhagic hydrocephalus
Posthemorrhagic hydrocephalus, shown by ultrasound in Figure 19-10, is diagnosed in the infant who meets all of the following criteria: presence of intraventricular blood, increasing ventriculomegaly (most commonly diagnosed by cranial ultrasonography), and evidence of increased intracranial pressure (defined as an opening pressure of greater than 140 mm H2O by either lumbar puncture or, if indicated, cerebral ventricular tap) [McCrea and Ment, 2008; Whitelaw, 2001; Whitelaw et al., 2004]. In addition, in infants with PHH, the ventricular width at the intraventricular foramen by sonographic measurement exceeds 4 mm over the 97th percentile for gestational age [Levene, 1981]. PHH is most common in those infants with high grades of hemorrhage and less frequent in infants with the lowest gestational ages [Kazan et al., 2005]. Because of the compliance of the neonatal brain, the signs and symptoms of hydrocephalus may not be evident for several weeks following hemorrhage, and routine ultrasonographic monitoring is recommended in those infants at risk for PHH.
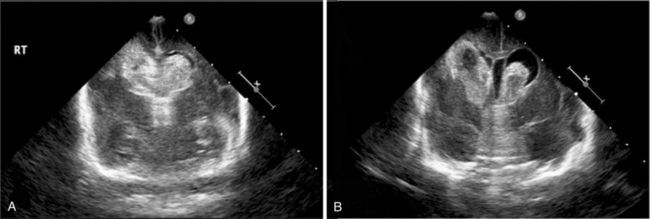
Fig. 19-10 Intraventricular hemorrhage leading to posthemorrhagic hydrocephalus.
(Courtesy of Walter C Allan, MD, Maine Medical Center, Portland, ME.)
In most instances, the sequence of events resulting in PHH begins with multiple small clots throughout the ventricles. Although PHH is traditionally thought to represent a communicating hydrocephalus with obstruction to the passage of CSF at the level of the arachnoid villi, it is also probable that the CSF is reabsorbed across the ependyma into small penetrating vessels within the developing white matter. Obstruction to flow may thus also occur at the level of these vessels, or less commonly, at the foramina of Luschka and Magendie in the posterior fossa [Cherian et al., 2004]. In the presence of an obstruction in the normal CSF flow pathways, ventriculomegaly develops and periventricular white matter damage may ensue. Suggested mechanisms for periventricular white matter injury following PHH include raised pressure and edema, damaging effects of the free iron released into the CSF during clot lysis, and/or proinflammatory cytokines [Savman et al., 2001]. In addition, a small percentage of infants with IVH may develop acute obstruction at either the foramen of Monro, as shown in Figure 19-11, or the aqueduct secondary to the presence of clots obstructing CSF flow; these infants present with signs of acutely increased intracranial pressure (i.e., apnea, bulging fontanel, split sutures, and lethargy or coma). These infants require immediate neurosurgical attention, as shown in Video 19-1.
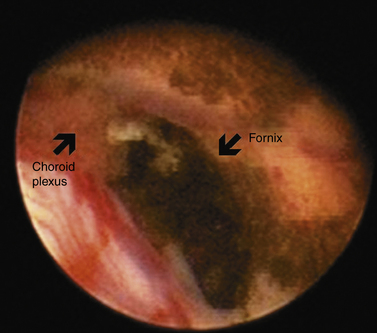
Fig. 19-11 Endoscopic view of the foramen of Monro.
(Courtesy of Charles C Duncan, MD, Department of Neurosurgery, Yale University School of Medicine, New Haven, CT.)
Randomized controlled trials have evaluated several treatment strategies either to prevent or to reduce the extent of PHH. These include intraventricular streptokinase, repeated lumbar or ventricular punctures, and DRIFT (drainage, irrigation and fibrinolytic therapy), but none of these has yet proved effective [Shooman et al., 2009; Whitelaw, 2000a, 2000b; Whitelaw and Odd, 2007; Whitelaw et al., 2001, 2007].
When treating infants with PHH, the physician must both protect the neonate from damage secondary to increased intracranial pressure and avoid the need for permanent CSF drainage procedures. (For review see Shooman et al. [2009].) Infants with intraventricular and/or intraparenchymal hemorrhage should undergo frequent determinations of occipitofrontal circumference and ultrasonographic determinations of ventricular size. Because prolonged increased intracranial pressure may result in poor feeding, lethargy, apnea, and, ultimately, optic atrophy, if ventricular size increases, neurosurgical consultation is recommended. Further, although some investigators have recommended ventricular puncture with the acute removal of 10–20 mL/kg of CSF for symptomatic infants with PHH, others have explored temporizing measures, such as subgaleal shunt placement, ventricular reservoir placement for intermittent tapping, or third ventriculostomy (Video 19-2). These strategies aim to avoid permanent ventriculoperitoneal shunt placement, and a small retrospective review of these interventions in neonates with IVH reported that 91 percent of those infants with subgaleal shunt placement and 62 percent of those with reservoir taps required permanent shunt placement. Of importance, infection rates were similar in the two populations, but future randomized trials are required to explore these treatment options.
Long-Term Outcome
Although recent advances in neonatal intensive care have resulted in increased survival rates for many critically ill and very preterm neonates, the neurodevelopmental fate of VLBW infants remains uncertain. Depending on the birth weight of the patient cohort and the years in which they were born, the incidence of minor developmental impairment is diagnosed in 30–40 percent and major disabilities are found in almost 20 percent of preterm children [Allen, 2008; Larroque et al., 2008; Neubauer et al., 2008; Saigal and Doyle, 2008; Voss et al., 2007]. Over half require special assistance in the classroom, 20 percent are in special education, and 15 percent have repeated at least one grade in school [Aylward, 2005; Bhutta et al., 2002]. Cerebral palsy has long been recognized as a frequent sequela of preterm birth, but significant cognitive and behavioral abnormalities are also being recognized at school age and beyond [Allen, 2008; Hack et al., 2009; Marlow et al., 2005; Robertson et al., 2009; Taylor et al., 2004].
Cerebral Palsy
Cerebral palsy (CP) is an important and well-established outcome of preterm infants that, in some centers, has been monitored for more than 45 years [Saigal and Doyle, 2008]. Recent data suggesting that the prevalence of neurodevelopmental handicap is increasing in the United States have attributed this finding to the increasing survival rates of many preterm and VLBW infants [Allen, 2008; Behrman and Stith Butler, 2007; Larroque et al., 2008].
Several recent studies have assessed the incidence of CP in children solely with IVH. In all, the incidence of CP is reported to increase with the grade of IVH. In pre-surfactant era studies, Pinto Martin reported that grade 4 IVH was strongly associated with CP (odds ratio = 15.4; 95 percent CI = 7.6–31.1), and in the study of Hansen and colleagues, the odds ratio for CP with grades 3 or 4 IVH was 19.9 (CI = 6.1–64.8) [Hansen et al., 2004; Pinto-Martin et al., 1999]. Further, in Pinto-Martin’s study, any grade IVH alone was associated with CP (odds ratio = 3.14; 95 percent CI = 1.5–6.5).
More recently, Sherlock evaluated 270 neonates of <1000 g birth weight or <28 weeks gestational age at 8 years of age and found CP in 100 percent of those children with grade 4 IVH [Sherlock et al., 2005, 2008]. In contrast, approximately one-fifth of neonates with grades 2 or 3 IVH developed CP, and those with grade 1 hemorrhage experienced no increase in incidence of motor handicap when compared to children with no IVH. Similarly, Patra examined 362 children with birth weights <1000 g at 20 months of age; 104 had experienced grade 1–2 IVH [Patra et al., 2006]. Abnormal neurological examinations, including CP, were significantly more common in the IVH group (odds ratio = 2.60, 95 percent CI = 1.06–6.36).
Although most investigators include the results of all scans performed during the infant’s hospitalization, Allan has reported that CP can be predicted from postnatal day 3 onward in preterm infants in whom serial ultrasonography is performed [Allan et al., 1997]. Further, although preterm infants with CP have traditionally been found to suffer spastic diplegia, concomitant with the rising rate of disabling spastic motor handicap, several investigators have also noted that CP among preterm infants is almost equally divided among the three classical forms: spastic diplegia, hemiplegia, and quadriplegia [Allan et al., 1997; Saigal and Doyle, 2008]. It is believed that this change in distribution reflects more focal and diffuse CNS injuries producing proportional increases in hemiplegias and quadriplegia.
Cognitive Outcome in Neonates with Intraventricular Hemorrhage
IVH is a significant cause of disability in the prematurely born. As shown in Figure 19-12, preterm subjects with grade 4 IVH have significantly worse cognitive abilities and educational performance when compared to those without IVH, as well as to those with lesser grades of hemorrhage [Luu et al., 2009a; Neubauer et al., 2008]. In Sherlock’s study of 298 infants with birth weight <1000 g or gestational age <28 weeks, 100 percent of children with grade 4 IVH had full-scale IQ scores more than 1 SD below the population mean, compared to 60 percent of subjects with grade 3 IVH and approximately 40 percent of all other study participants (no IVH, grade 1 IVH, and grade 2 hemorrhage) [Sherlock et al., 2005, 2008]. Similarly, in Patra’s study of 362 preterm neonates of the same birth weight, 45 percent of children with grades 1–2 IVH had mental scores >2 SD below the population mean, compared to 25 percent for those with no evidence of hemorrhage in the newborn period (odds ratio = 2.0, 95 percent CI = 1.20–3.60) [Patra et al., 2006].
Alterations in Brain Development
The advent of MRI has permitted assessment of the long-term influence of a neonatal injury such as IVH on the developing brain [Mewes et al., 2006; Nosarti et al., 2002; Reiss et al., 2004; Woodward et al., 2006]. Preterm children have smaller brain volumes at adolescence and beyond, and lower cortical gray, cortical white, deep gray, and cerebellar volumes when compared to term controls. In addition, IVH has been associated with significant additional reductions in cerebral gray volumes in preterm subjects during childhood and adolescence [Kesler et al., 2008; Nosarti et al., 2008; Reiss et al., 2004; Vasileiadis et al., 2004]. Studying the largest cohort of subjects born preterm at age 14 years and comparing them with age- matched and socioeconomically matched term adolescents, Nosarti also noted widespread alterations in white matter volumes throughout the developing hemispheres [Nosarti et al., 2008