Chapter 1 Fluorescein Angiography
Basic Principles and Interpretation
For additional online content visit http://www.expertconsult.com
For nearly 50 years, fundus photography and fluorescein angiography have been valuable in expanding our knowledge of the anatomy, pathology, and pathophysiology of the retina and choroid.1 Initially, fluorescein angiography was used primarily as a laboratory and clinical research tool; only later was it used for the diagnosis of fundus diseases.1–5 An understanding of fluorescein angiography and the ability to interpret fluorescein angiograms are essential to accurately evaluate, diagnose, and treat patients with retinal vascular and macular disease.
Basic principles
Fluorescence
This is a fundamental principle of fluorescein angiography. In the procedure, the patient, whose eyes have been dilated, is seated behind the fundus camera, on which a blue filter has been placed in front of the flash. Fluorescein is then injected intravenously. Eighty percent of the fluorescein becomes bound to protein and is not available for fluorescence, but 20% remains free in the bloodstream and is available for fluorescence. The blue flash of the fundus camera excites the unbound fluorescein within the blood vessels or the fluorescein that has leaked out of the blood vessels. The blue filter shields out (reflects or absorbs) all other light and allows through only the blue excitation light. The blue light then changes those structures in the eye containing fluorescein to green-yellow light at 520–530 nm. In addition, blue light is reflected off the fundus structures that do not contain fluorescein. The blue reflected light and the green-yellow fluorescent light are directed back toward the film of the fundus camera. Just in front of the film a filter is placed that allows the green-yellow fluorescent light through but keeps out the blue reflected light. Therefore the only light that penetrates the filter is true fluorescent light (Fig. 1.1).
Pseudofluorescence
Pseudofluorescence occurs when nonfluorescent light passes through the entire filter system. If green-yellow light penetrates the original blue filter, it will pass through the entire system. If blue light reflected from nonfluorescent fundus structures penetrates the green-yellow filter, pseudofluorescence occurs (Fig. 1.2). Pseudofluorescence (i.e., fake fluorescence) causes nonfluorescent structures to appear fluorescent. It can confuse the physician interpreting the fluorescein angiogram and lead him or her to think that certain fundus structures or materials are fluorescing when they are not. Pseudofluorescence also causes decreased contrast, as well as decreased resolution. Because fluorescein angiography uses black-and-white film, the nonfluorescent or pseudofluorescent light appears as a background illumination. The background illumination from pseudofluorescence is especially heightened if there are white areas of the fundus, such as highly reflective, hard exudates. Pseudofluorescence must be avoided. Therefore the excitation (blue) and barrier (green-yellow) filters should be carefully matched so that the overlap of light between them is minimal.
Equipment
Film-based versus digital fluorescein angiography – historical perspectives
Fluorescein angiography finds its origins in the late 1960s with the publication of an original article describing its use as well as subsequent atlases and textbooks for a medical retinal specialty in its infancy.1,6 The landmark text Atlas of Macular Diseases by Dr J. Donald Gass set a new standard for the use of stereoscopic fluorescein angiography in fundus diagnosis.7
As digital photography has evolved with improved resolution, the convenience of digital-based fluorescein angiography has gained wider acceptance. Though film-based images offer the highest amounts of resolution and 35-mm negatives are often easier to view for stereo, images are relatively difficult to manipulate, and training and effort are required to process and duplicate film. Transmitting or sharing film-based images is also time-consuming compared with digital images (Box 1.1).8
Box 1.1
Equipment and materials needed for angiography
Fundus camera and auxiliary equipment
Matched fluorescein filters (barrier and exciter)
Digital photoprocessing unit (computer-based) and software user interface
5 mL of l0% fluorescein solution
20-gauge, -inch needle to draw the dye
Camera and auxiliary equipment
Cameras differ in the degree of fundus area included in the photographs. Fundus cameras may range from 35° to 200° wide-field camera systems such as Optos.9,10 In clinical retinal practice, cameras ranging from 35° to 50° are routinely used (Fig. 1.3). Regardless of range, a camera with the ability to yield high resolutions of the posterior pole is essential for most macular problems, especially when laser treatment is to be done, as with background diabetic retinopathy, branch-vein occlusion, or choroidal neovascularization.
Wide-angle angiography has the benefit of capturing a single image of the retina in high resolution well beyond the equator. The potential for clinical efficiency and sensitivity in detecting neovascularization in the far periphery as well as acquiring an excellent clinical picture of the degree of capillary retinal nonperfusion is an exciting development in fluorescein angiography (Fig. 1.4).
Fluorescein solution
Various side-effects and complications can occur with fluorescein injection (Box 1.2).11–15
Box 1.2
Side-effects and complications of fluorescein injection
Extravasation and local-tissue necrosis13
Inadvertent arterial injection
Vasovagal reaction (circulatory shock, myocardial infarction)12
Vomiting occurs infrequently, affecting only 0.3–0.4% of patients.11,13 When it does occur, it usually begins 40–50 seconds after injection. By this time most of the initial-transit photographs of the angiogram will have been taken. A receptacle and tissues should be available in case vomiting does occur. When patients experience nausea or vomiting, they must be reassured that the unpleasant and uncomfortable feeling will subside rapidly. Photographs can be taken after the vomiting episode has passed. A slower, more gradual injection may help to prevent vomiting.
Technique
Aligning camera and photographing
The photographer moves the camera from side to side to ascertain the width of the pupil and the focusing peculiarities of the particular cornea and lens. The photographer studies the eye through the camera lens, moving the camera back and forth and up and down, looking for fundus details (e.g., retinal blood vessels). The photographer then determines the single best position from which to photograph (Figs 1.6 and 1.7).
Any abnormalities, such as an unusual light reflex or a poorly resolved image the photographer sees through the camera system, will appear on the photograph. If the ophthalmoscopic view seen through the camera is not optimal, the photograph will not be optimal (Fig. 1.8). If the view is optimal, well aligned, in focus, and without reflexes, the photograph can be optimal. A helpful concept for the photographer is “what you see is what you get (or worse – never better).”
Focusing
The photographer first turns the eyepiece counterclockwise (toward the plus, or hyperopic, range) to relax his or her own accommodation; this causes the crosshairs to blur. The photographer then turns the eyepiece slowly clockwise to bring the crosshairs into sharp focus. The eyepiece is focused properly when the crosshairs appear sharp and clear (Fig. 1.9, online). They must remain perfectly clear while the photographer focuses on the fundus with the camera’s focusing detail. With experience, the photographer becomes expert in adjusting the eyepiece and in keeping the crosshairs in focus throughout the entire photographic sequence.
Digital angiography
In theory, film-based photography has advantages over digital imaging: image resolution and stereoscopic viewing.8 Film-based images contain 10 000 lines of resolution, in contrast to digital imaging, which may have as little as 1000 lines of resolution.8 However, some argue that, despite higher resolution in film, the greater ability to magnify digital images makes the disadvantage of digital photography less clinically relevant.8 Digital angiography offers advantages, including the instantaneous availability of the angiogram, and the avoidance of the equipment and time necessary to develop film. With instantaneous images, digital angiography facilitates education and discussion concerning the patient’s condition and treatment options. Also, digital angiography facilitates training of ophthalmic personnel. We have found that it is useful to stay in the room during the initial frames of the angiogram to ensure that the desired pathology is photographed. Any changes can be promptly made, and the photographer can also learn from this prompt feedback. Digital angiography, however, necessitates an ongoing investment of money both in software updates and storage of digital electronic files. Also, excessive image manipulation with image-editing software may result in artifacts. Specifically, some areas may appear overly hyperfluorescent due to limited dynamic range in images and software manipulation. Care should be taken in avoiding misinterpretation of hyperfluorecence and hypofluorescence in digital images.
Using stereophotography
Stereophotography separates, photographically, the tissues of the eye for the observer. Stereo fluorescein angiography facilitates interpretation by separating in depth the retinal and choroidal circulation.16,17 Stereo angiography is considered absolutely essential in certain situations.18 The photographic protocol for the Macular Photocoagulation Study required stereo fluorescein angiography. Without well-resolved stereo images, interpretation of angiograms with, for instance, choroidal neovascularization associated with age-related macular degeneration, can be extremely difficult, if not impossible (Fig. 1.10, online). On the other hand, stereophotography, although extremely helpful in cases that are difficult to interpret, is not always absolutely necessary because other fundus features and characteristics usually indicate the level at which abnormal fluorescence is located.
Photographing the periphery
Photographing the peripheral retina with a standard 50° fundus camera demands precision and skills acquired only after many hours of practice. Problems with patient position and camera alignment and focus are compounded by marginal corneal astigmatism, unsteadiness of patient fixation, light reflexes, and awkward camera placement. All steps necessary for taking posterior photographs, such as alignment and focusing, must be employed to achieve good peripheral fundus photography. The Zeiss camera comes with an astigmatic dial to help neutralize the induced astigmatism. A tilt mechanism, now standard on most cameras, helps position the camera for extreme superior and inferior peripheral photography (Fig. 1.11).
Positioning the patient
The patient is positioned at the camera with the chin in the chinrest and the forehead against the head bar. Because the most common cause of poor fluorescein photographs is involuntary movement of the patient’s head, the photographer should prepare and make adjustment for this before the fluorescein is injected. The photographer should aim and focus the camera on the specific area of primary interest, at the same time noting the patient’s responses. If the photographer finds that the camera must continually be moved closer to the patient while aligning it or taking preliminary photographs, or if reflexes suddenly appear in the view even though the camera is steady, then the patient’s head has moved away from the chinrest. If so, the photographer can make some adjustment before injecting the fluorescein dye. Sometimes having an assistant hold the patient’s head in the chinrest is helpful (Fig. 1.12, online). The photographer either may lower the entire camera and chinrest or raise the patient’s chair. This causes the patient to lean forward in the chinrest and against the forehead bar, making it more difficult for the patient to pull back.
Injecting the fluorescein
The color stereoscopic fundus photographs are taken first, before the fluorescein is injected. For injection, we recommend a syringe with a 23-gauge scalp-vein needle (Fig. 1.13, online). The scalp-vein needle has several advantages: it is small enough to enter most visible veins, and an intravenous opening is then available in the event of an emergency. Once in the vein, it requires no further attention, and although it can be taped in place, this usually is unnecessary. Whenever an antecubital vein is not visible or accessible, the vein in the back of the hand or radial (thumb) side of the wrist can usually be used for injection. Injecting the fluorescein into a hand or wrist vein increases the circulation time by a few seconds, but this seldom makes any difference.
Developing a photographic plan
To photograph and print the fluorescein angiogram, we suggest the following comprehensive plan, designed to yield maximal angiographic information from each fundus and to facilitate a thorough and complete interpretation (Fig. 1.14). In contrast to film-based angiograms which required multiple duplicate attempts to assure at least one image would be optimal, fewer photographs are necessary in digital angiography. Although most angiograms will be complete by following this procedure, there will be exceptions. This plan must be modified if abnormalities occur in areas other than the macula and disc.
It is both essential and extremely cost-effective for the physician to indicate specifically what areas to photograph. The photographer should be directed as to where to start the angiogram and the issues important for each specific angiogram. It is most efficient to use a photographic instruction slip that indicates the specific number of color photographs to take of each area, where to start the angiogram, what the diagnosis is, and any other information about the patient or fundus that is pertinent to the photographic process (Fig. 1.15, online). Although digital color and angiograms avoid the issue of wasted film and developing costs, unnecessary computer storage of images and patient inconvenience can be avoided with good technique and a repeatable, accurate algorithm for angiography.
At this point the fluorescein injection is begun. The needle is inserted in a vein in the patient’s arm (Fig. 1.16, online). The photographer waits for confirmation of successful venous access and awaits verbal confirmation that infusion is about to begin. Once the injecting clinician starts the infusion of fluorescein, the photographer begins the initial “injection” image. When the injecting clinician has completed infusion, he or she announces “injection complete” and the photographer takes the “end-of-injection” image. Because it is important to observe the site of the needle tip for extravasation of fluorescein, the lights are turned off only at the end of the injection. An alternative method is to turn the lights off after the needle has been inserted in the vein. The person injecting can hold a hand light to observe the fluorescein flow into the vein to be sure extravasation is not occurring. With the lights off, the photographer can become dark-adapted, which allows him or her to be better able to see the flow of fluorescein into the fundus as it occurs.
Box 1.3 provides a checklist of important steps in the fluorescein angiography procedure.
Box 1.3
Checklist for fluorescein angiography
• Inform patient about fluorescein angiography. Obtain written informed consent
• Dilate patient’s pupil if necessary
• Prepare fluorescein solution, scalp-vein needle, and syringe
• Input patient identification and demographic data in computer database
• Position patient for alignment, focus, and comfort
• Take red-free photos if indicated
• Simultaneously start timer from zero and inject fluorescein dye
• Take preinjection photograph: these serve as controls in detecting auto- and pseudofluorescence
• Shoot at exact start of injection as timer is turned on and shoot second shot at exact finish of injection (length of time of injection is automatically recorded)
• Start fluorescein photograph 8 seconds after the start of injection in young patients and 12 seconds in older patients
• Follow fluorescein angiography plan
• When photography is done, reassure patient that urine will be discolored for a day or so
• At the discretion of the physician, have patient wait for observation for possible reactions to fluorescein
Interpretation
Fundus anatomy and histology
1. preretinal area, where contraction from an epiretinal membrane may influence the retinal circulation and where hemorrhage may be located
2. vascular layers of the sensory retina, both superficial and deep
3. avascular portion of the sensory retina, particularly the outer plexiform layer, the principal site of intraretinal edema and exudate
4. retinal pigment epithelium, which has the potential for many manifestations, including proliferation, depigmentation, hyperpigmentation, and detachment
5. choroidal circulation, including the choriocapillaris and the large choroidal vessels
Throughout this chapter a modified schematic drawing relates various fluorescein angiographic abnormalities to fundus histopathologic changes (Fig. 1.17). The size and proportion of these various layers have been modified to include various pathologic manifestations and to illustrate the effects of these abnormalities on the angiogram. Because of its importance and various pathologic changes, the pigment epithelium is drawn to a larger scale in relation to other fundus structures. Only the inner portion of the sclera is represented because the outer portion of the sclera is usually of little importance to angiographic interpretation. The retinal and choroidal vessels are drawn larger and more numerous than they appear in a normal histopathologic section to emphasize the contribution of circulatory pathophysiologic interpretation.
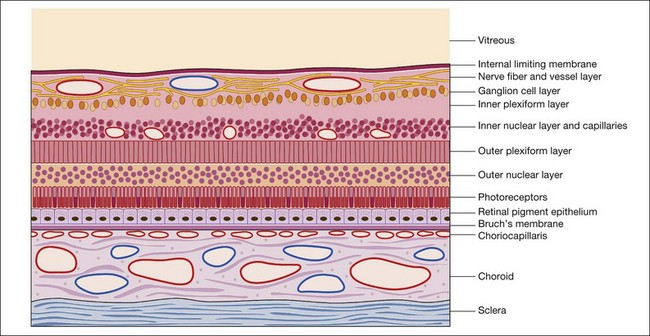
Fig. 1.17 Modified schematic drawing of a microscopic section of retina, pigment epithelium, and choroid.
Two specialized areas of the fundus warrant more detailed discussion: the macula (Fig. 1.18) and the optic nerve head. The fovea is the center of the macula and contains only four layers of the retina: (1) the internal limiting membrane; (2) the outer plexiform layer; (3) the outer nuclear layer; and (4) the rods and cones. No intermediate layers exist between the internal limiting membrane and the outer plexiform layer in the fovea, which in the macula is oblique. This is an important factor in understanding the stellate appearance of cystoid edema in the macula as opposed to the honeycomb appearance of cystoid edema outside the macula. Beyond the macular region the outer plexiform layer is perpendicular rather than oblique.
Abnormal fluorescein angiogram
The purpose of this section is to offer a schema by which the interpretation of the fluorescein angiogram follows a simple and logical progression. The first step is to recognize areas of abnormal fluorescence and determine if they are hypofluorescent or hyperfluorescent (Fig. 1.20).
Hypofluorescence
Hypofluorescence is a reduction or absence of normal fluorescence, whereas hyperfluorescence is abnormally excessive fluorescence. A systematic series of decisions follows this initial differentiation to arrive at a proper diagnosis. These decisions relate to: (l) the anatomic location of various abnormalities; (2) the quality and quantity of the abnormal fluorescence; and (3) other unique characteristics, as indicated in Fig. 1.20.
Blocked retinal fluorescence
Any translucent or opacified material in the retina or in the nerve fiber layer blocks fluorescence from both planes of retinal vessels, as well as from the choroidal vessels. The large retinal vessels and precapillary arterioles are located in the nerve fiber layer in the anterior plane of the retina. The capillaries and postcapillary venules are located deeper in the retina, in the inner nuclear layer. If a blocking material lies in front of the nerve fiber layer, it blocks both planes of retinal vessels (Fig. 1.21). However, if the material lies beneath the nerve fiber layer but within or in front of the inner nuclear layer (where the smaller retinal vessels are located), it blocks only the retinal capillaries (and choroidal vessels), leaving the view of the large retinal vessels unobstructed. If a blocking material lies deeper than the retinal vascular structures, deep to the inner nuclear layer, it does not block the vessels but will block the choroidal vascular fluorescence. In other words, deep intraretinal blocking material, such as hemorrhage or exudate, does not obstruct retinal vascular fluorescence, since the retinal vessels are located in the inner half of the retina (Fig. 1.22).
If blocking material is present, the next step is to determine its anatomic location.
Blocked choroidal fluorescence
Hypofluorescence caused by blocked choroidal vasculature occurs when fluid, exudate, hemorrhage, pigment, scar, inflammatory material, or the like accumulates in front of the choroidal vasculature and deep to the retinal vasculature (Fig. 1.23).
Subretinal material
Any opaque or translucent substance located beneath the retina but in front of the choroid blocks fluorescence of the choroidal vasculature but does not block retinal vascular fluorescence (Fig. 1.23). Blood located under the retina causes complete blockage of choroidal fluorescence, with the retinal fluorescence showing through normally. Subretinal hemorrhage appears red, and subpigment epithelial hemorrhage is dark. Subretinal hemorrhage is generally scalloped with somewhat irregular margins, whereas subpigment epithelial hemorrhage is often quite round and well demarcated (Fig. 1.23).
Accumulated pigment (melanin and lipofuscin) from diseased retinal pigment epithelium causes blocked choroidal fluorescence (Fig. 1.24). Any hyperpigmentation of the pigment epithelium causes blocked choroidal fluorescence. Xanthophyll, the pigment present in the outer layers of the fovea, blocks choroidal fluorescence by selectively absorbing the blue exciting light, which results in less fluorescence. Finally, a choroidal nevus may block much of the choroidal fluorescence (Fig. 1.25) and especially blocks the later hyperfluorescent staining of the sclera. The choriocapillaris may be seen normally over the nevus.
Retinal vascular filling defect
If a retinal vascular filling defect is present, the clinician then considers whether the defect results from obstruction of a retinal artery or vein, capillary bed, or any combination of these. Distinguishing the cause of the obstruction is not difficult because the fluorescein angiographic process is dynamic and timed. When nonfilling of a specific retinal vessel occurs, it is easy to differentiate an arterial occlusion from a venous occlusion because the retinal arteries fill first, then the retinal capillary bed, followed by the retinal veins. In addition, retinal vascular filling defects can be localized by tracing the course of a particular vessel; these defects correspond anatomically to the normal distribution of the retinal vasculature (Figs 1.26 and 1.27). Thus retinal vascular filling defects result from a variety of disease processes, but most are commonly associated with atherosclerosis and diabetes.
Vascular filling defects of the disc
Vascular filling defects of the disc occur because of the failure of the capillaries of the optic nerve head to fill. This failure can be caused by: (1) congenital absence of disc tissue, as in an optic pit or optic nerve head coloboma (Fig. 1.28); (2) atrophy of the disc tissue and its vasculature, as in optic atrophy; or (3) vascular occlusion, as in an ischemic optic neuropathy.19,20 Each condition is characterized by early hypofluorescence caused by nonfilling and late hyperfluorescence resulting from staining of the involved tissue.
Choroidal vascular filling defect
When choroidal vessels do not fill, dark patches of hypofluorescence beneath the retina appear early in the angiogram. The distribution and morphology of the hypofluorescence vary according to the disease process. Because the choroidal circulation is completely separate from the retinal circulation, choroidal vascular filling defects do not correlate with the retinal vascular distribution. If the choriocapillaris is absent and the large choroidal vessels are still present, the choroidal and retinal vessels fluoresce, but hypofluorescent gaps appear because of the loss of the diffuse “ground-glass” fluorescence from the choriocapillaris (Fig. 1.29). When the choroidal vasculature does not fill, as in total occlusion or in atrophy, hypofluorescence occurs early in the angiogram. The hypofluorescence remains throughout the late stages of the procedure, although leakage from surrounding areas of normal choriocapillaris extends into the occluded area. When sufficient leakage occurs, the sclera retains fluorescein (stains) late in the angiogram. When the involved area is large and the leakage is minimal, the hypofluorescence remains throughout the later stages.
In general, vascular filling defects of the choroid are caused by obstructive disorders or absence of tissue with the following fluorescein angiographic characteristics: (1) normal retinal vascular flow; (2) depigmentation of the pigment epithelium; (3) reduction of choroidal blood flow; and (4) hypofluorescence in the early phases of angiography caused by loss of the normal ground-glass choriocapillaris fluorescence. In some conditions the large choroidal vessels are also absent, resulting in total early hypofluorescence in the affected area, with scleral staining only on the circumference of the lesion because of the adjacent patent choriocapillaris. Choroidal vascular defects result from a variety of disease processes (Figs 1.30 and 1.31).
Hyperfluorescence
Autofluorescence
Autofluorescence is the emission of fluorescent light from ocular structures in the absence of sodium fluorescein. Conditions that cause autofluorescence are optic nerve head drusen and astrocytic hamartoma (Fig. 1.32).
Pseudofluorescence occurs when the blue exciter and green barrier filters overlap. The blue filter overlaps into the green range, allowing the passage of green light, or the green barrier filter overlaps into the blue range, allowing the passage of blue light (Fig. 1.2). The overlapping light passes through the system, reflects off highly reflective surfaces (light-colored or white structures), and stimulates the film. This reflected nonfluorescent light is called pseudofluorescence.
Transmitted fluorescence (pigment epithelial window defect)
This fluorescence is an accentuation of the visibility of normal choroidal fluorescence. Transmitted fluorescence occurs when fluorescence from the choroidal vasculature appears to be increased because of the absence of pigment in the pigment epithelium, which normally forms a visual barrier to choroidal fluorescence. The major cause of pigment epithelial window defect is atrophy of the pigment epithelium (Figs 1.33–1.36).
Transmitted fluorescence has the following four basic characteristics:
1. It appears early in angiography, coincidental with choroidal filling.
2. It increases in intensity as dye concentration increases in the choroid.
3. It does not increase in size or shape during the later phases of angiography.
4. It tends to fade and sometimes disappear as the choroid empties of dye at the end of angiography.
Abnormal retinal and disc vessels
Abnormal vascular fluorescence occurs when abnormal vessels are present. Such pathologic vessels may be in the retina, on the disc, or at the level of the choroid. Normal and abnormal retinal and disc vessels are clearly visible on the angiogram because no barrier obscures them from view. Gross abnormalities of the retinal and disc vasculature and subtle microvascular changes that cannot be appreciated adequately by ophthalmoscopic examination will be well defined and easily distinguished by fluorescein angiography. These changes in the retinal vasculature can be classified into six morphologic categories: (1) tortuosity and dilation (Figs 1.37 and 1.38); (2) telangiectasis (Figs 1.39 and 1.40); (3) neovascularization (Fig. 1.41); (4) anastomosis (Fig. 1.38); (5) aneurysms (Figs 1.38 and 1.39); and (6) tumor vessels (Figs 1.42 and 1.43).
Abnormal choroidal vessels
Abnormal vessels that may be present under the retina and originate from the choroid are subretinal neovascularization and vessels within a tumor. When subretinal neovascularization is present, the early angiogram often shows a lacy, irregular, and nodular hyperfluorescence (Figs 1.44 and 1.45). With a choroidal tumor, the abnormal hyperfluorescence is a similar, early vascular-type fluorescence, although it may be coarser, as seen in choroidal hemangioma (Fig. 1.46) and malignant melanoma (Fig. 1.47).
Vitreous leak
Vitreous hyperfluorescence secondary to retinal neovascularization is usually localized and appears as a cotton-ball type of fluorescence surrounding the neovascularization (Fig. 1.41B). The vitreous fluorescence secondary to intraocular inflammation is often generalized, giving a diffuse, white haze to the vitreous because of generalized leakage of fluorescein from the iris and ciliary body. The vitreous fluorescence secondary to tumors is most often localized over the tumor.
Papilledema and optic disc edema
Papilledema is swelling of the optic nerve head as a result of increased intracranial pressure. Edema of the optic disc is defined as swelling of the optic nerve head secondary to local or systemic causes (Fig. 1.48). The angiogram is similar in each case, demonstrating leakage associated with swelling of the optic nerve head. In the early phases of the angiogram, dilation of the capillaries on the optic nerve head may be seen; in the late angiogram, the dilated vessels leak, resulting in a fuzzy fluorescence of the disc margin.
Retinal leak
In the late stages of the normal angiogram, the retinal vessels have emptied of fluorescein and the retina is dark. Any late retinal hyperfluorescence is abnormal and indicates leakage of retinal vessels. When the leakage is severe, the extracellular fluid may flow into cystic pockets, and the angiogram shows fluorescence of the cystic spaces. Fluorescein flows out of the patent retinal vessels to lie in pools in the cystoid spaces or stains the edematous (noncystic) retinal tissue. Cystoid retinal edema is apparent as the fluorescein pools in small loculated pockets. In the macula, cystoid edema takes on a stellate appearance (Fig. 1.49); elsewhere in the retina, it has a honeycombed appearance (Fig. 1.50). Fluorescent staining of noncystoid edema is diffuse, irregular, and not confined to well-demarcated spaces (Figs 1.51 and 1.52).
The amount of fluorescein leakage depends on the dysfunction of the retinal vascular endothelium (Fig. 1.52). When leakage is not pronounced, the cystoid spaces fill slowly and become visible only late in angiography. When this occurs, the area of cystoid retinal edema may be somewhat hypofluorescent early in the angiogram because the fluid in these spaces acts as a barrier and blocks the underlying choroidal fluorescence. When there is heavy fluorescein leakage, the cystoid spaces fill rapidly, in some cases within a minute after injection. The large confluent cysts seen with severe cystoid macular edema may fill late in the angiogram. The large retinal vessels can also leak. This is called perivascular staining and is seen in three distinct situations: inflammation (indicating a perivasculitis), traction (severe pulling on a large retinal vessel, Fig. 1.52), and occlusion. When a large retinal vessel leak is partially occluded, or when it traverses an area of occlusion (and capillary nonperfusion), it will leak (Fig. 1.53).
Choroidal leak
Late hyperfluorescence under the retina can be classified as either pooling or staining (Fig. 1.54). Pooling is defined as leakage of fluorescein into a distinct anatomic space; staining is leakage of fluorescein diffused into tissue.
Depending on the specific disease, the late angiogram may or may not portray the full fluorescent filling of the subretinal fluid. For example, in central serous chorioretinopathy the leakage is gradual, and fluorescence of the subsensory retinal fluid will not be complete. In other conditions, such as subretinal neovascularization, fluorescein leakage is profuse, and the subsensory fluid often completely fluoresces (Fig. 1.55).
In contrast to the attachment of the sensory retina, the basement membrane of the pigment epithelium adheres firmly to the collagenous fibers of Bruch’s membrane. The firm adhesion and wide angle of detachment make it easy to discern a pigment epithelial detachment ophthalmoscopically. Occasionally a light-orange ring appears around the periphery of a pigment epithelial detachment, further facilitating identification (Fig. 1.56).
Pooling of fluorescein under a sensory retinal detachment in central serous retinopathy takes place slowly, since the dye passes through one or more points of leakage in the defective pigment epithelium (Fig. 1.54). When leakage comes from subretinal neovascularization (Fig. 1.55) or a tumor (Fig. 1.47), it is more rapid and complete. When the pigment epithelium is detached from Bruch’s membrane, fluorescein passes freely and rapidly through Bruch’s membrane from the choriocapillaris into the subpigment epithelial space (Fig. 1.56).
In some cases of central serous chorioretinopathy, there is an associated pigment epithelial detachment, and pooling under each (sensory retinal detachment and the pigment epithelial detachment) is evident. Occasionally, the edge of a pigment epithelial detachment may tear, or rip, and allow fluorescein dye to pass freely into the subretinal space (Fig. 1.57). Drusen may also show late hyperfluorescence similar to that seen with a pigment epithelial detachment (Fig. 1.58). In some cases of pigment epithelial detachment, especially in older patients, subretinal neovascularization is also present. This combination of subretinal neovascularization and pigment epithelial detachment results in an interesting angiogram that can be challenging to interpret (Fig. 1.59).
Staining
Drusen
The most common form of staining occurs with drusen. Most drusen hyperfluoresce early in the angiogram because choroidal fluorescence is transmitted through defects in the pigment epithelium overlying the drusen (Fig. 1.34). Fluorescence from most small drusen diminishes as the dye leaves the choroidal circulation. However, some larger drusen display later hyperfluorescence or staining (Fig. 1.58). The larger the drusen, the more likely they will retain fluorescein and staining will occur. When drusen are large and have smooth edges, the late staining on the angiogram is similar in appearance to that of pooling of fluorescein under a pigment epithelial detachment. In many cases it is difficult, if not impossible, to differentiate large drusen from small pigment epithelial detachments: they have a similar ophthalmoscopic, fluorescein angiographic, and even microscopic appearance.
Scar
Scar tissue retains fluorescein and usually demonstrates well-demarcated hyperfluorescence because little, if any, fluid surrounds the scar. Later in the healing process, when only a few vessels remain, the early angiogram is hypofluorescent because of the paucity of vessels and blockage by the scar tissue. The most commonly seen scar tissue is the disciform scar, which is the endstage of subretinal neovascularization. Scarring is also seen following numerous other insults to the pigment epithelium and choroid, especially inflammation (Fig. 1.60).
Sclera
In several situations the sclera is visible ophthalmoscopically and exhibits late hyperfluorescent staining on fluorescein angiography. Scleral staining is best seen when the retinal pigment epithelium is very pale (as in a blonde patient) or when the choriocapillaris is fully intact. When the choriocapillaris is not intact, fluorescein staining of the sclera can occur from the edges of the atrophic area where fluorescein leaks from the intact choriocapillaris inward toward the atrophy (Fig. 1.60).
Acknowledgments
For online acknowledgments visit http://www.expertconsult.com
Bonus images for this chapter can be found online at http://www.expertconsult.com
Fig. 1.9 The photographer focuses the eyepiece of the camera by initially turning the eyepiece counterclockwise, then clockwise, and stopping when it is in exact focus. The photographer must be sure that the eyepiece crosshairs remain in perfect focus throughout the photographic procedure.
Fig. 1.10 Viewing stereo fundus photographs. (A) Negatives are placed on a viewing back-lit display. Two negative images are then viewed with an adjustable stereo lens. Reading the contact print of the angiogram. The stereo viewer can be easily made up using a trial frame. In viewing negative images, “hyperfluorescence” corresponds to dark objects while corresponding “hypofluorescent” objects are lighter. (B) Reading a stereo pair of digital angiograms. The special viewer allows the observer to focus both images. The software displayed is Ophthalmic Imaging Systems.
Fig. 1.12 An assistant holds the patient’s head as a reminder to the patient to keep the chin in the chinrest and forehead against the bar.
Fig. 1.13 Ten percent fluorescein solution, 5 mL syringe, and 23-gauge scalp-vein needle.
Fig. 1.15 Photographic request form. In the top left portion of the form, the physician indicates the number of color photographs required for each area in the fundus. The physician also indicates the diagnosis because experienced photographers will know which type of photographs to take for each particular diagnosis. The physician also indicates in which location of the fundus the initial-transit phase of the angiogram should take place or, in other words, where the photographer should start the angiogram. In the lower right portion of the photographic request form, the physician can indicate to the photographer specifics about the patient that will facilitate the photographic process and save the photographer time. The physician can indicate whether an eye can fixate on a light, whether the media are clear (so that if the photographer cannot get a clear view, he or she can immediately understand that it is caused by a problem of the eye), and what the patient’s refraction is so that the photographer can know which special lenses to use in the photographic process.
Fig. 1.16 After the needle is placed in the vein, the lights can be turned off so that the photographer can become dark-adapted and see fluorescein flow in the eye. With the use of a hand light, the person injecting can carefully observe the injection site so as to be sure extravasation is not occurring. In this way the fluorescein solution can be injected while the room lights are out.
1 Novotny HR, Alvis DL. A method of photographing fluorescence in circulating blood in the human retina. Circulation. 1961;24:82–86.
2 Rabb MF, Burton TC, Schatz H, et al. Fluorescein angiography of the fundus: a schematic approach to interpretation. Surv Ophthalmol. 1978;22:387–403.
3 Schatz H. Letter: flow sheet for the interpretation of the fluorescein angiograms. Arch Ophthalmol. 1976;94:687.
4 Gass JD, Sever RJ, Sparks D, et al. A combined technique of fluorescein funduscopy and angiography of the eye. Arch Ophthalmol. 1967;78:455–461.
5 Haining WM, Lancaster RC. Advanced techniques for fluorescein angiography. Arch Ophthalmol. 1968;79:10–15.
6 Novotny HR, Alvis D. A method of photographing fluorescence in circulating blood of the human eye. Tech Doc Rep SAMTDR USAF Sch Aerosp Med. 1960;60-82:1–4.
7 Gass JD. Atlas of macular diseases: diagnosis and treatment. St Louis: Mosby; 1970.
8 Yannuzzi LA, Ober MD, Slakter JS, et al. Ophthalmic fundus imaging: today and beyond. Am J Ophthalmol. 2004;137:511–524.
9 Friberg TR, Gupta A, Yu J, et al. Ultrawide angle fluorescein angiographic imaging: a comparison to conventional digital acquisition systems. Ophthalmic Surg Lasers Imaging. 2008;39:304–311.
10 Manivannan A, Plskova J, Farrow A, et al. Ultra-wide-field fluorescein angiography of the ocular fundus. Am J Ophthalmol. 2005;140:525–527.
11 Moosbrugger KA, Sheidow TG. Evaluation of the side-effects and image quality during fluorescein angiography comparing 2 mL and 5 mL sodium fluorescein. Can J Ophthalmol. 2008;43:571–575.
12 Pacurariu RI. Low incidence of side-effects following intravenous fluorescein angiography. Ann Ophthalmol. 1982;14:32–36.
13 Lipson BK, Yannuzzi LA. Complications of intravenous fluorescein injections. Int Ophthalmol Clin. 1989;29:200–205.
14 Lu VH, Ho IV, Lee V, et al. Complications from fluorescein angiography: a prospective study. Clin Exp Ophthalmol. 2009;37:826–827.
15 Zografos L. [International survey on the incidence of severe or fatal complications which may occur during fluorescein angiography.]. J Fr Ophtalmol. 1983;6:495–506.
16 Chen LJ, Yeh SI. Computer-assisted image processing for a simulated stereo effect of ocular fundus and fluorescein angiography photographs. Ophthalmic Surg Lasers Imaging. 2010;41:293–300.
17 Watzke RC, Klein ML, Hiner CJ, et al. A comparison of stereoscopic fluorescein angiography with indocyanine green videoangiography in age-related macular degeneration. Ophthalmology. 2000;107:1601–1606.
18 Patz A, Finkelstein D, Fine SL, et al. The role of fluorescein angiography in national collaborative studies. Ophthalmology. 1986;93:1466–1470.
19 Arnold AC, Hepler RS. Fluorescein angiography in acute nonarteritic anterior ischemic optic neuropathy. Am J Ophthalmol. 1994;117:222–230.
20 Segato T, Piermarocchi S, Midena E. The role of fluorescein angiography in the interpretation of optic nerve head diseases. Metab Pediatr Syst Ophthalmol. 1990;13:111–114.