Factors That Influence the Immune Response
Learning Objectives
• Compare and contrast the determinant selection and hole in the T cell repertoire models
• Recognize how the physical form of the antigen influences the immune response
• Compare the effects of low-dose and high-dose antigens on T and B cells
• Compare and contrast the three different CD4 regulator cells
• Identify the role of FOXp3 in the downregulation of an immune response
• Identify the disease caused by mutations in FOXp3
• Compare and contrast the characteristics of antigen-stimulated CD4 regulator cells
• Recognize the role of Qa-1 in immune regulation by CD8 cells
• Explain activation-induced cell death (AICD)
• Identify the defects or alterations in AICD in individuals with systemic lupus erythematosus (SLE)
• Differentiate between an idiotope and an idiotype
• Explain idiotype network theory
• Recognize the two mechanisms by which anti-idiotype antibodies can downregulate an immune response
Key Terms
Anergy
FOXp3
Idiotope
Idiotype
NTreg cell
Qa-1
Regulator cells
Treg1 cell
TH3 cell
Introduction
The generation of an immune response is determined by genetic factors related to the nature of the antigen, dose, and route of administration. Once a response is mounted, it must be carefully controlled to ensure that it is not directed at normal host tissue. A response must also be easily downregulated when no more antigen is present to drive the immune response.
The immune system has multiple control mechanisms available to damper an immune response after the antigen has been reduced or eliminated. A network of CD4 and CD8 regulatory cells (Tregs) suppresses the function of the lymphocytes involved in autoimmune diseases, allergies, and transplant rejection but without disrupting the function of lymphocytes involved in normal adaptive immune responses. Responses are dampered by two different mechanisms: (1) Some responses are terminated by the killing of CD4 effector cells by a mechanism called activation-induced cell death (AICD). (2) Other responses may be terminated by a perforin and granzymes, anti-inflammatory cytokines, or an anti-idiotypic network.
Genetics and the Immune Response
Human Leukocyte Antigen Glycoproteins and the Immune Response
Some individuals are genetically restricted and are unable to respond to specific antigens. Failure to respond to a specific antigen can be explained by the determinant selection and hole in the T cell repertoire models. The determinant selection model proposes that class II molecules differ in their ability to bind processed antigens. In essence, individuals lack human leukocyte antigen (HLA) molecules that present a specific antigen to T cells. Conversely, the hole in the T cell repertoire model proposes that class II antigen presentation is normal but that some individuals lack a lymphocyte with a T cell receptor (TCR) that recognizes the specific antigen. In both cases, T cell activation fails to occur.
Physical and Biologic Factors Influencing the Immune Response
Form of Antigen and Route of Administration
The physical form of the antigen is important in the generation of an immune response. Because insoluble antigens are easily processed by macrophages, large aggregates favor the generation of an immune response. In contrast, small, soluble antigens cannot be processed by antigen-presenting cells and generate a tolerogenic effect.
The route of administration also determines whether the host mounts an immune response or becomes tolerogenic. Intramuscular and subcutaneous administration of antigens usually elicits an immune response by favoring antigen uptake and presentation by Langerhans cells. In contrast, inhalation, oral, or intravenous administration often fails to generate an immune response.
Maturity of the Antigen-Presenting Cell
Dendritic cells (DCs) are the major antigen-presenting cells in the body. They originate in bone marrow and are released into peripheral blood before migration to lymph nodes. In tissues and lymph nodes, DCs are present as “immature,” partially differentiated cells. These cells can capture and present the antigen in the context of class II molecules but lack the co-stimulatory molecules B7-1 and B7-2, which normally engage CD28 receptors on T cells (see Chapter 6). T cells interacting with immature DCs become anergic. Anergic T cells cannot proliferate or respond to antigen stimulation. In contrast, mature DCs in tissue express class I or class II molecules as well as co-stimulatory molecules. They are efficient in presenting antigens to T cells within the lymph node.
Antigen Concentration
When the antigen is administered over a wide concentration range, only intermediate concentrations induce an immune response. High doses paralyze the immune system by inactivating B cells. Low doses or subimmunogenic doses administered over an extended period inactivate T cells (Figure 22-1).
Passively Administered Immunoglobulin
High doses of passively administered immunoglobulin can suppress the immune response. Pooled immunoglobulin contains antibodies to common bacterial and viral pathogens. Interaction between antibodies in pooled serum and microbial antigens creates immune complexes that block B cell receptor (BCR) activation. Pooled immunoglobulin also suppresses an immune response by engaging the FcγRIIB receptors expressed by monocytes, macrophages, and lymphocytes. FcγRIIB has a cytoplasmic immuno-receptor tyrosine-based inhibitory motif (ITIM), which associates with a tyrosine phosphatase (SHP-1) and downregulates intracellular signaling (Figure 22-2).
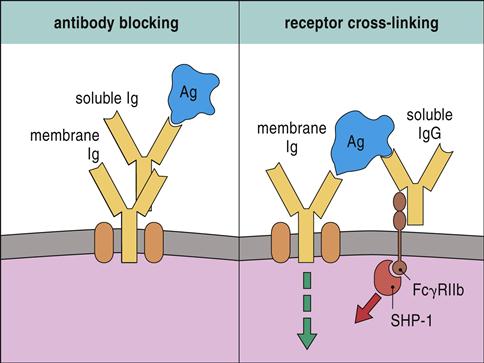