CHAPTER 275 Evaluation, Indications, and Techniques of Revision Spine Surgery
Revision spine operations are increasingly being performed and pose a unique set of challenges to the neurosurgeon. Selection of patients for revision spine surgery is more difficult than selection for primary spine operations, and the likelihood of a good clinical outcome declines with each successive operation.1–4 In addition, reoperations are more technically demanding than primary operations because the normal anatomic structures and tissue planes have been disturbed.5 Scar tissue from previous operations further increases the difficulty of achieving adequate exposure and decompression, as well as the risk for postoperative leakage of cerebrospinal fluid and wound infection or dehiscence.5–7 Complication rates, in general, are higher for revision operations, and thus the decision to perform revision surgery must carefully account for both the altered risk-benefit ratios and the decreased chance of achieving a good clinical outcome with each successive procedure.1,3 Most importantly, the goals of surgery must be clearly defined, and the surgically correctable pathology must be accurately identified.
Initial Evaluation
Radiographic evaluation is made more difficult by previous spine operations. Plain anteroposterior (AP) and lateral radiographs provide the clinician with a great deal of anatomic information, as well as data on preexisting spinal instrumentation constructs. Dynamic radiographs such as flexion-extension films are very helpful for investigating the stability of the spinal column and for determining the integrity of instrumentation constructs and bone fusions. Standing 36-inch AP and lateral radiographs taken with both the hips and knees extended are useful in assessing the sagittal and coronal balance of the spinal column, especially in patients with spinal instability and deformity. Evaluation of bone anatomy and surgical instrumentation is best accomplished with computed tomography (CT) and plain radiography. The information provided by high-resolution, multiplanar CT is helpful when revising previous instrumentation constructs because it can serve as a surgical “road map.” This is particularly important during the revision of pedicle or lateral mass screws when planning new screw entry points and trajectories. High-resolution CT also aids in the assessment of spinal fusion, especially when combined with the information from dynamic radiographs; however, the “gold standard” for assessment of fusion remains open surgical exploration.8,9 Magnetic resonance imaging (MRI) is the most useful study for evaluating the neural elements, ligamentous structures, and intervertebral disks. MRI with gadolinium enhancement is often used for the differentiation of recurrent herniated disk material from epidural scar tissue. Frequently, in patients with spinal instrumentation, imaging artifacts significantly limit detailed MRI evaluation of closely adjacent structures. Myelography, often accompanied by postmyelographic CT, is helpful in determining the presence of thecal sac or nerve root compression in cases in which MRI of the neural elements is limited by artifact.
General Surgical Principles
Attention to the integrity of superficial tissues is essential to decrease the risk for postoperative wound complications such as wound infection and dehiscence. Spine reoperations generally take longer than primary operations, and this is directly correlated with the risk for wound infection.7 Several techniques, such as frequent wound irrigation and hourly release of tissue retractors, help ensure adequate blood flow to tissues and lessen the risk for surgical infections. If the wound edges appear hypovascular or otherwise nonviable, they may be excised to allow primary closure of fresh wound edges.
Recurrent Lumbar Disk Herniation
Recurrent lumbar disk herniation is generally defined as ipsilateral or contralateral herniated disk material at the same level as the index operation that causes symptoms of radiculopathy after a postoperative symptom-free interval of at least 6 months.1,10 Reherniation of a lumbar disk at the same level and site of a previous diskectomy causing recurrent radicular symptoms occurs in 3% to 19%, with higher rates being quoted in studies with longer follow-up.11–16 This phenomenon is more common at the L4-5 level, perhaps because of its increased mobility relative to other spinal motion segments.17 Patients typically experience recurrent symptoms of radiculopathy after a postoperative symptom-free period of at least 6 months. Recurrent radiculopathy at the same level may also occur secondary to degenerative foraminal stenosis, a prolapsed annulus, or excessive epidural scar tissue formation.
Recurrent herniation may pose a diagnostic challenge because imaging studies will demonstrate significant postoperative changes in the epidural space at the operated level, such as epidural scar tissue, adhesions, and bony hypertrophy. Gadolinium-enhanced MRI is optimally used for the differentiation of postoperative changes from recurrent herniated disk material (Fig. 275-1).18–20 Scar tissue generally exhibits a homogeneous pattern of enhancement, whereas disk material will usually demonstrate peripheral enhancement. The herniated disk fragment is typically continuous with the intervertebral parent disk unless sequestration of a free fragment has occurred. Neural elements are often displaced away from a herniated disk fragment, but thecal retraction toward epidural scar tissue can also be observed. Flexion-extension radiographs should be obtained to rule out dynamic instability and to evaluate the need for spinal fusion should revision surgery be necessary. If spinal fusion is being considered, standing 36-inch AP and lateral radiographs are used to assess the sagittal and coronal balance of the spinal column.
Epidural scarring, which occurs after any procedure in the epidural space, may also cause recurrent radiculopathy in some patients.21 The degree of scarring has been correlated with the development of recurrent symptoms. Radiculopathy in this setting is thought to be secondary to nerve root tethering. The generally accepted teaching is that in the absence of a herniated disk, patients with epidural scar tissue and concurrent symptoms do not benefit from reoperation on a consistent basis whereas those with a recurrent herniated disk and concordant radiculopathy do benefit from reoperation.22,23 Patients with degenerative foraminal stenosis or annular prolapse with neural compression may also benefit from revision surgery in selected cases.
Postoperative Spinal Instability and Deformity
Iatrogenic disruption of the posterior tension band, paraspinal muscles, and facet joint complexes may result in the development of spinal instability, with or without subsequent deformity. Additionally, abnormalities of the ventral spinal column may lead to or hasten the development of spinal instability after dorsal surgery (Fig. 275-2). Other factors, including disruption of more than half of the medial facets or young age, also increase the risk for iatrogenic spinal destabilization, especially in the cervical spine.24
Neurological injury is one of the most serious complications that may occur during surgery for correction of deformity. Many surgeons elect to perform intraoperative neurophysiologic monitoring (IOM) in the form of somatosensory evoked potentials, motor evoked potentials, and electromyography to minimize this risk.25–29 Although several studies have demonstrated that IOM may be beneficial in predicting and possibly minimizing the occurrence of postoperative neurological deficits, the importance of proper surgical technique and close attention to the integrity of the neural elements can be neither overemphasized nor replaced. Despite the increasing use of IOM in many centers, its routine use has yet to become universally accepted. This may, in part, be due to concerns regarding the time added to the procedure, cost, availability, and the potential for generating misleading information and unnecessary measures taken to address any false-positive alerts generated by IOM.30–32
Postlaminectomy Cervical Kyphosis
Postoperative deformity of the cervical spine most commonly occurs in the form of kyphosis or anterior subluxation. Accordingly, postlaminectomy cervical kyphosis is a well-established complication of cervical laminectomy.33–38 Cervical kyphosis in this regard refers to a loss of the normal lordotic cervical curvature of at least 5 degrees per motion segment (C2-7); however, overt reversal of the natural lordotic curvature to the point of global cervical kyphosis is not uncommonly encountered in this population. Cervical laminectomy involves resection of the spinous processes, laminae, and interspinous and supraspinous ligaments. The muscular attachments of the paraspinal muscles are disrupted and the erector spinae muscles are weakened as a result of partial denervation.39 The disrupted dorsal elements and weakened posterior tension band favor the development of cervical kyphosis because the natural balance between the extension and flexion moments of the cervical spine is shifted toward the latter.40,41 In the pediatric population, this imbalance often leads to the development of anteriorly wedged vertebral bodies because the growth of immature bone is strongly influenced by the asymmetry of the altered flexion and extension moments.42 Resection of more than 50% of the cervical facet joint complexes is also a strong risk factor for the development of cervical kyphosis and anterolisthesis.24,43,44
The true incidence of postlaminectomy cervical kyphosis has not been fully established, in part because of conflicting definitions of deformity, lack of the long-term follow-up required to detect the development of slowly progressive kyphosis in the adult population, and inclusion of only symptomatic patients in some studies. However, the risk for postlaminectomy cervical deformity in the adult population is reported to be between 5% and 50%.35,43,45–47 An incidence as high as 75% has been reported in patients who have undergone extensive (>50%) bilateral facet resection, thus highlighting the significant stabilizing force of the facet joint complexes.40,43 The exact proportion of patients in whom subsequent clinically symptomatic instability develops is not well established, but the rate may be as low as 5%.33,34
Although the precise incidence of postlaminectomy kyphosis is not known, several risk factors have been identified, including young age (<25 years), preoperative evidence of cervical instability or deformity, a neutral cervical spine, laminectomy performed for degenerative spondylosis, coexisting vertebral fracture or ligamentous injury at the levels to be operated, coexistent structural abnormalities of the ventral elements, the extent of laminectomy (partial resection of the facet joint complexes), and the number of segments (more than four) treated.36,40,46,48,49 Given the relative frequency of this complication, many surgeons elect to perform laminaplasty or prophylactically perform a dorsal instrumented fusion in patients with one of the aforementioned risk factors.37,42,48
Techniques for the correction of cervical postlaminectomy kyphosis include a dorsal approach, ventral approach, or a combined dorsal and ventral approach.38–40,50–53 Preoperative traction may be used in patients with a kyphotic deformity that does not fully reduce with simple neck extension but is not clearly a rigid deformity.38,39,54 The choice of surgical approach is dependent on several factors, including the degree of kyphotic angulation, the structural integrity of the anterior and posterior elements, the presence and location of compression of neural elements, and the presence of either a fixed, incompletely reducible or rigid deformity.39 In general, either a ventrally or dorsally performed revision surgery for cervical postlaminectomy kyphosis requires instrumentation and fusion.
Dorsal Approach
A dorsal surgical approach is often used for patients in whom the kyphotic deformity is either completely or satisfactorily reducible with neck extension and the structural integrity of the ventral bony elements is maintained.39 The neural elements should be free of any bone impingement in the reduced position. The basic techniques of revision spine surgery are applied as outlined earlier, such as extending the margins of the previous operative area in the rostral and caudal directions and working from areas of normal anatomy to those of surgically altered anatomy. Use of visual magnification techniques, such as the operating microscope or loupes, greatly facilitates safe dissection of scar tissue from both the bony and neural elements within the previous surgical field. After exposure of the appropriate spinal levels, additional decompression of the neural elements may be performed as necessary. Once decompression is achieved, reduction of the deformity can be performed. Reduction can be accomplished by having an assistant manually reposition the patient’s head into extension. Lateral fluoroscopy is used to confirm adequate cervical lordosis after manual reduction.
Ventral Approach
The ventral surgical approach is chosen when the kyphotic deformity is either rigid or minimally reducible with neck extension and preoperative traction.38,39 In addition, the ventral approach is used when the ventral bony elements are structurally compromised or are of insufficient height to maintain satisfactory alignment and a long-segment interbody fusion is required (see Fig. 275-2). Ventral decompression may be performed in patients with significant neural compression by ventral bony elements, prolapsed intervertebral disks, and osteophytic ridges. In those with high-degree kyphotic deformity, the spinal cord may be draped over the ventral bony elements (Figs. 275-3 and 275-4). In such cases, ventral decompression is required before any procedure for correction of the kyphotic deformity is performed because the application of corrective distraction forces will increase ventral compression of the spinal cord and may lead to spinal cord injury. The ventral approach affords the additional opportunity to perform ventral release of the longus colli muscles, the anterior longitudinal ligament, and the anterior annulus, thereby facilitating further anterior reduction of the kyphotic deformity.
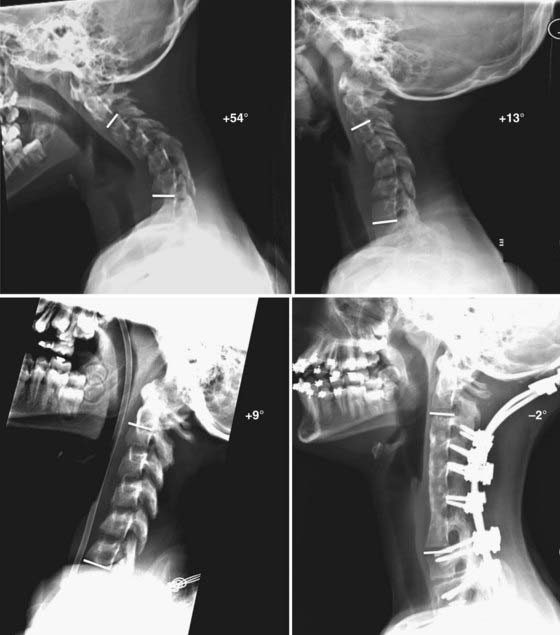
FIGURE 275-4 Flexion (upper left) and extension (upper right) lateral cervical spine radiographs of the patient in Figure 275-3 demonstrating a 48-degree kyphotic deformity measured from C2 to C7 and centered on the C5 vertebral body. The deformity partially reduces to 13 degrees of kyphosis in extension. Lower left, Lateral cervical spine radiograph after 25 lb of Gardner-Wells traction, which provided an additional 4 degrees of correction of the deformity. Lower right, Postoperative lateral cervical spine radiograph 1 year after anterior diskectomies (C3-6), internal reduction, and interbody fusion. A supplemental occipital-T2 posterior fusion with screw-rod stabilization was also performed during the same procedure. An additional 11 degrees of correction of the deformity was achieved and resulted in 2 degrees of lordosis (C2-7).
Gardner-Wells traction is applied and a shoulder roll is placed beneath the scapulae to encourage cervical lordosis. Intraoperative lateral fluoroscopy is valuable in assessing alignment of the cervical spine and centering the incision above the levels to be operated on and during the placement of instrumentation. Either a transverse skin incision or, alternatively, an oblique incision along the anterior border of the sternocleidomastoid muscle (when exposure of three or more spinal segments is required) is performed. The usual method of anterior cervical spinal exposure as described by Cloward is then carried out.55 Caspar distraction posts are placed parallel to the vertebral body end plates, which will lie in a convergent fashion in patients with cervical kyphosis. Distraction is applied along the Caspar posts to place the posts in a parallel orientation. This effectively extends the cervical spine and reduces the kyphotic deformity; however, this maneuver must be used judiciously in patients with suboptimal bone mineral density to avoid vertebral body fracture. Additional distraction and reduction of the deformity are achieved by increasing axial traction via Gardner-Wells tongs by approximately 5 lb per cervical level. Further reduction of the kyphotic deformity is accomplished after performing anterior release of the anterior longitudinal ligament and the outer annulus.
Anterior decompression is performed, as necessary, depending on the degree of kyphotic angulation, the presence of compression of neural elements by ventral structures, and the structural integrity of the ventral bony elements. With regard to correction of deformity, we preferably perform multiple diskectomies rather than corpectomy, when possible, because we are able to obtain improved correction of a kyphotic deformity with the use of multiple lordotic interbody grafts rather than larger strut grafts. Single or multilevel discectomy is performed in the usual manner, depending on the number of segments involved in the kyphotic deformity. Should significant ventral bony compression of the neural elements or evidence of vertebral body collapse exist on preoperative studies, single or multilevel corpectomies are performed at the involved levels. This may be combined with additional discectomies above and below the corpectomy segment or segments as needed, depending on the number of segments with kyphosis. Some authors have advocated preservation of an intermediate point of fixation rather than performing multiple adjacent corpectomies for ventral correction of cervical kyphosis.53 This technique provides three- or four-point bending forces, which enhances the stability of the construct in addition to allowing the surgeon to “deliver” the intermediate point of fixation to a contoured implant for reduction of the deformity. Once complete anterior decompression has been achieved, manual intraoperative neck extension may be used to further increase cervical lordosis.
After decompression, lordotically shaped interbody strut grafts are applied. The interbody grafts should be placed in a ventral position, flush with the anterior margin of the vertebral bodies, to more effectively counteract the flexion moment in the sagittal plane because they will lie in a position as far ventral to the internal axis of rotation (IAR) as possible. This provides the interbody graft with a mechanical advantage to resist the sagittal flexion moment because the force required to resist the flexion moment decreases in a linear manner with increasing distance from the IAR.56 The Caspar distracting pins are then removed and the axial traction is relieved. This maneuver effectively applies an axial load on the interbody strut grafts and thereby enhances fusion and, in effect, offloads the screw-plate system. When performing deformity correction procedures it is preferable to place instrumentation and achieve immediate internal stabilization.50,52,57,58 An appropriately contoured screw-plate construct is then inserted under intraoperative fluoroscopic guidance. If an intermediate point of fixation has been preserved, it may be delivered to the plate system during screw tightening to further enhance lordosis as it is brought in toward the cervical plate. Use of a dynamic anterior screw-plate system, which permits axial subsidence while maintaining lordosis, both lessens the incidence of instrumentation failure and relieves mechanical stress at the screw-bone interface.59–61 The incision is closed in layers and a rigid cervical collar is applied for at least 12 weeks.
If a combined dorsal-ventral procedure has been planned, the patient may be placed in the prone position and dorsal decompression and fusion performed in the same setting or in a staged manner. In general, we prefer to supplement ventral surgery involving corpectomies at two or more levels with a dorsal lateral mass–pedicle screw instrumentation and fusion procedure.40,57,58,62 In cases in which significant posterior spinal canal compromise exists, dorsal decompression can be performed before dorsal fixation, as outlined earlier.
Segmental Instability after Posterior Lumbar Decompression
Dorsal decompression of the lumbar spinal canal is a well-established treatment of degenerative lumbar stenosis.63–67 In some patients who undergo dorsal decompression of the lumbar spine, segmental instability will develop as a consequence of both surgery and, to a lesser degree, structural compromise of the ventral elements. Iatrogenic disruption of the posterior tension band, paraspinal muscles, and facet joint complexes may lead to the development of segmental instability, with or without subsequent spinal deformity.68 Additionally, coexisting abnormalities of the ventral spinal elements, which often arise from degenerative disease, may also contribute to the development of spinal instability after dorsal surgery.
Several risk factors for the development of spinal instability after posterior lumbar decompression have been established, including preoperative radiologic evidence of dynamic instability or anterior spondylolisthesis, preserved disk space height, sagittally oriented facet joints, multilevel decompression, and the extent of facet joint complex or pars resection.44,69–72 It is also important to note that glacial spinal instability may develop in a minority of patients who undergo only partial laminectomy and diskectomy, particularly if more than half to a third of the facet joint complex has been disrupted or if overaggressive resection of the pars interarticularis has been performed.44 This may especially hold true when performing multilevel lumbar laminectomies because the distance between the pars interarticularis progressively decreases at the more cranial vertebral levels. Overaggressive pars resection is more likely to occur at the cranial levels, particularly L3 and above, if the decompression follows a rectangular rather than a trapezoidal shape. Injury to the facet joint from direct capsular cauterization may also contribute to facet joint denervation and glacial destabilization. Nevertheless, the presence one or more of the aforementioned risk factors should encourage the surgeon to consider the inclusion of an instrumented fusion in addition to a dorsal decompressive procedure. Furthermore, inclusion of an instrumented fusion will allow substantially more aggressive decompression of the neural elements because resection of the facet joints and pars interarticularis can be carried out. Such destabilizing maneuvers are frequently required in patients with high-grade canal and foraminal stenosis.
The initial treatment of lumbar spinal instability after lumbar decompression includes a comprehensive trial of nonoperative therapy. If nonoperative therapy fails to control the symptoms or if symptoms of neural compression are present, revision surgery is undertaken. The goals of revision surgery must be carefully and specifically defined. The surgical approach is influenced by the presence of compression of neural elements, the type and severity of the spinal deformity, and the need for grafts or instrumentation to address the deformity. In our experience, patients with lumbar instability and evidence of neural compression will attain maximal benefit from dorsal decompression and instrumented posterior lumbar interbody fusion (Fig. 275-5). The goals of this procedure are to provide decompression of the neural elements, reduction of the deformity, restoration of sagittal alignment, and 360-degree stabilization through one approach. Alternatively, if there is no evidence of neural compression, either an anterior, posterior, or combined stabilization and fusion procedure can be performed. Because many of these patients harbor superimposed progressive multilevel degenerative spinal disease, additional procedures to address the degenerative spine disease at adjacent levels may also be performed as indicated during the same procedure. Accordingly, decompression plus instrumented fusion is often performed at adjacent levels not included in the previous operation.
The surgical technique of posterior lumbar interbody fusion for postlaminectomy lumbar instability consists of exposure of the normal anatomy not included in the previous operation, decompression of the neural elements by further resection of bone and epidural scar tissue, posterior interbody fusion, and instrumented lateral fusion. Under general anesthesia, the patient is placed on a radiolucent Jackson table in the prone position. It is essential that the hips be extended fully to allow the lumbar spine to achieve maximal lordosis. Any hip flexion can be associated with relative kyphotic angulation of the lumbar spine and may predispose to the development of flat back syndrome.73 A radiolucent table is used to aid in intraoperative fluoroscopic imaging in both the coronal and sagittal planes.
After pedicle screw placement, a diskectomy is then performed. Care is taken to preserve the ventral and lateral margins of the annulus to allow the graft material, both structural and morselized, to be contained within the interbody space. Once this is completed, the interbody graft is placed. We prefer the use of two carbon fiber cages packed with morselized graft material, although other types of structural interbody graft can be used as well. The carbon fiber cages that we use have a lordotic sagittal contour with the ventral surface being 2 mm larger than the dorsal surface. In patients with significant loss of lumbar lordosis or a positive sagittal balance, correction of the deformity can be achieved with the use of lordotically shaped interbody grafts.74,75 In patients with spondylolisthesis, a cage of shorter depth is used. For higher grades of spondylolisthesis, the interbody graft is placed after at least partial reduction of the deformity has been accomplished. We perform interbody graft placement via the transforaminal approach. By resecting the medial aspects of the subjacent superior facets, manipulation of the thecal sac and nerve roots is kept to a minimum during placement of the interbody graft.76
Pseudarthrosis
Pseudarthrosis is a commonly encountered complication of both instrumented and noninstrumented spinal fusion surgery.77–86 Pseudarthrosis is defined as the presence of symptomatic bony nonunion more than 1 year after fusion surgery.56 It may lead to motion of the operated spinal motion segment with correlative signs and symptoms of spinal instability. The term nonunion is defined as permanent failure of bone growth across a fracture, regardless of the presence or absence of symptoms and signs of instability. Spinal nonunion can result in a variety of clinical manifestations ranging from an asymptomatic radiographic finding to persistent mechanical or radicular pain or, in its most severe form, to catastrophic construct failure with resultant deformity and neurological injury. Although pseudarthrosis is one of the leading complications of cervical, thoracic, and lumbar arthrodesis surgery, there is a wide range of its reported incidence in the literature.9,78,79,81–83,85–91 This variability is due to diverse surgical and patient factors and the varying methods used to assess bony fusion. The incidence of pseudarthrosis in spinal fusion surgery, in general, has been reported to be approximately 15%91; however, rates approaching 50% have also been reported.85,86,90 This figure varies greatly according to the indications for and the type of surgery, the number of levels fused, the surgical technique, the use of different graft materials or fixation devices, patient demographics, and the presence of systemic factors that could adversely affect bone fusion.
Biology of Bone and Spinal Fusion
Long bone healing occurs in a continuous sequence of acute inflammation, early and late repair, and remodeling.92–94 The acute inflammatory phase occurs 2 to 4 weeks after injury. This phase is initiated by local vascular injury, hemorrhage, and hematoma formation. Local vascular injury leads to an infiltration of acute inflammatory cells and an influx of fibroblasts. Neovascularization and growth of granulation tissue then occur after the acute inflammatory phase. Secretion of a fibrocartilaginous matrix by fibroblasts and formation of soft callus characterize the early repair phase. Callus formation typically occurs approximately 4 to 6 weeks after injury. As the repair process continues, osteoblastic activity progressively replaces the soft fibrocartilaginous callus with woven bone. After a period of 3 to 6 months, the callus is fully replaced by mature cancellous and cortical bone. During the remodeling phase, the fracture callus is remodeled into a new shape that resembles the bone’s original geometry.
Bone graft incorporation plus fusion is a distinct process that entails a phasic response similar to that of fracture healing in which devascularized bone graft is progressively revascularized, resorbed, and incorporated into areas of new bone growth.92,95–97 Decortication and bleeding from the graft recipient site initiate an acute inflammatory phase in which there is local influx of inflammatory cells into the graft recipient site. Revascularization occurs from the host site into the grafted bone. In cortical bone, much of the original bone matrix is replaced during a remodeling phase in which osteoclasts first migrate into the newly vascularized bone graft and resorb the graft matrix. The influx of osteoclasts is followed by activated osteoblasts that produce new bone matrix within the bony voids created by the osteoclasts. The structural integrity of grafted cortical bone is substantially weakened approximately 6 months postoperatively because of osteoclastic resorption until it undergoes remodeling.
In contrast to cortical bone, cancellous bone is first remodeled by an influx of osteoblasts that surround the edges of the grafted cancellous bone trabeculae. Osteoid is deposited by osteoblasts in a lamellar fashion around the dead trabeculae. This process is eventually followed by resorption of the entrapped areas of grafted bone by osteoclastic activity. Thus, in contrast to the early resorption of grafted cortical bone during the remodeling phase, cancellous graft is first strengthened by the formation of new bone before it is resorbed. In this manner, cancellous bone graft attains structural integrity over a period of 3 to 6 months, whereas cortical bone graft attains structural integrity over a period of up to 1 to 2 years. Additionally, revascularization of cancellous bone occurs over a 1- to 2-week period, whereas revascularization of cortical bone takes several months.96,97
A graft is referred to as osteogenic if it can become bone. The process of osteoinduction entails the differentiation of mesenchymal cells into osteogenic cells. Recombinant human bone morphogenetic protein-2 (rhBMP-2) is a well-studied osteoinductive growth factor in spinal fusions.98–100 Osteoconduction involves the growth of capillaries into a graft and the accompanying migration of osteoprogenitor cells along the neovascular channels. These processes are tightly regulated by numerous cytokines, growth factors, and hormonal factors.93,101,102 Autologous graft retains some surviving osteogenic cells, as well as endogenous bone morphogenetic protein (BMP). Autograft is therefore osteogenic, osteoconductive, and osteoinductive.103 Allograft exhibits osteoinductive and osteoconductive properties. Demineralized bone matrix also has osteoinductive and osteoconductive properties.104
Factors Affecting Fusion
Medical Considerations
Bone quality is a well-established factor that affects bone healing and fusion. In addition to age-related osteoporosis, bone quality is also negatively influenced by the presence of systemic diseases such as rheumatoid arthritis, diabetes mellitus, hyperparathyroidism, poor nutritional status, and systemic inflammatory conditions.105–110 Medications used to treat systemic inflammatory diseases, such as nonsteroidal anti-inflammatory drugs (NSAIDs), steroids, and cytotoxic agents, further confound the issue of bone quality in these patients because they are also factors that are known to substantially impede bone healing.102,111–114 Steroids, NSAIDs, and cytotoxic medications interfere with the acute inflammatory phase of bone healing and should be avoided for a minimum of 4 to 6 weeks postoperatively or preferably until fusion has been confirmed.115,116 In patients with the aforementioned risk factors, bone densitometric studies, such as dual-energy x-ray absorptiometry (DEXA), are helpful in quantifying bone quality in an objective manner. DEXA studies are usually performed on the femoral neck, distal radius, and lumbar spine. In patients who have previously undergone lumbar fusion, the femoral neck and radius should be used instead to assess global bone mineral density.117 Quantitative CT is a highly specific and sensitive modality for the detection of osteoporosis that can be used to analyze bone density in both the cortical and trabecular compartments at any skeletal site.118–120 Such use of this modality is currently limited by increased cost and radiation exposure.121 Quantitative evidence of poor bone mineral density provides a scientific basis for the failure of a previous spinal fusion and should compel the clinician to use advanced techniques for enhancement of the chance of successful fusion in subsequent operations. This may be accomplished by improving the construct design, using autograft or biologic modifiers of bone healing, and load sharing with extended constructs or the addition of circumferential stabilization. Modifiable risk factors such as malnutrition and hyperparathyroidism require correction before any elective revision fusion surgery is performed. In patients with rheumatoid disease or other systemic inflammatory conditions, cytotoxic medications, steroids, and NSAIDs should be tapered and discontinued (if possible) preoperatively and avoided for as long as possible during the postoperative period to promote bone healing and fusion.
Osteoporosis is a widely prevalent metabolic disease that mainly affects postmenopausal women. Iatrogenic osteoporosis is also known to occur in patients who have undergone bilateral oophorectomy or long-term corticosteroid therapy. Osteoporosis is characterized by a general loss of bone mineral density and total bone mass. DEXA studies are essential in quantifying the extent of disease. In patients with significantly reduced bone mass, fusion surgery is generally avoided when possible. In the setting of osteoporosis and failed spinal fusion, a multifaceted strategy is required to optimize bone mineral density, as well as the biomechanics of the construct design. Medical therapy, which includes calcium and vitamin D supplementation, should be used to optimize bone mass in these patients.122 Patients who have osteoporosis secondary to oophorectomy are often able to achieve increased bone mineral density after estrogen replacement therapy.123 Bisphosphonate therapy, which has emerged as a mainstay in the treatment of postmenopausal osteoporosis, has been shown to paradoxically decrease spinal fusion rates in several animal models.124–127 Thus, this agent should be avoided in patients undergoing fusion surgery. More recently, recombinant parathyroid hormone (rPTH) has gained increasing acceptance for the treatment of postmenopausal osteoporosis.128 rPTH must be administered systemically on a daily basis and contains amino acids 1 to 34 of the complete PTH molecule, which is the osteogenic component of PTH. rPTH exerts an anabolic affect on bone healing by increasing the rate of new bone formation, as well as by increasing bone mineral density.129,130 rPTH has been shown to increase the rate of bone formation, accelerate spinal fusion, and increase the mineral density of spinal fusion masses in animal models.131,132
Cigarette smoking is a common modifiable risk factor for pseudarthrosis that can drastically affect the rate of spinal fusion. The risk for nonunion in patients who smoke is approximately three times that of nonsmoking patients, with fusion rates being decreased by up to 40% in smokers.133–138 Thus, smoking is generally regarded as the single most deleterious modifiable risk factor for nonunion. Smoking is known to restrict the growth of small vessels into fusion sites, inhibit revascularization of the bone graft, and inhibit osteoblastic function, and it may lead to a generalized loss of bone density.139,140 The nicotine component of tobacco is a well-recognized inhibitor of revascularization of bone grafts and a direct inhibitor of osteoblastic function.139,141 The presence of systemic nicotine itself is an established risk factor for spinal nonunion.141,142 Given the high rate of pseudarthrosis in smokers, many physicians simply refuse to perform elective spinal fusion in patients who continue to smoke. An intermediary approach that involves referral of patients with moderate symptoms and no evidence of structural instability to smoking cessation programs has also been advocated.143 The decision to perform fusion surgery in these patients then depends on a combination of the success of a smoking abstinence program, the presence of additional risk factors for nonunion, and the patient’s symptoms. In patients with symptomatic pseudarthrosis who smoke, however, implementation of a preoperative smoking cessation program may not be feasible. In this setting, the focus should be placed on surgical strategies to improve bone healing, as outlined earlier, in addition to an aggressive postoperative smoking cessation program.135 Although nicotine replacement therapy is useful in preoperative smoking cessation programs, given the deleterious effects of nicotine on spinal fusion, postoperative nicotine replacement therapy should be avoided in patients undergoing fusion surgery.
General Surgical Considerations
In patients with established pseudarthrosis or who harbor additional risk factors for nonunion, it is critical to use all available surgical techniques that will optimize bone healing and maximize the chance of obtaining a successful fusion. Surgical techniques that affect bone healing and improve the chance of achieving successful fusion include proper preparation of the host site, graft placement, and type of graft implanted; the use of biologic agents that enhance bone growth, such as rhBMP-2; and electrical bone stimulation. Meticulous attention should be directed toward placement of a biomechanically sound internal fixation construct to attain immediate and durable stabilization of the operated motion segments. Surgical strategies are often directed toward load sharing by extending the length of the construct over many segments and optimizing the biomechanics of the bone-implant interfaces. Additionally, circumferential stabilization may also be achieved by performing ventral stabilization in addition to dorsal stabilization.4,143–146
Graft Selection
Selection of the graft material depends on the biomechanical environment of the construct, the presence of biologic risk factors for nonunion, and the type of surgery. The gold standard graft material for spinal fusions remains autologous iliac crest.103,147–156 Other options for graft material include locally harvested bone, autologous rib or fibula, morselized nonstructural autograft packed in titanium, polyetheretherketone (PEEK) or carbon fiber cages, allograft (structural or morselized), or bone modification agents such as BMP.103 Each type of graft material has its own advantages and disadvantages.157 In general, use of the patient’s own bone is preferable to the use of allograft or bone modifiers for several reasons. Autograft is genetically identical to the patient, carries no risk for donor-related infection or rejection, and has inherent osteogenic, osteoinductive, and osteoconductive properties.92,103,158 Disadvantages of autograft include harvest site complications such as bleeding, pain, nerve injury, and hernia formation, as well as the chance of harvesting structurally inadequate bone in osteoporotic patients.159–161
Allograft bone is advantageous in that it is readily available in the United States, is structurally sound, and carries no risk for donor site complications. There is a low risk for donor-related infection and immune-mediated rejection, although this risk varies according to the particular methods used for pretreatment of the donor bone.158 Allograft does retain osteoinductive and osteoconductive properties but has no osteogenic properties. Its osteogenic properties may be enhanced, however, by supplementation of the allograft with BMP or autograft. Multiple studies comparing the fusion rates obtained with autograft and allograft continue to substantiate the superiority of autograft in achieving solid bone fusion.148 However, as newer technologies have emerged, the fusion rates seen with allograft continue to improve. Although equivalent fusion rates with autograft and allograft have been observed for certain types of spinal fusion (e.g., single-level anterior cervical fusion),149,162 every effort to ensure successful fusion should be made in patients with symptomatic pseudarthrosis. This should be considered on a case-by-case basis. If pseudarthrosis has occurred after the use of allograft, autograft should be used for the revision surgery. If it is not possible to use autograft, other methods of enhancing the chance of successful fusion should be considered, such as the use of allograft supplemented with autograft or circumferential stabilization techniques.144,163 If pseudarthrosis has occurred after the use of autograft, the fusion should be revised and optimized biomechanically by the addition of additional stabilization or a circumferential fusion procedure with an interbody graft.
Bone Morphogenetic Protein
BMPs are a group of structurally similar proteins within the transforming growth factor-β family.164 These molecules have receptors on multiple cell types and play a role in the proliferation of mesenchymal cells and the differentiation of chondrogenic and osteogenic precursor cells.98,165 rhBMP-2 is a well-studied osteoinductive agent that has been shown to increase the rate of new bone formation and improve fusion rates in the lumbar and cervical spine in multiple human studies.98–100,166,167 As of 2008, rhBMP-2 is approved by the U.S. Food and Drug Administration for single-level anterior interbody fusion at the L4-5 and L5-S1 levels when administered in a collagen sponge and tapered interbody cage. Other uses of rhBMP-2 as an adjunct to spinal fusion remain off label.167 Because of its strong osteoinductive capacity and improved fusion rates, rhBMP-2 is frequently used in an off-label fashion to enhance spinal fusion, especially in patients who harbor risk factors for nonunion. Concerns regarding soft tissue swelling, particularly with the use of rhBMP-2 in anterior cervical surgery, as well as vertebral osteolysis, end plate erosion, and ectopic bone formation, have been raised.153,167–170 Specific guidelines regarding the off-label use of rhBMP-2 are lacking because of the limited amount of data on this subject and the diversity of clinical studies that currently exist. A careful risk-benefit analysis should be done when using rhBMP-2 in an off-label fashion, particularly when considering its use for anterior cervical surgery. We reserve the off-label use of rhBMP-2 for the most challenging cases of pseudarthrosis involving multilevel posterior lumbar revision surgery in patients with multiple coexistent risk factors for recurrent nonunion.
Electromagnetic Bone Stimulation
Electromagnetic bone stimulation (ES) was first described as a method of promoting fusion in long-bone fractures.171 The bioelectric potentials of bone have since been well described.172–178 Areas of resting bone or bone placed under tension carry a net electropositive charge, whereas actively remodeling bone and bone placed under compressive forces carry a net electronegative charge. ES enhances bone growth and fusion by creating an electronegative environment in the region of an intended fusion.175 Induction of an electronegative environment has been shown to improve bone remodeling and healing by the inhibition of osteoclastic activity and promotion of osteogenesis through several cellular and subcellular mechanisms. Three forms of ES are currently in clinical use.173 Direct-current ES requires surgical implantation of stimulating electrodes and a current generator. Pulsing electromagnetic fields and capacitatively coupled electrical energy are two types of externally applied ES that may be less effective than direct-current ES in that the latter creates a more focused region of electromagnetic current within the intended fusion bed.173 Several studies have supported the use of ES for improving fusion in the lumbar spine.179–181 It has also consistently been observed that the benefits of ES appear to be more significant in multilevel fusions or in patients deemed at high risk for nonunion, such as those who smoke or have a history of pseudarthrosis.182–184 More recently, the use of ES has been extended to include cervical spine fusions,185,186 although the data are less robust than for lumbar spine fusions. We prefer to reserve the use of direct-current ES for patients undergoing revision surgery for lumbar pseudarthrosis who harbor multiple additional risk factors for nonunion such as smoking or systemic inflammatory disease. Implanted ES devices may be removed after radiographic demonstration of successful fusion.
Dorsal Surgical Techniques
Preparation of the Graft Recipient Bed and Graft Placement
Nonstructural bone grafts carry a higher risk for nonunion than do grafts placed under compression. The posterior elements are under tensile force, whereas the anterior column is under compressive force. In accordance with Wolff’s law, compression provides a more favorable environment for bone growth and fusion to occur.187 Posterolateral bone grafts placed under tension carry a higher risk for nonunion than do anterior column grafts placed under compressive force.143 In addition, the recipient graft bed in posterolateral fusions is a much less ideal environment for bone growth because the host-graft interface is less robust and less vascular than in anterior column grafts. Given these inherent barriers to posterolateral fusion, autograft or, less ideally, allograft supplemented with autograft should be used for posterolateral fusions in patients with established pseudarthrosis.
Thorough preparation of the graft recipient site is essential to allow proper bone growth, graft incorporation, and successful fusion to occur. The recipient bed serves as the primary source of both the cellular components and the neovascularization necessary for full incorporation of the bone graft. Complete removal of soft tissue from the recipient bed ensures direct apposition of donor and recipient bone graft material and prevents excessive growth of fibrous tissue into the area of the planned fusion.96 Decortication of the recipient bed until osseous bleeding is encountered ensures adequate contact between recipient cancellous bone and the bone graft, which further enhances bone growth. Care must be taken to avoid excessive removal of cortical bone, which may lead to unnecessary structural compromise of the recipient site. The joint surfaces are prepared for fusion by removal of articular cartilage and other soft tissue structures. The use of bone wax to achieve osseous hemostasis in areas of planned fusion poses a mechanical barrier to neovascularization of and cell migration into the graft and should be avoided.
Instrumentation and Biomechanical Environment
Placement of a biomechanically sound posterior fixation construct requires a robust bone-implant interface and a proper biomechanical environment for stabilization. Screw-plate or screw-rod constructs provide a bone-implant interface superior to that of hook or wire stabilization constructs, and their use is preferred in cases requiring revision stabilization and fusion.77,81,82,105,144,188–192 To obtain the best possible screw-implant interface, the largest possible screw that is permitted by the local anatomy should be used to maximize bone purchase and improve the strength of the construct.193 Screw pullout strength is directly related to the major (outer) screw diameter, whereas bending strength and fracture resistance are directly related to the minor (inner) diameter.
Sagittal plane deformity may be either a cause or a result of pseudarthrosis. Correction of positive sagittal imbalance is imperative when treating patients with pseudarthrosis.143,144,194,195 If positive sagittal balance exists, substantially increased flexion moment and strain are placed on the posterior instrumentation. The additional stresses placed on the posterior instrumentation by the increased flexion moment may lead to screw loosening, pullout, or fracture and, subsequently, failure of the construct (Fig. 275-6). Additionally, the increased flexion moment lessens the compressive forces that enhance bone growth and spinal fusion. Positive sagittal imbalance may be corrected by applying compressive force to the posterior instrumentation to effectively shorten the posterior column and improve lordosis. This technique is effective only when combined with ventral interbody structural support to prevent excessive flexion on axial loading. In patients with low bone mineral density, however, we prefer to use instrumentation only to stabilize the bony elements rather than exert sustained corrective forces. Pedicle subtraction osteotomy can be performed in more severe cases of excessive positive sagittal plane deformity (Fig. 275-7).196,197 Alternatively, placement of a lordotically shaped ventral interbody graft, which effectively lengthens the anterior column, will serve to improve a positive sagittal imbalance.74,75,143,144,194,198
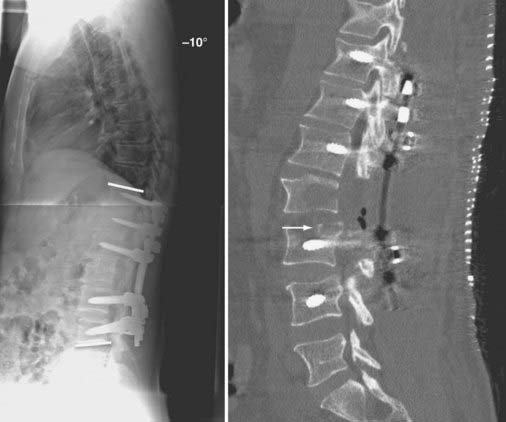
FIGURE 275-7 Postoperative lateral radiograph (left) and a sagittal reconstructed computed tomography scan (right) of the patient in Figure 275-6. Posterior decompression of L2-3 was performed, followed by pedicle subtraction osteotomy at L2. Posterior fusion and stabilization of T11-L4 were also performed. Bone purchase and construct strength were improved by placement of larger diameter pedicle screws and a caudal extension of the construct to L4. New screw entry points and trajectories were used at L3 bilaterally to improve bone purchase. The previous L3 screw trajectory is visible (arrow) superior to the new L3 pedicle screw trajectory. A 26-degree correction of the deformity was accomplished. The patient reported significant improvement in his lower back pain and bowel function.
Ventral Surgical Techniques
Preparation of the Graft Recipient Bed and Graft Placement
Anterior column graft recipient sites are prepared in a similar manner while ensuring that all articular cartilage is removed from the vertebral end plates. Decortication of the end plates is performed until osseous bleeding is encountered. Care is taken to avoid excessive structural compromise of the end plate, which may lead to subsidence under axial loading.199 The graft–recipient site interface should be flat to maximize the area of direct contact between the graft and host site.
Placement of anterior column grafts in conditions of axial compression is also an important technique that leads to load sharing with posterior instrumentation constructs.198,200,201 A properly loaded anterior column interbody graft may absorb up to 80% of the axial load,143 thereby decreasing the biomechanical stress (both axial loading and flexion moments) placed on posterior constructs. This maneuver decreases the incidence of screw pullout and loosening and failure of the posterior instrumentation construct. Additionally, anterior column grafts should be placed under compressive axial loading force because such force will enhance bone growth and fusion in accordance with Wolff’s law.187 Axial loading of anterior column grafts is typically accomplished by placing the graft while a distractive force is applied to the vertebral bodies. In anterior cervical surgery, this is accomplished with the use of distraction pins or skeletal traction via skull-mounted tongs. For the placement of interbody devices through a posterior approach, interbody grafts are preloaded by first distracting across previously placed pedicle screw instrumentation. It is important to note that distraction of the posterior elements is a kyphogenic maneuver because most distraction occurs posterior to the IAR. Distraction across long pedicle screws that span ventral to the IAR can minimize this effect. As noted earlier, placement of a lordotically shaped ventral interbody graft, which effectively lengthens the anterior column, will serve to improve a positive sagittal imbalance.75,194 After the graft is placed in its final position, the distractive force is then relieved and the vertebral body is allowed to recoil under its native compressive force. Caution should be exercised when using this maneuver in patients with low bone mineral density because an excessive distraction force may lead to screw pullout or fracture.
Instrumentation and Biomechanical Environment
As discussed earlier, placement of a ventral interbody graft under axial compressive force enhances fusion according to Wolff’s law. In this manner, the interbody graft is preloaded before placement of the ventral plating system. Ventral plating systems should share load with the interbody construct. By sharing load with the ventral plating system, the graft is shielded from excessively high stress under axial loading, which can lead to axial subsidence or collapse of the graft. Dynamic ventral plating systems, which allow controlled axial subsidence while maintaining sagittal plane alignment, are used to minimize the risk for screw fracture and implant failure.202,203 New screw entry points and trajectories should be used when possible to maximize the strength of the bone-implant interface (Fig. 275-8; also see Fig. 275-7). Merely cannulating the previous screw holes is avoided whenever possible.
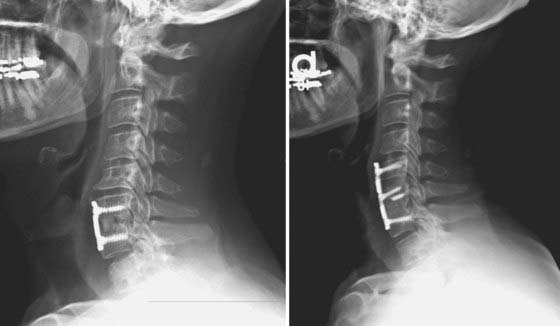
FIGURE 275-8 Left, Lateral cervical spine radiograph of the patient in Figure 275-12 demonstrating linear radiolucencies above and below the interbody allograft consistent with nonunion. Given the radiologic evidence of nonunion and concurrent symptoms of axial neck pain, a diagnosis of pseudarthrosis was made. Right, Postoperative lateral cervical spine radiograph after revision of the C6-7 interbody fusion, C5-6 anterior diskectomy, fusion, and C5-7 screw-plate stabilization. Intraoperatively, the previously placed C6 screws were found to be fractured bilaterally. Autologous iliac crest was used at both levels in view of the patient’s history of pseudarthrosis. New screw entry points and trajectories were used to improve screw purchase in the C6 and C7 vertebral bodies.
Clinical Evaluation
The diagnosis of pseudarthrosis requires a thorough radiographic evaluation and correlation of the radiographic findings with symptoms and signs that are directly referable to spinal instability or neural compression. The general scheme for the clinical evaluation of pseudarthrosis first delineates between patients with and without mechanical or radicular pain. In patients with pain, a distinction is made between those with evidence of neural compression and those with evidence of spinal instability, although these two entities often coexist in many cases. In general, the presence of persistent or recurrent mechanical pain for longer than 1 year after spinal fusion surgery with coexistent radiographic evidence of nonunion establishes a diagnosis of pseudarthrosis. In many cases, however, accurate diagnosis of pseudarthrosis remains difficult. The gold standard for the diagnosis of pseudarthrosis remains open surgical exploration,86,87,204 although routine use of this modality is neither cost-effective nor practical.
Radiographic evaluation includes obtaining AP, lateral, and oblique radiographs. Fine-cut CT with multiplanar reconstruction has proved very helpful for the evaluation of bone fusion, with several studies demonstrating increased sensitivity and specificity with this modality over the use of plain films alone.8,9,78,86,89 Flexion-extension dynamic radiographs are critical for evaluating spinal stability, although low-grade dynamic instability may not be detectable on plain radiographs in some cases.78,88 Excessive motion at the fused levels may be indicative of nonunion, but there is disagreement about which parameters constitute excessive motion.90 Most reports consider between 5 and 10 degrees of angular motion on flexion-extension studies as being abnormal. In the cervical spine, measurement of the interspinous distance may demonstrate nonunion if more than 2 mm of motion is present on flexion-extension radiographs.86 MRI is not routinely used for the assessment of fusion because it is often limited by artifact from adjacent instrumentation and poor resolution of bony anatomy.
When evaluating plain films or CT scans for nonunion, one should first look for radiographic evidence of solid fusion. After anterior interbody fusion, bridging trabecular bone between an interbody graft and the vertebral end plates indicates successful fusion. After posterior cervical fusion, bridging trabecular bone within the lateral gutters or between the posterior elements is suggestive of fusion (Fig. 275-9).89 In the thoracic and lumbar spine, a solid and continuous trabecular pattern of bone across the grafted segments is indicative of fusion. In posterior lumbar fusions, bridging trabecular bone incorporated into the transverse processes or posterior lateral elements generally indicates that successful fusion has occurred.
Overt radiographic evidence of nonunion includes fracture or movement of the instrumentation, collapse of the construct, a progressive spinal deformity, resorption or collapse of the bone graft, a gap between the vertebral end plate and an interbody graft, or a radiolucency within the fusion mass (Fig. 275-10). Screw instrumentation may demonstrate screw pullout, screw fracture (see Figs. 275-8 and 275-9), or a circular radiolucency or “halo” at the bone-screw interface, which is also suggestive of nonunion (Fig. 275-11; also see Fig. 275-6). The presence of a halo at the bone-screw interface is indicative of persistent motion. This may be an acceptable finding in some cervical nonconstrained or semiconstrained stabilization systems; however, the halo should resolve over time in this clinical scenario. Fine-cut CT with three-dimensional reconstructions may demonstrate defects or gaps within the fusion mass or a radiolucency between an interbody graft and vertebral end plate that are not detectable on plain radiographic studies.
Because the clinical diagnosis of pseudarthrosis is difficult, the treatment decision paradigm is also difficult. The decision to perform revision surgery is based on the severity of the clinical signs and symptoms, as well as the degree of radiographic evidence of nonunion. The presence of isolated radiographic evidence of nonunion without any symptoms or signs does not warrant surgical treatment. A patient with clear radiographic evidence of instability or gross deformity and progressive neurological deficits referable to the spinal instability warrants a timely revision operation. However, most patients with pseudarthrosis have a clinical picture that is intermediate between these two extremes, and the indications to proceed to a revision fusion are not absolute. The presence of a painful nonunion without progressive deformity or compression of neural elements may be treated conservatively by observation, an external orthosis, facet or epidural steroid injections, or an external bone stimulator.182 Radiographic follow-up is indicated to track the progress of the fusion and identify any evidence of delayed bony union. A unique clinical situation can arise in which there is late instrumentation failure followed by bony fusion. This relatively rare event may occur in the cervical spine with constrained stabilization systems because of settling of the construct. Revision surgery is considered after failure of the aforementioned modalities, in patients with intolerable and intractable pain, or in those who have a progressive spinal deformity or a new neurological deficit. The patient’s willingness to undergo a revision operation is a critical element of the decision process.
Surgical Strategy
Cervical Procedures
Ventral Surgical Techniques
Cervical pseudarthrosis after anterior fusion may be addressed by directly revising the anterior fusion construct or by performing a posterior fusion.205–208 Considerations in choosing the approach include the presence of spinal deformity or graft extrusion, residual anterior neural compression, symptomatic adjacent segment disease, and cervical stenosis. Anterior surgery is indicated if there is evidence of graft extrusion,209 anterior neural compression, or symptomatic adjacent segment herniated disk disease (Fig. 275-12; also see Fig. 275-8). Anterior surgery has the advantage of being able to remove fractured instrumentation or an extruded interbody graft, provides superior decompression of the nerve roots, and avoids the potential pain and muscular atrophy associated with a posterior cervical approach. Anterior revisions have several inherent drawbacks, however. Scarring of the anterior neck structures makes the surgical approach inherently more difficult, and there is a high incidence of dysphagia when revision surgery is performed via an ipsilateral approach.210 A contralateral approach may be used to avoid dissection through scar tissue; however, recurrent laryngeal nerve injury related to the index operation must be ruled out by laryngoscopy before this approach is considered.211 If there is any evidence of recurrent laryngeal nerve palsy, the contralateral approach cannot be performed because bilateral laryngeal nerve injury may lead to life-threatening upper airway obstruction. In addition, several studies have demonstrated an increased rate of nonunion after anterior cervical revisions.205,206,208 Posterior fusion may be performed with the same technique as used for primary posterior cervical fusions. In addition, with posterior surgery, decompressive laminectomy to address cervical stenosis may be performed, and the fusion may be extended above or below the levels of a previous anterior fusion in patients with symptomatic degenerative instability.
Dorsal Procedures
Subaxial Cervical Spine
Pseudarthrosis after ventral fusion of the subaxial cervical spine may be addressed by the addition of a dorsal fusion and fixation procedure, as discussed earlier (see Figs. 275-9 and 275-10). Accordingly, pseudarthrosis after dorsal subaxial cervical fusions may be addressed by the addition of ventral fusion and fixation if additional indications for ventral surgery exist, such as the need for ventral decompression of the operated levels or for ventral decompression and fusion of motion segments adjacent to the previous fusion.
Revision of a dorsal fusion of the subaxial spine is performed by obtaining complete exposure of the fusion construct out to the lateral borders of the facet joint complexes. Care is taken to preserve the posterior tension band and facet joint complexes cranial and caudal to the fusion construct. Thorough exploration for gaps within the fusion mass and inspection of the instrumentation for motion or fracture are carried out after exposure. It is often necessary to remove all hardware when performing a dorsal revision fusion procedure. The posterior elements are thoroughly cleaned of scar tissue, and fibrous tissue is removed from the previous bone-implant interfaces by curettage. If the screw holes are to be reused, the inner edges of the screw holes are scraped clean with small angled curets. The remaining fusion mass and posterior elements are then decorticated with a high-speed drill until bleeding bone surfaces are exposed. It is important to achieve complete exposure and disruption of all articular surfaces that are to be fused. Instrumentation is then placed accordingly. In cases in which lateral mass and pedicle or pars screws are to be placed, screws with the largest diameters and lengths are implanted under the guidance of preoperative CT scans and the clinical impression at the time of surgery. Larger diameter “rescue screws” may be placed in the previous screw holes to improve bone purchase. If possible, new screw entry points and trajectories can alternatively be used.192 In patients with poor bone quality or those who require multisegment stabilization, we prefer to use constructs that extend to C2 and C7 to allow the placement of pedicle or pars screws at these levels. These screws are longer and have higher pullout strength than cervical lateral mass screws do.192,212–214 Preoperative evaluation of the C2 pars by CT with multiplanar reconstructions is often needed to determine the relative position of the transverse foramen and the feasibility of a placing a C2 pars screw. We are typically able to place screws 20 mm in length at the C2 pars and screws 24 to 26 mm in length at the C7 pedicles. This technique provides increased strength at the cranial and caudal ends of the construct and improves the overall stability of the construct. After insertion of the instrumentation, bone graft is placed throughout the lateral gutters in direct contact with the decorticated bone surfaces. An autograft, either local or remote, is preferably used in patients with established pseudarthrosis. A local autograft is supplemented with an allograft or additional remote autograft when insufficient bone is able to be harvested locally. Depending on the presence of additional risk factors for nonunion and the patient’s bone quality, an allograft may be used either as an alternative or in addition to an autograft. After closure, the patient is routinely placed in a hard cervical collar for at least 12 weeks postoperatively.
Lumbar Procedures
The surgical strategies available to address lumbar pseudarthrosis are diverse and depend primarily on the type of fusion that was performed previously. The overall goal of lumbar pseudarthrosis revision surgery is to optimize both mechanical fixation and the environment for bone healing. Strict adherence to the basic techniques that promote bone healing, such as proper graft placement and recipient bed preparation, is critical for obtaining successful fusion. In the lumbar spine, biomechanical optimization can be accomplished by restoring the sagittal balance of the spine and by load sharing through the addition of internal fixation devices. The presence of a significantly positive sagittal balance places a substantially increased flexion moment on posterior instrumentation.143,194,195 This may lead to implant loosening, failure, and pseudarthrosis. Methods used to correct positive sagittal imbalance include the addition of ventral interbody devices, dorsal compression and fixation, and pedicle subtraction osteotomy.
The presence of neural element compression may dictate the choice of approach for a revision fusion (Figs. 275-13 and 275-14). We prefer to perform posterior interbody fusion in cases involving compression of the neural elements because more complete decompression can be performed via the dorsal approach. In the absence of neural element compression, the choice of approach is largely influenced by the nature of the previous operation.
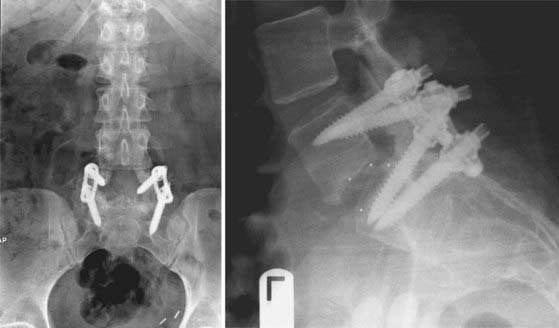
FIGURE 275-14 Postoperative anteroposterior (left) and lateral (right) radiographs of the same patient in Figure 275-13. Revision posterior lumbar interbody fusion was performed. All previous instrumentation, including the migrated interbody cage, was removed. Intraoperative exploration demonstrated L5-S1 nonunion and segmental instability. New, bilateral interbody cages were placed through the same posterior exposure. Bilateral posterolateral fusion with autologous iliac crest graft and bilateral pedicle screw-rod stabilization were performed. The patient experienced complete relief of her radicular symptoms and lower back pain after the revision operation.
The addition of internal fixation devices is a commonly used technique in revision lumbar surgery. If a noninstrumented posterolateral fusion has failed, the fusion can be revised by the addition of pedicle screw instrumentation. Supplementation with circumferential stabilization improves the chance of achieving successful fusion and better clinical outcomes.4,144–146,163 In contrast to the cervical and thoracic spine, 360-degree stabilization and fusion of the lumbar spine can be accomplished through a single posterior approach inasmuch as an interbody strut graft can be placed through either a direct posterior or transforaminal approach. This opportunity often negates the need to perform combined anterior and posterior surgery. Thus, the majority of cases of pseudarthrosis of the lumbar spine can be managed successfully through a single dorsal exposure.
In cases in which an instrumented posterolateral fusion has failed, an interbody device may be implanted via the posterior approach to provide ventral column support and load sharing with the posterior construct. In addition, revision of the posterolateral fusion and replacement of the instrumentation can be carried out through the same posterior exposure (Figs. 275-15 and 275-16).4 Alternatively, an anterior approach may be used to avoid reoperating through a previous dorsal exposure.
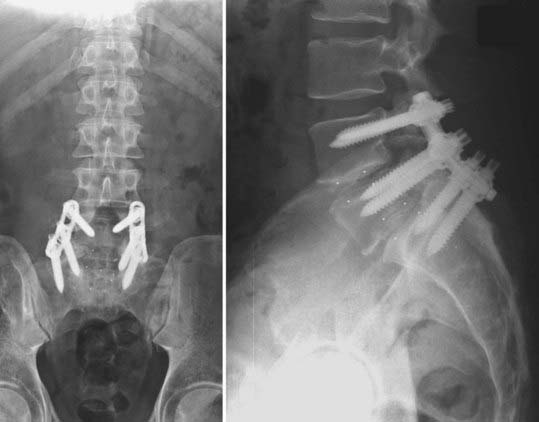
FIGURE 275-16 Postoperative anteroposterior (left) and lateral (right) radiographs of the patient in Figure 275-15. Exploration of the L5-S1 fusion mass demonstrated nonunion and segmental instability. The L5-S1 fusion was revised by placement of bilateral interbody cages through a posterior approach. Open reduction of the L4-5 spondylolisthesis was accomplished, followed by bilateral interbody L4-5 cages and an instrumented L4-S1 posterior fusion with autologous iliac crest graft.
In cases of failure of a standalone ventral interbody fusion, the fusion may be revised through the anterior approach.4,215 Alternatively, the revision can be performed by the addition of an instrumented posterolateral fusion, with or without revision of the ventral interbody fusion, both accomplished through a single dorsal approach.146 In cases of failure of a dorsal-ventral fusion, the entire construct should be revised with an emphasis on improving the biologic environment for bone healing, often with the inclusion of an internal bone stimulator or other adjunctive measures. In the great majority of cases of revision lumbar fusion surgery, the use of autologous graft is preferred given the established history of a failed spinal fusion and the increased risk for recurrent nonunion.
Thoracic Procedures
Nonunion of the thoracic spine occurs infrequently because of its inherent rigidity and immobility relative to the cervical and lumbar spine.216 Thoracic spinal fusions are also performed less commonly than fusions in the cervical or lumbar region. The greater rigidity of the thoracic spine is largely afforded by its articulations with the ribs, the anterior support offered by the sternum, and the paraspinal musculature.217 The cervicothoracic and thoracolumbar junctions endure substantial stress as a result of the lever arms exerted on the thoracic spine at these anatomically transitional locations.218–222 Stabilization systems placed in the transitional segments at the cranial or caudal extremes of the thoracic spine must accordingly bear increased biomechanical stress and are consequently more susceptible to construct failure, instability, and pseudarthrosis.
The majority of cases of thoracic pseudarthrosis are attributable to implant failure, sagittal imbalance, and improper hardware placement. In particular, positive sagittal plane deformities place an exaggerated flexion moment on posterior stabilization constructs that may lead to implant loosening and instability. Revision strategies must be individualized and are focused on correction of the identifiable mechanisms responsible for the failure of fusion. Placement of a biomechanically robust internal fixation construct capable of withstanding the substantial bending moments inherent in the cranial and caudal ends of the thoracic spine is critical for achieving successful fusion. Thoracic pedicle screw instrumentation provides more robust multiplanar stabilization than does the use of hook-rod constructs.189–191 Pseudarthrosis that is either caused by or has resulted in failure of the bone-implant interface is often addressed by extending the length of the fusion construct. In most cases, adequate thoracic stabilization can be achieved with dorsal constructs.216 However, in cases of ventral column destabilization, greater biomechanical stability is provided by a ventral column fusion and stabilization procedure.220,223 The addition of ventral interbody support is made difficult by the anatomic structures ventral to the thoracic spine and can be performed through transsternal, anterolateral, or posterolateral extrapleural thoracic approaches. Ventral stabilization constructs often require augmentation with a dorsal stabilization procedure.
Postarthrodesis Adjacent Segment Disease
Degeneration of adjacent segments is frequently observed in patients who have undergone spinal arthrodesis. Numerous long-term studies have demonstrated that degenerative changes in the motion segments cranial or caudal to a fusion are radiographically demonstrable in approximately 25% to 45% of patients,224–233 with some studies reporting an incidence as high as 60% to 90%.234–239 Adjacent segment degeneration may be manifested radiographically as mechanical instability, spondylolisthesis, degenerative disk disease, facet joint hypertrophy, ligamentous hypertrophy, or osteophytic spurs of the motion segments cranial or caudal to a fusion (Fig. 275-17; also see Fig. 275-11). Adjacent segment disease, however, which is defined as the development of symptoms of myelopathy, radiculopathy, or mechanical pain referable to pathology of an adjacent motion segment, occurs in only a minority of patients with radiographic evidence of adjacent segment degeneration. Accordingly, radiographically demonstrable adjacent segment degeneration has been poorly correlated with long-term clinical outcomes across multiple studies. Adjacent segment degeneration or disease may follow arthrodesis surgery regardless of whether successful fusion has occurred.
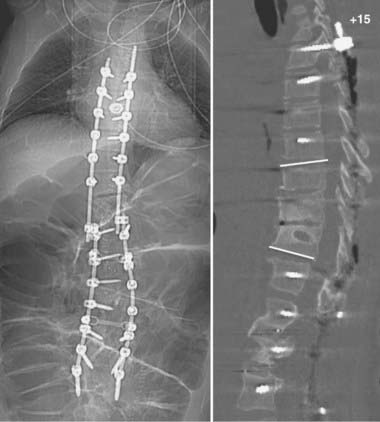
FIGURE 275-17 Postoperative anteroposterior (left) and sagittal (right) reconstructed computed tomography scans of the patient in Figure 275-11. After removal of the T11 pedicle screws, revision of the T11-12 fusion, internal reduction of the T10-11 kyphoscoliotic deformity, T5-12 posterior fusion, and screw-rod stabilization were performed. A sagittal 52-degree correction of the deformity was accomplished.
Although the precise cause of adjacent segment degeneration remains a subject of debate, it is apparent that the accelerated degenerative changes occurring at motion segments above or below a fusion are the result of increased biomechanical stress and the resultant hypermobility of motion segments cranial and caudal to a fusion.234,240–245 Biomechanical factors that have been implicated include increased facet loading, elevated intradiscal pressure, redistribution of mobility to the juxtafused segments, and the exaggerated bending moments and shear strain exerted by the lengthened lever arm of the fused segments. Several authors have proposed that the natural progression of preexisting degenerative spinal disease and these patients’ propensity for the development of spinal stenosis or instability are often superimposed on the increased biomechanical stress and accelerated degeneration that occur at adjacent motion segments.224,227,228,238,246 There remains an ongoing debate in the literature with regard to the relative contributions of the natural history of progressive degenerative spinal disease versus the altered kinematics and accelerated degeneration of motion segments adjacent to a fusion.247
The incidence of radiographically demonstrable adjacent segment degenerative changes after cervical fusion ranges from approximately 25% to 90%.225,226,234,235,241,248,249 The incidence of symptomatic adjacent segment disease after cervical fusion appears to be much lower, with reports ranging from 6% to 26% in patients with long-term follow-up.224,226,235,239,249,250 Hilibrand and colleagues demonstrated an incidence of symptomatic adjacent segment disease of 2.9% per year in the first 10 years after anterior cervical fusion.224 Radiographically apparent adjacent segment degeneration after lumbar fusion has been described more extensively in the literature, with a reported incidence of approximately 23% to 70% in patients with long-term radiographic follow-up.228,230,251–253 Symptomatic adjacent segment disease after lumbar fusions requiring reoperation has been reported to occur in approximately 3% to 27% of patients.229,251,254–256 Among these studies, there is a diversity of surgical indications, surgical techniques, definitions of what constitutes significant degenerative changes, patient age, and the length of clinical and radiographic follow-up. This diversity, in part, accounts for the wide range of reported incidences of both symptomatic and radiographically apparent adjacent segment degeneration. Although the true risk for adjacent segment disease is difficult to assess, it is clear that radiographically demonstrable adjacent segment degenerative changes, although common, are clinically relevant in a minority of patients harboring them. In general, symptomatic adjacent segment disease is most commonly observed to occur from 2 to 15 years after the index fusion procedure.257 Preexisting degenerative changes, such as spinal stenosis or disk herniation adjacent to an intended fusion, have been correlated with more rapid development of adjacent segment disease after arthrodesis.224
Treatment
Cervical Procedures
Adjacent segment disease may occur after anterior or posterior cervical fusions. The location of the neural element compression and the type of arthrodesis that was performed previously largely dictate the choice of approach for a revision operation. In cases in which neural element compression or kyphotic degeneration is present at nonadjacent levels, a multilevel procedure should be considered to prevent the future development of adjacent segment disease at these levels (Figs. 275-18 and 275-19). After anterior fusion, ventral pathology of the adjacent segments, such as osteophytic spurs or disk herniations, should be directly addressed by performing ventral decompression and fusion. In patients with extensive ventral osseous compression, excellent results have been obtained by performing corpectomies followed by strut grafting and stabilization.38,79,258,259
Lumbar Procedures
Adjacent segment disease after either dorsal or ventral lumbar fusion can often be successfully managed from a dorsal approach (Figs. 275-20 and 275-21). In addition to providing access for both dorsal and ventral decompression of the neural elements, circumferential fusion and stabilization are also readily accomplished via the dorsal approach. Although a simple decompression can be performed without fusion, the decision to avoid the additional morbidity associated with fusion and stabilization must be weighed carefully against the risk for spinal destabilization and the development of deformity. Noninstrumented dorsal fusion in the setting of lumbar adjacent segment disease carries an 80% risk for nonunion as opposed to 17% when instrumentation is used.260
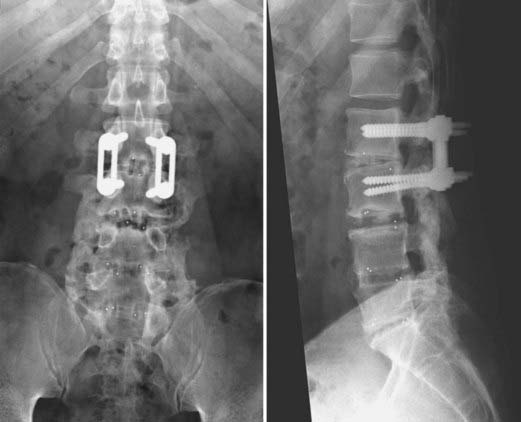
FIGURE 275-21 Postoperative anteroposterior (left) and lateral (right) radiographs of the patient in Figure 275-20 after posterior decompression and L2-3 posterior lumbar interbody fusion. A solid L3-S1 fusion mass was demonstrated intraoperatively, and the previous instrumentation construct was able to be removed.
Acosta FLJr, Aryan HE, Chou D, et al. Long-term biomechanical stability and clinical improvement after extended multilevel corpectomy and circumferential reconstruction of the cervical spine using titanium mesh cages. J Spinal Disord Tech. 2008;21:165-174.
Albert TJ, Vacarro A. Postlaminectomy kyphosis. Spine. 1998;23:2738-2745.
Benglis D, Wang MY, Levi AD. A comprehensive review of the safety profile of bone morphogenetic protein in spine surgery. Neurosurgery. 2008;62(5 suppl 2):ONS423-ONS431.
Bohlman HH, Emery SE, Goodfellow DB, et al. Robinson anterior cervical discectomy and arthrodesis for cervical radiculopathy. Long-term follow-up of one hundred and twenty-two patients. J Bone Joint Surg Am. 1993;75:1298-1307.
Carreon LY, Djurasovic M, Glassman SD, et al. Diagnostic accuracy and reliability of fine-cut CT scans with reconstructions to determine the status of an instrumented posterolateral fusion with surgical exploration as reference standard. Spine. 2007;32:892-895.
Carreon L, Glassman SD, Campbell MJ. Treatment of anterior cervical pseudoarthrosis: posterior fusion versus anterior revision. Spine J. 2006;6:154-156.
Cassinelli EH, Wallach C, Hanscom B, et al. Prospective clinical outcomes of revision fusion surgery in patients with pseudarthrosis after posterior lumbar interbody fusions using stand-alone metallic cages. Spine J. 2006;6:428-434.
Deutsch H, Haid RW, Rodts GE, et al. Postlaminectomy cervical deformity. Neurosurg Focus. 2003;15(3):E5.
Etebar S, Cahill DW. Risk factors for adjacent-segment failure following lumbar fixation with rigid instrumentation for degenerative instability. J Neurosurg. 1999;90(2 suppl):163-169.
Gertzbein SD, Hollopeter MR, Hall S. Pseudarthrosis of the lumbar spine. Outcome after circumferential fusion. Spine. 1998;23:2352-2356.
Gill JB, Levin A, Burd T, et al. Corrective osteotomies in spine surgery. J Bone Joint Surg Am. 2008;90:2509-2520.
Glassman SD, Minkow RE, Dimar JR, et al. Effect of prior lumbar discectomy on outcome of lumbar fusion: a prospective analysis using the SF-36 measure. J Spinal Disord. 1998;11:383-388.
Goffin J, Geusens E, Vantomme N, et al. Long-term follow-up after interbody fusion of the cervical spine. J Spinal Disord Tech. 2004;17:79-85.
Gore DR, Sepic SB. Anterior discectomy and fusion for painful cervical disc disease. A report of 50 patients with an average follow-up of 21 years. Spine. 1998;23:2047-2051.
Gore DR, Sepic SB. Anterior cervical fusion for degenerated or protruded discs. A review of one hundred forty-six patients. Spine. 1984;9:667-671.
Herman JM, Sonntag VK. Cervical corpectomy and plate fixation for postlaminectomy kyphosis. J Neurosurg. 1994;80:963-970.
Hilibrand AS, Carlson GD, Palumbo MA, et al. Radiculopathy and myelopathy at segments adjacent to the site of a previous anterior cervical arthrodesis. J Bone Joint Surg Am. 1999;81:519-528.
Hilibrand AS, Dina TS. The use of diagnostic imaging to assess spinal arthrodesis. Orthop Clin North Am. 1998;29:591-601.
Hilibrand AS, Fye MA, Emery SE, et al. Impact of smoking on the outcome of anterior cervical arthrodesis with interbody or strut-grafting. J Bone Joint Surg Am. 2001;83:668-673.
Iguchi T, Kurihara A, Nakayama J, et al. Minimum 10-year outcome of decompressive laminectomy for degenerative lumbar spinal stenosis. Spine. 2000;25:1754-1759.
Kaptain GJ, Simmons NE, Replogle RE, et al. Incidence and outcome of kyphotic deformity following laminectomy for cervical spondylotic myelopathy. J Neurosurg. 2000;93(2 suppl):199-204.
Kaufman HH, Jones E. The principles of bony spinal fusion. Neurosurgery. 1989;24:264-270.
Kim YJ, Lenke LG, Cho SK, et al. Comparative analysis of pedicle screw versus hook instrumentation in posterior spinal fusion of adolescent idiopathic scoliosis. Spine. 2004;29:2040-2048.
Kulkarni V, Rajshekhar V, Raghuram L. Accelerated spondylotic changes adjacent to the fused segment following central cervical corpectomy: magnetic resonance imaging study evidence. J Neurosurg. 2004;100(1 suppl Spine):2-6.
Mummaneni PV, Dhall SS, Rodts GE, et al. Circumferential fusion for cervical kyphotic deformity. J Neurosurg Spine. 2008;9:515-521.
Ondra SL, Marzouk S. Revision strategies for lumbar pseudarthrosis. Neurosurg Focus. 2003;15(3):E9.
Resnick DK, Choudhri TF, Dailey AT, et al. American Association of Neurological Surgeons/Congress of Neurological Surgeons. Guidelines for the performance of fusion procedures for degenerative disease of the lumbar spine. Part 12: pedicle screw fixation as an adjunct to posterolateral fusion for low-back pain. J Neurosurg Spine. 2005;2:700-706.
Resnick DK, Choudhri TF, Dailey AT, et al. American Association of Neurological Surgeons/Congress of Neurological Surgeons. Guidelines for the performance of fusion procedures for degenerative disease of the lumbar spine. Part 4: radiographic assessment of fusion. J Neurosurg Spine. 2005;2:653-657.
Steinmann JC, Herkowitz HN. Pseudarthrosis of the spine. Clin Orthop Relat Res. 1992;284:80-90.
Stewart TJ, Steinmetz MP, Benzel EC. Techniques for the ventral correction of postsurgical cervical kyphotic deformity. Neurosurgery. 2005;56(1 suppl):191-195.
Whitecloud TS3rd, Davis JM, Olive PM. Operative treatment of the degenerated segment adjacent to a lumbar fusion. Spine. 1994;19:531-536.
Yorimitsu E, Chiba K, Toyama Y, et al. Long-term outcomes of standard discectomy for lumbar disc herniation: a follow-up study of more than 10 years. Spine. 2001;26:652-657.
Yuan HA, Garfin SR, Dickman CA, et al. A historical cohort study of pedicle screw fixation in thoracic, lumbar, and sacral spinal fusions. Spine. 1994;19(20 suppl):2279S-2296S.
1 Erbayraktar S, Acar F, Tekinsoy B, et al. Outcome analysis of reoperations after lumbar discectomies: a report of 22 patients. Kobe J Med Sci. 2002;48:33-41.
2 Silvers HR, Lewis PJ, Asch HL, et al. Lumbar diskectomy for recurrent disk herniation. J Spinal Disord. 1994;7:408-419.
3 Glassman SD, Minkow RE, Dimar JR, et al. Effect of prior lumbar discectomy on outcome of lumbar fusion: a prospective analysis using the SF-36 measure. J Spinal Disord. 1998;11:383-388.
4 Santos ER, Pinto MR, Lonstein JE, et al. Revision lumbar arthrodesis for the treatment of lumbar cage pseudoarthrosis: complications. J Spinal Disord Tech. 2008;21:418-421.
5 Eichholz KM, Ryken TC. Complications of revision spinal surgery. Neurosurg Focus. 2003;15(3):E1.
6 Cammisa FPJr, Girardi FP, Sangani PK, et al. Incidental durotomy in spine surgery. Spine. 2000;25:2663-2667.
7 Fang A, Hu SS, Endres N, et al. Risk factors for infection after spinal surgery. Spine. 2005;30:1460-1465.
8 Hilibrand AS, Dina TS. The use of diagnostic imaging to assess spinal arthrodesis. Orthop Clin North Am. 1998;29:591-601.
9 Buchowski JM, Liu G, Bunmaprasert T, et al. Anterior cervical fusion assessment: surgical exploration versus radiographic evaluation. Spine. 2008;33:1185-1191.
10 Swartz KR, Trost GR. Recurrent lumbar disc herniation. Neurosurg Focus. 2003;15(3):E10.
11 Carragee EJ, Han MY, Suen PW, et al. Clinical outcomes after lumbar discectomy for sciatica: the effects of fragment type and anular competence. J Bone Joint Surg Am. 2003;85:102-108.
12 Cinotti G, Gumina S, Giannicola G, et al. Contralateral recurrent lumbar disc herniation. Results of discectomy compared with those in primary herniation. Spine. 1999;24:800-806.
13 Suk KS, Lee HM, Moon SH, et al. Recurrent lumbar disc herniation: results of operative management. Spine. 2001;26:672-676.
14 Davis RA. A long-term outcome analysis of 984 surgically treated herniated lumbar discs. J Neurosurg. 1994;80:415-421.
15 Yorimitsu E, Chiba K, Toyama Y, et al. Long-term outcomes of standard discectomy for lumbar disc herniation: a follow-up study of more than 10 years. Spine. 2001;26:652-657.
16 Malter AD, McNeney B, Loeser JD, et al. 5-year reoperation rates after different types of lumbar spine surgery. Spine. 1998;23:814-820.
17 Herron L. Recurrent lumbar disc herniation: results of repeat laminectomy and discectomy. J Spinal Disord. 1994;7:161-166.
18 Hueftle MG, Modic MT, Ross JS, et al. Lumbar spine: postoperative MR imaging with Gd-DTPA. Radiology. 1988;167:817-824.
19 Grane P. The postoperative lumbar spine. A radiological investigation of the lumbar spine after discectomy using MR imaging and CT. Acta Radiol Suppl. 1998;414:1-23.
20 Mirowitz SA, Shady KL. Gadopentetate dimeglumine–enhanced MR imaging of the postoperative lumbar spine: comparison of fat-suppressed and conventional T1-weighted images. AJR Am J Roentgenol. 1992;159:385-389.
21 Ross JS, Robertson JT, Frederickson RC, et al. Association between peridural scar and recurrent radicular pain after lumbar discectomy: magnetic resonance evaluation. ADCON-L European Study Group. Neurosurgery. 1996;38:855-861.
22 Fiume D, Sherkat S, Callovini GM, et al. Treatment of the failed back surgery syndrome due to lumbo-sacral epidural fibrosis. Acta Neurochir Suppl. 1995;64:116-118.
23 Ozgen S, Naderi S, Ozek MM, et al. Findings and outcome of revision lumbar disc surgery. J Spinal Disord. 1999;12:287-292.
24 Raynor RB, Pugh J, Shapiro I. Cervical facetectomy and its effect on spine strength. J Neurosurg. 1985;63:278-282.
25 Kelleher MO, Tan G, Sarjeant R, et al. Predictive value of intraoperative neurophysiological monitoring during cervical spine surgery: a prospective analysis of 1055 consecutive patients. J Neurosurg Spine. 2008;8:215-221.
26 Khan MH, Smith PN, Balzer JR, et al. Intraoperative somatosensory evoked potential monitoring during cervical spine corpectomy surgery: experience with 508 cases. Spine. 2006;31:E105-E113.
27 Smith PN, Balzer JR, Khan MH, et al. Intraoperative somatosensory evoked potential monitoring during anterior cervical discectomy and fusion in nonmyelopathic patients—a review of 1,039 cases. Spine J. 2007;7:83-87.
28 Deutsch H, Arginteanu M, Manhart K, et al. Somatosensory evoked potential monitoring in anterior thoracic vertebrectomy. J Neurosurg. 2000;92(2 suppl):155-161.
29 Schwartz DM, Auerbach JD, Dormans JP, et al. Neurophysiological detection of impending spinal cord injury during scoliosis surgery. J Bone Joint Surg Am. 2007;89:2440-2449.
30 Kim DH, Zaremski J, Kwon B, et al. Risk factors for false positive transcranial motor evoked potential monitoring alerts during surgical treatment of cervical myelopathy. Spine. 2007;32:3041-3046.
31 Lee JY, Hilibrand AS, Lim MR, et al. Characterization of neurophysiologic alerts during anterior cervical spine surgery. Spine. 2006;31:1916-1922.
32 May DM, Jones SJ, Crockard HA. Somatosensory evoked potential monitoring in cervical surgery: identification of pre- and intraoperative risk factors associated with neurological deterioration. J Neurosurg. 1996;85:566-573.
33 Cho WS, Chung CK, Jahng TA, et al. Post-laminectomy kyphosis in patients with cervical ossification of the posterior longitudinal ligament: does it cause neurological deterioration? J Korean Neurosurg Soc. 2008;43:259-264.
34 Guigui P, Benoist M, Deburge A. Spinal deformity and instability after multilevel cervical laminectomy for spondylotic myelopathy. Spine. 1998;23:440-447.
35 Jenkins DH. Extensive cervical laminectomy. Long-term results. Br J Surg. 1973;60:852-854.
36 McLaughlin MR, Wahlig JB, Pollack IF. Incidence of postlaminectomy kyphosis after Chiari decompression. Spine. 1997;22:613-617.
37 Heller JG, Edwards CC2nd, Murakami H, et al. Laminoplasty versus laminectomy and fusion for multilevel cervical myelopathy: an independent matched cohort analysis. Spine. 2001;26:1330-1336.
38 Herman JM, Sonntag VK. Cervical corpectomy and plate fixation for postlaminectomy kyphosis. J Neurosurg. 1994;80:963-970.
39 Deutsch H, Haid RW, Rodts GE, et al. Postlaminectomy cervical deformity. Neurosurg Focus. 2003;15(3):E5.
40 Albert TJ, Vacarro A. Postlaminectomy kyphosis. Spine. 1998;23:2738-2745.
41 Saito T, Yamamuro T, Shikata J, et al. Analysis and prevention of spinal column deformity following cervical laminectomy. I. Pathogenetic analysis of postlaminectomy deformities. Spine. 1991;16:494-502.
42 Yasuoka S, Peterson HA, Laws ERJr, et al. Pathogenesis and prophylaxis of postlaminectomy deformity of the spine after multiple level laminectomy: difference between children and adults. Neurosurgery. 1981;9:145-152.
43 Herkowitz HN. A comparison of anterior cervical fusion, cervical laminectomy, and cervical laminoplasty for the surgical management of multiple level spondylotic radiculopathy. Spine. 1988;13:774-780.
44 Schlenk RP, Stewart T, Benzel EC. The biomechanics of iatrogenic spinal destabilization and implant failure. Neurosurg Focus. 2003;15(3):E2.
45 Mikawa Y, Shikata J, Yamamuro T. Spinal deformity and instability after multilevel cervical laminectomy. Spine. 1987;12:6-11.
46 Sciubba DM, Chaichana KL, Woodworth GF, et al. Factors associated with cervical instability requiring fusion after cervical laminectomy for intradural tumor resection. J Neurosurg Spine. 2008;8:413-419.
47 Kaptain GJ, Simmons NE, Replogle RE, et al. Incidence and outcome of kyphotic deformity following laminectomy for cervical spondylotic myelopathy. J Neurosurg. 2000;93(2 suppl):199-204.
48 Papagelopoulos PJ, Peterson HA, Ebersold MJ, et al. Spinal column deformity and instability after lumbar or thoracolumbar laminectomy for intraspinal tumors in children and young adults. Spine. 1997;22:442-451.
49 Katsumi Y, Honma T, Nakamura T. Analysis of cervical instability resulting from laminectomies for removal of spinal cord tumor. Spine. 1989;14:1171-1176.
50 Mummaneni PV, Dhall SS, Rodts GE, et al. Circumferential fusion for cervical kyphotic deformity. J Neurosurg Spine. 2008;9:515-521.
51 Butler JC, Whitecloud TS3rd. Postlaminectomy kyphosis. Causes and surgical management. Orthop Clin North Am. 1992;23:505-511.
52 Steinmetz MP, Stewart TJ, Kager CD, et al. Cervical deformity correction. Neurosurgery. 2007;60(1 supp1 1):S90-S97.
53 Stewart TJ, Steinmetz MP, Benzel EC. Techniques for the ventral correction of postsurgical cervical kyphotic deformity. Neurosurgery. 2005;56(1 suppl):191-195.
54 Francis WRJr, Noble DP. Treatment of cervical kyphosis in children. Spine. 1988;13:883-887.
55 Cloward RB. The anterior approach for removal of ruptured cervical disks. J Neurosurg. 1958;15:602-617.
56 Benzel EC. Spine Surgery: Techniques, Complication Avoidance, and Management, 2nd ed. Philadelphia: Churchill Livingstone; 2005.
57 Sevki K, Mehmet T, Ufuk T, et al. Results of surgical treatment for degenerative cervical myelopathy: anterior cervical corpectomy and stabilization. Spine. 2004;29:2493-2500.
58 Singh K, Vaccaro AR, Kim J, et al. Biomechanical comparison of cervical spine reconstructive techniques after a multilevel corpectomy of the cervical spine. Spine. 2003;28:2352-2358.
59 Brodke DS, Klimo PJr, Bachus KN, et al. Anterior cervical fixation: analysis of load-sharing and stability with use of static and dynamic plates. J Bone Joint Surg Am. 2006;88:1566-1573.
60 Nunley PD, Jawahar A, Kerr EJ3rd, et al. Choice of plate may affect outcomes for single versus multilevel ACDF: results of a prospective randomized single-blind trial. Spine J. 2009;9:121-127.
61 Kaiser MG, Haid RWJr, Subach BR, et al. Anterior cervical plating enhances arthrodesis after discectomy and fusion with cortical allograft. Neurosurgery. 2002;50:229-236.
62 Zdeblick TA, Bohlman HH. Cervical kyphosis and myelopathy. Treatment by anterior corpectomy and strut-grafting. J Bone Joint Surg Am. 1989;71:170-182.
63 Jane JASr, Sherman JH, Boulos PT, et al. Lumbar stenosis: a personal record. Invited submission from the Joint Section Meeting on Disorders of the Spine and Peripheral Nerves, March 2004. J Neurosurg Spine. 2004;1:31-38.
64 Hilibrand AS, Rand N. Degenerative lumbar stenosis: diagnosis and management. J Am Acad Orthop Surg. 1999;7:239-249.
65 Weinstein JN, Tosteson TD, Lurie JD, et al. Surgical versus nonsurgical therapy for lumbar spinal stenosis. N Engl J Med. 2008;358:794-810.
66 Gibson JN, Waddell G. Surgery for degenerative lumbar spondylosis. Cochrane Database Syst Rev. 2005;2:CD001352.
67 Caputy AJ, Luessenhop AJ. Long-term evaluation of decompressive surgery for degenerative lumbar stenosis. J Neurosurg. 1992;77:669-676.
68 Konno S, Kikuchi S. Prospective study of surgical treatment of degenerative spondylolisthesis: comparison between decompression alone and decompression with graft system stabilization. Spine. 2000;25:1533-1537.
69 Iguchi T, Kurihara A, Nakayama J, et al. Minimum 10-year outcome of decompressive laminectomy for degenerative lumbar spinal stenosis. Spine. 2000;25:1754-1759.
70 Bambakidis NC, Feiz-Erfan I, Klopfenstein JD, et al. Indications for surgical fusion of the cervical and lumbar motion segment. Spine. 2005;30(16 suppl):S2-S6.
71 Resnick DK, Choudhri TF, Dailey AT, et al. American Association of Neurological Surgeons/Congress of Neurological Surgeons. Guidelines for the performance of fusion procedures for degenerative disease of the lumbar spine. Part 9: fusion in patients with stenosis and spondylolisthesis. J Neurosurg Spine. 2005;2:679-685.
72 Fox MW, Onofrio BM, Hanssen AD. Clinical outcomes and radiological instability following decompressive lumbar laminectomy for degenerative spinal stenosis: a comparison of patients undergoing concomitant arthrodesis versus decompression alone. J Neurosurg. 1996;85:793-802.
73 Jagannathan J, Sansur CA, Shaffrey CI. Iatrogenic spinal deformity. Neurosurgery. 2008;63(3 suppl):104-116.
74 Groth AT, Kuklo TR, Klemme WR, et al. Comparison of sagittal contour and posterior disc height following interbody fusion: threaded cylindrical cages versus structural allograft versus vertical cages. J Spinal Disord Tech. 2005;18:332-336.
75 Gödde S, Fritsch E, Dienst M, et al. Influence of cage geometry on sagittal alignment in instrumented posterior lumbar interbody fusion. Spine. 2003;28:1693-1699.
76 Heary RF, Kumar S, Karimi RJ. Dorsal lumbar interbody fusion for chronic axial, mechanical low back pain: a modification of two established techniques. Neurosurgery. 2008;63(1 suppl 1):ONS102-ONS106.
77 Resnick DK, Choudhri TF, Dailey AT, et al. American Association of Neurological Surgeons/Congress of Neurological Surgeons. Guidelines for the performance of fusion procedures for degenerative disease of the lumbar spine. Part 12: pedicle screw fixation as an adjunct to posterolateral fusion for low-back pain. J Neurosurg Spine. 2005;2:700-706.
78 Resnick DK, Choudhri TF, Dailey AT, et al. American Association of Neurological Surgeons/Congress of Neurological Surgeons. Guidelines for the performance of fusion procedures for degenerative disease of the lumbar spine. Part 4: radiographic assessment of fusion. J Neurosurg Spine. 2005;2:653-657.
79 Mayr MT, Subach BR, Comey CH, et al. Cervical spinal stenosis: outcome after anterior corpectomy, allograft reconstruction, and instrumentation. J Neurosurg. 2002;96(1 Suppl):10-16.
80 Kornblum MB, Fischgrund JS, Herkowitz HN, et al. Degenerative lumbar spondylolisthesis with spinal stenosis: a prospective long-term study comparing fusion and pseudarthrosis. Spine. 2004;29:726-733.
81 Thomsen K, Christensen FB, Eiskjaer SP, et al. 1997 Volvo Award winner in clinical studies. The effect of pedicle screw instrumentation on functional outcome and fusion rates in posterolateral lumbar spinal fusion: a prospective, randomized clinical study. Spine. 1997;22:2813-2822.
82 Vaccaro AR, Garfin SR. Internal fixation (pedicle screw fixation) for fusions of the lumbar spine. Spine. 1995;20(24 suppl):157S-165S.
83 Fischgrund JS, Mackay M, Herkowitz HN, et al. 1997 Volvo Award winner in clinical studies. Degenerative lumbar spondylolisthesis with spinal stenosis: a prospective, randomized study comparing decompressive laminectomy and arthrodesis with and without spinal instrumentation. Spine. 1997;22:2807-2812.
84 Wright IP, Eisenstein SM. Anterior cervical discectomy and fusion without instrumentation. Spine. 2007;32:772-774.
85 Lee C, Dorcil J, Radomisli TE. Nonunion of the spine: a review. Clin Orthop Relat Res. 2004;419:71-75.
86 Steinmann JC, Herkowitz HN. Pseudarthrosis of the spine. Clin Orthop Relat Res. 1992;284:80-90.
87 Blumenthal SL, Gill K. Can lumbar spine radiographs accurately determine fusion in postoperative patients? Correlation of routine radiographs with a second surgical look at lumbar fusions. Spine. 1993;18:1186-1189.
88 Burkus JK, Foley K, Haid RW, et al. Surgical Interbody Research Group—radiographic assessment of interbody fusion devices: fusion criteria for anterior lumbar interbody surgery. Neurosurg Focus. 2001;10(4):E11.
89 Carreon LY, Djurasovic M, Glassman SD, et al. Diagnostic accuracy and reliability of fine-cut CT scans with reconstructions to determine the status of an instrumented posterolateral fusion with surgical exploration as reference standard. Spine. 2007;32:892-895.
90 Hipp JA, Reitman CA, Wharton N. Defining pseudoarthrosis in the cervical spine with differing motion thresholds. Spine. 2005;30:209-210.
91 Jenkins LT, Jones AL, Harms JJ. Prognostic factors in lumbar spinal fusion. Contemp Orthop. 1994;29:173-180.
92 Pilitsis JG, Lucas DR, Rengachary SS. Bone healing and spinal fusion. Neurosurg Focus. 2002;13(6):E1.
93 Dimitriou R, Tsiridis E, Giannoudis PV. Current concepts of molecular aspects of bone healing. Injury. 2005;36:1392-1404.
94 Bielby R, Jones E, McGonagle D. The role of mesenchymal stem cells in maintenance and repair of bone. Injury. 2007;38(suppl 1):S26-S32.
95 Kaufman HH, Jones E. The principles of bony spinal fusion. Neurosurgery. 1989;24:264-270.
96 Goldberg VM, Stevenson S. The biology of bone grafts. Semin Arthroplasty. 1993;4:58-63.
97 Burchardt H. The biology of bone graft repair. Clin Orthop Relat Res. 1983;174:28-42.
98 Sandhu HS. Bone morphogenetic proteins and spinal surgery. Spine. 2003;28(15 suppl):S64-S73.
99 Burkus JK, Transfeldt EE, Kitchel SH, et al. Clinical and radiographic outcomes of anterior lumbar interbody fusion using recombinant human bone morphogenetic protein-2. Spine. 2002;27:2396-2408.
100 Haid RWJr, Branch CLJr, Alexander JT, et al. Posterior lumbar interbody fusion using recombinant human bone morphogenetic protein type 2 with cylindrical interbody cages. Spine J. 2004;4:527-538.
101 Tsiridis E, Upadhyay N, Giannoudis P. Molecular aspects of fracture healing: which are the important molecules? Injury. 2007;38(suppl 1):S11-S25.
102 Boden SD. The biology of posterolateral lumbar spinal fusion. Orthop Clin North Am. 1998;29:603-619.
103 Brandoff JF, Silber JS, Vaccaro AR. Contemporary alternatives to synthetic bone grafts for spine surgery. Am J Orthop. 2008;37:410-414.
104 Bauer TW, Muschler GF. Bone graft materials. An overview of the basic science. Clin Orthop Relat Res. 2000;371:10-27.
105 Bendo JA, Spivak J, Moskovich R, et al. Instrumented posterior arthrodesis of the lumbar spine in patients with diabetes mellitus. Am J Orthop. 2000;29:617-620.
106 Inaoka M, Tada K, Yonenobu K. Problems of posterior lumbar interbody fusion (PLIF) for the rheumatoid spondylitis of the lumbar spine. Arch Orthop Trauma Surg. 2002;122:73-79.
107 Kraus DR, Peppelman WC, Agarwal AK, et al. Incidence of subaxial subluxation in patients with generalized rheumatoid arthritis who have had previous occipital cervical fusions. Spine. 1991;16(10 suppl):S486-S489.
108 Clarke MJ, Cohen-Gadol AA, Ebersold MJ, et al. Long-term incidence of subaxial cervical spine instability following cervical arthrodesis surgery in patients with rheumatoid arthritis. Surg Neurol. 2006;66:136-140.
109 Zoma A, Sturrock RD, Fisher WD, et al. Surgical stabilisation of the rheumatoid cervical spine. A review of indications and results. J Bone Joint Surg Br. 1987;69:8-12.
110 Szpalski M, Gunzburg R. What are the advances for surgical therapy of inflammatory diseases of the spine? Best Pract Res Clin Rheumatol. 2002;16:141-154.
111 Duarte PM, Nogueira Filho GR, Sallum EA, et al. The effect of an immunosuppressive therapy and its withdrawal on bone healing around titanium implants. A histometric study in rabbits. J Periodontol. 2001;72:1391-1397.
112 Sawin PD, Dickman CA, Crawford NR, et al. The effects of dexamethasone on bone fusion in an experimental model of posterolateral lumbar spinal arthrodesis. J Neurosurg. 2001;94(1 suppl):76-81.
113 Simon AM, Manigrasso MB, O’Connor JP. Cyclo-oxygenase 2 function is essential for bone fracture healing. J Bone Miner Res. 2002;17:963-976.
114 Tortolani PJ, Park AE, Louis-Ugbo J, et al. The effects of doxorubicin (Adriamycin) on spinal fusion: an experimental model of posterolateral lumbar spinal arthrodesis. Spine J. 2004;4:669-674.
115 Thaller J, Walker M, Kline AJ, et al. The effect of nonsteroidal anti-inflammatory agents on spinal fusion. Orthopedics. 2005;28:299-303.
116 Riew KD, Long J, Rhee J, et al. Time-dependent inhibitory effects of indomethacin on spinal fusion. J Bone Joint Surg Am. 2003;85:632-634.
117 Singh K, An HS, Samartzis D, et al. A prospective cohort analysis of adjacent vertebral body bone mineral density in lumbar surgery patients with or without instrumented posterolateral fusion: a 9- to 12-year follow-up. Spine. 2005;30:1750-1755.
118 Prevrhal S, Genant HK. [Quantitative computer tomography]. Radiologe. 1999;39:194-202.
119 Guglielmi G, Lang TF. Quantitative computed tomography. Semin Musculoskelet Radiol. 2002;6:219-227.
120 Damilakis J, Maris TG, Karantanas AH. An update on the assessment of osteoporosis using radiologic techniques. Eur Radiol. 2007;17:1591-1602.
121 Genant HK, Engelke K, Prevrhal S. Advanced CT bone imaging in osteoporosis. Rheumatology (Oxford). 2008;47(suppl 4):iv9-iv16.
122 North American Menopause Society. The role of calcium in peri- and postmenopausal women: consensus opinion of The North American Menopause Society. Menopause. 2001;8:84-95.
123 Notelovitz M. Effects of estrogen/androgen therapy on bone mineral density parameters. J Reprod Med. 2001;46(3 suppl):325-331.
124 Huang RC, Khan SN, Sandhu HS, et al. Alendronate inhibits spine fusion in a rat model. Spine. 2005;30:2516-2522.
125 Xue Q, Li H, Zou X, et al. The influence of alendronate treatment and bone graft volume on posterior lateral spine fusion in a porcine model. Spine. 2005;30:1116-1121.
126 Lehman RAJr, Kuklo TR, Freedman BA, et al. The effect of alendronate sodium on spinal fusion: a rabbit model. Spine J. 2004;4:36-43.
127 Sama AA, Khan SN, Myers ER, et al. High-dose alendronate uncouples osteoclast and osteoblast function: a study in a rat spine pseudarthrosis model. Clin Orthop Relat Res. 2004;425:135-142.
128 Canalis E, Giustina A, Bilezikian JP. Mechanisms of anabolic therapies for osteoporosis. N Engl J Med. 2007;357:905-916.
129 Jerome CP, Johnson CS, Vafai HT, et al. Effect of treatment for 6 months with human parathyroid hormone (1-34) peptide in ovariectomized cynomolgus monkeys (Macaca fascicularis). Bone. 1999;25:301-309.
130 Thomas T. Intermittent parathyroid hormone therapy to increase bone formation. Joint Bone Spine. 2006;73:262-269.
131 Abe Y, Takahata M, Ito M, et al. Enhancement of graft bone healing by intermittent administration of human parathyroid hormone (1-34) in a rat spinal arthrodesis model. Bone. 2007;41:775-785.
132 O’Loughlin PF, Cunningham ME, Bukata SV, et al. Parathyroid hormone (1-34) augments spinal fusion, fusion mass volume, and fusion mass quality in a rabbit spinal fusion model. Spine. 2009;34:121-130.
133 Hanley ENJr, Levy JA. Surgical treatment of isthmic lumbosacral spondylolisthesis. Analysis of variables influencing results. Spine. 1989;14:48-50.
134 Brown CW, Orme TJ, Richardson HD. The rate of pseudarthrosis (surgical nonunion) in patients who are smokers and patients who are nonsmokers: a comparison study. Spine. 1986;11:942-943.
135 Glassman SD, Anagnost SC, Parker A, et al. The effect of cigarette smoking and smoking cessation on spinal fusion. Spine. 2000;25:2608-2615.
136 Hilibrand AS, Fye MA, Emery SE, et al. Impact of smoking on the outcome of anterior cervical arthrodesis with interbody or strut-grafting. J Bone Joint Surg Am. 2001;83:668-673.
137 Andersen T, Christensen FB, Laursen M, et al. Smoking as a predictor of negative outcome in lumbar spinal fusion. Spine. 2001;26:2623-2628.
138 Hadley MN, Reddy SV. Smoking and the human vertebral column: a review of the impact of cigarette use on vertebral bone metabolism and spinal fusion. Neurosurgery. 1997;41:116-124.
139 Daftari TK, Whitesides TEJr, Heller JG, et al. Nicotine on the revascularization of bone graft. An experimental study in rabbits. Spine. 1994;19:904-911.
140 Krall EA, Dawson-Hughes B. Smoking increases bone loss and decreases intestinal calcium absorption. J Bone Miner Res. 1999;14:215-220.
141 Silcox DH3rd, Daftari T, Boden SD, et al. The effect of nicotine on spinal fusion. Spine. 1995;20:1549-1553.
142 Wing KJ, Fisher CG, O’Connell JX, et al. Stopping nicotine exposure before surgery. The effect on spinal fusion in a rabbit model. Spine. 2000;25:30-34.
143 Ondra SL, Marzouk S. Revision strategies for lumbar pseudarthrosis. Neurosurg Focus. 2003;15(3):E9.
144 Etminan M, Girardi FP, Khan SN, et al. Revision strategies for lumbar pseudarthrosis. Orthop Clin North Am. 2002;33:381-392.
145 Albert TJ, Pinto M, Denis F. Management of symptomatic lumbar pseudarthrosis with anteroposterior fusion. A functional and radiographic outcome study. Spine. 2000;25:123-129.
146 Cassinelli EH, Wallach C, Hanscom B, et al. Prospective clinical outcomes of revision fusion surgery in patients with pseudarthrosis after posterior lumbar interbody fusions using stand-alone metallic cages. Spine J. 2006;6:428-434.
147 Malloy KM, Hilibrand AS. Autograft versus allograft in degenerative cervical disease. Clin Orthop Relat Res. 2002;394:27-38.
148 An HS, Simpson JM, Glover JM, et al. Comparison between allograft plus demineralized bone matrix versus autograft in anterior cervical fusion. A prospective multicenter study. Spine. 1995;20:2211-2216.
149 Martin GJJr, Haid RWJr, MacMillan M, et al. Anterior cervical discectomy with freeze-dried fibula allograft. Overview of 317 cases and literature review. Spine. 1999;24:852-858.
150 Samartzis D, Shen FH, Matthews DK, et al. Comparison of allograft to autograft in multilevel anterior cervical discectomy and fusion with rigid plate fixation. Spine J. 2003;3:451-459.
151 Gibson S, McLeod I, Wardlaw D, et al. Allograft versus autograft in instrumented posterolateral lumbar spinal fusion: a randomized control trial. Spine. 2002;27:1599-1603.
152 Floyd T, Ohnmeiss D. A meta-analysis of autograft versus allograft in anterior cervical fusion. Eur Spine J. 2000;9:398-403.
153 Buttermann GR. Prospective nonrandomized comparison of an allograft with bone morphogenic protein versus an iliac-crest autograft in anterior cervical discectomy and fusion. Spine J. 2008;8:426-435.
154 Wimmer C, Krismer M, Gluch H, et al. Autogenic versus allogenic bone grafts in anterior lumbar interbody fusion. Clin Orthop Relat Res. 1999;360:122-126.
155 Bishop RC, Moore KA, Hadley MN. Anterior cervical interbody fusion using autogeneic and allogeneic bone graft substrate: a prospective comparative analysis. J Neurosurg. 1996;85:206-210.
156 Zhang ZH, Yin H, Yang K, et al. Anterior intervertebral disc excision and bone grafting in cervical spondylotic myelopathy. Spine. 1983;8:16-19.
157 Vaccaro AR, Chiba K, Heller JG, et al. Bone grafting alternatives in spinal surgery. Spine J. 2002;2:206-215.
158 Ehrler DM, Vaccaro AR. The use of allograft bone in lumbar spine surgery. Clin Orthop Relat Res. 2000;371:38-45.
159 Heary RF, Schlenk RP, Sacchieri TA, et al. Persistent iliac crest donor site pain: independent outcome assessment. Neurosurgery. 2002;50:510-516.
160 Ahlmann E, Patzakis M, Roidis N, et al. Comparison of anterior and posterior iliac crest bone grafts in terms of harvest-site morbidity and functional outcomes. J Bone Joint Surg Am. 2002;84:716-720.
161 Schnee CL, Freese A, Weil RJ, et al. Analysis of harvest morbidity and radiographic outcome using autograft for anterior cervical fusion. Spine. 1997;22:2222-2227.
162 Samartzis D, Shen FH, Goldberg EJ, et al. Is autograft the gold standard in achieving radiographic fusion in one-level anterior cervical discectomy and fusion with rigid anterior plate fixation? Spine. 2005;30:1756-1761.
163 Gertzbein SD, Hollopeter MR, Hall S. Pseudarthrosis of the lumbar spine. Outcome after circumferential fusion. Spine. 1998;23:2352-2356.
164 Rengachary SS. Bone morphogenetic proteins: basic concepts. Neurosurg Focus. 2002;13(6):E2.
165 Wang EA, Rosen V, D’Alessandro JS, et al. Recombinant human bone morphogenetic protein induces bone formation. Proc Natl Acad Sci U S A. 1990;87:2220-2224.
166 Glassman SD, Carreon LY, Djurasovic M, et al. RhBMP-2 versus iliac crest bone graft for lumbar spine fusion: a randomized, controlled trial in patients over sixty years of age. Spine. 2008;33:2843-2849.
167 Benglis D, Wang MY, Levi AD. A comprehensive review of the safety profile of bone morphogenetic protein in spine surgery. Neurosurgery. 2008;62(5 suppl 2):ONS423-ONS431.
168 McClellan JW, Mulconrey DS, Forbes RJ, et al. Vertebral bone resorption after transforaminal lumbar interbody fusion with bone morphogenetic protein (rhBMP-2). J Spinal Disord Tech. 2006;19:483-486.
169 Joseph V, Rampersaud YR. Heterotopic bone formation with the use of rhBMP2 in posterior minimal access interbody fusion: a CT analysis. Spine. 2007;32:2885-2890.
170 Perri B, Cooper M, Lauryssen C, et al. Adverse swelling associated with use of rh-BMP-2 in anterior cervical discectomy and fusion: a case study. Spine J. 2007;7:235-239.
171 Kraus W, Lechner F. Healing of pseudoarthrosis and spontaneous fractures with structure-forming electrodynamic potentials. Munch Med Wochenschr. 1972;114:1814-1819.
172 Spadaro JA. Mechanical and electrical interactions in bone remodeling. Bioelectromagnetics. 1997;18:193-202.
173 Hodges SD, Eck JC, Humphreys SC. Use of electrical bone stimulation in spinal fusion. J Am Acad Orthop Surg. 2003;11:81-88.
174 Aaron RK, Ciombor DM, Simon BJ. Treatment of nonunions with electric and electromagnetic fields. Clin Orthop Relat Res. 2004;419:21-29.
175 Gan JC, Glazer PA. Electrical stimulation therapies for spinal fusions: current concepts. Eur Spine J. 2006;15:1301-1311.
176 Tsai MT, Chang WH, Chang K, et al. Pulsed electromagnetic fields affect osteoblast proliferation and differentiation in bone tissue engineering. Bioelectromagnetics. 2007;28:519-528.
177 Ciombor DM, Aaron RK. Influence of electromagnetic fields on endochondral bone formation. J Cell Biochem. 1993;52:37-41.
178 Ciombor DM, Aaron RK. The role of electrical stimulation in bone repair. Foot Ankle Clin. 2005;10:579-593. vii
179 Goodwin CB, Brighton CT, Guyer RD, et al. A double-blind study of capacitively coupled electrical stimulation as an adjunct to lumbar spinal fusions. Spine. 1999;24:1349-1356.
180 Linovitz RJ, Pathria M, Bernhardt M, et al. Combined magnetic fields accelerate and increase spine fusion: a double-blind, randomized, placebo controlled study. Spine. 2002;27:1383-1389.
181 Kahanovitz N. Electrical stimulation of spinal fusion: a scientific and clinical update. Spine J. 2002;2:145-150.
182 Simmons JWJr, Mooney V, Thacker I. Pseudarthrosis after lumbar spine fusion: nonoperative salvage with pulsed electromagnetic fields. Am J Orthop. 2004;33:27-30.
183 Morone MA, Feuer H. The use of electrical stimulation to enhance spinal fusion. Neurosurg Focus. 2002;13:E5.
184 Kane WJ. Direct current electrical bone growth stimulation for spinal fusion. Spine. 1988;13:363-365.
185 Welch WC, Willis SL, Gerszten PC. Implantable direct current stimulation in para-axial cervical arthrodesis. Adv Ther. 2004;21:389-400.
186 Foley KT, Mroz TE, Arnold PM, et al. Randomized, prospective, and controlled clinical trial of pulsed electromagnetic field stimulation for cervical fusion. Spine J. 2008;8:436-442.
187 Goodman S, Aspenberg P. Effects of mechanical stimulation on the differentiation of hard tissues. Biomaterials. 1993;14:563-569.
188 Kim YJ, Lenke LG, Cho SK, et al. Comparative analysis of pedicle screw versus hook instrumentation in posterior spinal fusion of adolescent idiopathic scoliosis. Spine. 2004;29:2040-2048.
189 Hackenberg L, Link T, Liljenqvist U. Axial and tangential fixation strength of pedicle screws versus hooks in the thoracic spine in relation to bone mineral density. Spine. 2002;27:937-942.
190 Yuan HA, Garfin SR, Dickman CA, et al. A historical cohort study of pedicle screw fixation in thoracic, lumbar, and sacral spinal fusions. Spine. 1994;19(20 suppl):2279S-2296S.
191 Yahiro MA. Comprehensive literature review. Pedicle screw fixation devices. Spine. 1994;19(20 suppl):2274S-2278S.
192 Hostin RA, Wu C, Perra JH, et al. A biomechanical evaluation of three revision screw strategies for failed lateral mass fixation. Spine. 2008;33:2415-2421.
193 Kiner DW, Wybo CD, Sterba W, et al. Biomechanical analysis of different techniques in revision spinal instrumentation: larger diameter screws versus cement augmentation. Spine. 2008;33:2618-2622.
194 Gilad R, Gandhi CD, Arginteanu MS, et al. Uncorrected sagittal plane imbalance predisposes to symptomatic instrumentation failure. Spine J. 2008;8:911-917.
195 Kim YJ, Bridwell KH, Lenke LG, et al. Pseudarthrosis in long adult spinal deformity instrumentation and fusion to the sacrum: prevalence and risk factor analysis of 144 cases. Spine. 2006;31:2329-2336.
196 Gill JB, Levin A, Burd T, et al. Corrective osteotomies in spine surgery. J Bone Joint Surg Am. 2008;90:2509-2520.
197 Mummaneni PV, Dhall SS, Ondra SL, et al. Pedicle subtraction osteotomy. Neurosurgery. 2008;63(3 suppl):171-176.
198 Cunningham BW, Polly DWJr. The use of interbody cage devices for spinal deformity: a biomechanical perspective. Clin Orthop Relat Res. 2002;394:73-83.
199 Polikeit A, Ferguson SJ, Nolte LP, et al. The importance of the endplate for interbody cages in the lumbar spine. Eur Spine J. 2003;12:556-561.
200 Rapoff AJ, O’Brien TJ, Ghanayem AJ, et al. Anterior cervical graft and plate load sharing. J Spinal Disord. 1999;12:45-49.
201 Acosta FLJr, Aryan HE, Chou D, et al. Long-term biomechanical stability and clinical improvement after extended multilevel corpectomy and circumferential reconstruction of the cervical spine using titanium mesh cages. J Spinal Disord Tech. 2008;21:165-174.
202 Brodke DS, Gollogly S, Alexander Mohr R, et al. Dynamic cervical plates: biomechanical evaluation of load sharing and stiffness. Spine. 2001;26:1324-1329.
203 Rhee JM, Riew KD. Dynamic anterior cervical plates. J Am Acad Orthop Surg. 2007;15:640-646.
204 Kant AP, Daum WJ, Dean SM, et al. Evaluation of lumbar spine fusion. Plain radiographs versus direct surgical exploration and observation. Spine. 1995;20:2313-2317.
205 Kuhns CA, Geck MJ, Wang JC, et al. An outcomes analysis of the treatment of cervical pseudarthrosis with posterior fusion. Spine. 2005;30:2424-2429.
206 Lowery GL, Swank ML, McDonough RF. Surgical revision for failed anterior cervical fusions. Articular pillar plating or anterior revision? Spine. 1995;20:2436-2441.
207 Tribus CB, Corteen DP, Zdeblick TA. The efficacy of anterior cervical plating in the management of symptomatic pseudoarthrosis of the cervical spine. Spine. 1999;24:860-864.
208 Carreon L, Glassman SD, Campbell MJ. Treatment of anterior cervical pseudoarthrosis: posterior fusion versus anterior revision. Spine J. 2006;6:154-156.
209 Thongtrangan I, Balabhadra RS, Kim DH. Management of strut graft failure in anterior cervical spine surgery. Neurosurg Focus. 2003;15(3):E4.
210 Lee MJ, Bazaz R, Furey CG, et al. Risk factors for dysphagia after anterior cervical spine surgery: a two-year prospective cohort study. Spine J. 2007;7:141-147.
211 Paniello RC, Martin-Bredahl KJ, Henkener LJ, et al. Preoperative laryngeal nerve screening for revision anterior cervical spine procedures. Ann Otol Rhinol Laryngol. 2008;117:594-597.
212 Johnston TL, Karaikovic EE, Lautenschlager EP, et al. Cervical pedicle screws vs. lateral mass screws: uniplanar fatigue analysis and residual pullout strengths. Spine J. 2006;6:667-672.
213 Jones EL, Heller JG, Silcox DH, et al. Cervical pedicle screws versus lateral mass screws. Anatomic feasibility and biomechanical comparison. Spine. 1997;22:977-982.
214 Kothe R, Rüther W, Schneider E, et al. Biomechanical analysis of transpedicular screw fixation in the subaxial cervical spine. Spine. 2004;29:1869-1875.
215 Brau SA, Delamarter RB, Kropf MA, et al. Access strategies for revision in anterior lumbar surgery. Spine. 2008;33:1662-1667.
216 Berven S, Kao H, Deviren V, et al. Treatment of thoracic pseudarthrosis in the adult: is combined surgery necessary? Clin Orthop Relat Res. 2003;411:25-31.
217 Lapsiwala S, Benzel E. Surgical management of cervical myelopathy dealing with the cervical-thoracic junction. Spine J. 2006;6(6 suppl):268S-273S.
218 Ames CP, Bozkus MH, Chamberlain RH, et al. Biomechanics of stabilization after cervicothoracic compression-flexion injury. Spine. 2005;30:1505-1512.
219 Prybis BG, Tortolani PJ, Hu N, et al. A comparative biomechanical analysis of spinal instability and instrumentation of the cervicothoracic junction: an in vitro human cadaveric model. J Spinal Disord Tech. 2007;20:233-238.
220 Heary RF, Salas S, Bono CM, et al. Complication avoidance: thoracolumbar and lumbar burst fractures. Neurosurg Clin N Am. 2006;17:377-388. viii
221 Resnick DK, Weller SJ, Benzel EC. Biomechanics of the thoracolumbar spine. Neurosurg Clin N Am. 1997;8:455-469.
222 McLain RF. The biomechanics of long versus short fixation for thoracolumbar spine fractures. Spine. 2006;31(11 suppl):S70-S79.
223 Bence T, Schreiber U, Grupp T, et al. Two column lesions in the thoracolumbar junction: anterior, posterior or combined approach? A comparative biomechanical in vitro investigation. Eur Spine J. 2007;16:813-820.
224 Hilibrand AS, Carlson GD, Palumbo MA, et al. Radiculopathy and myelopathy at segments adjacent to the site of a previous anterior cervical arthrodesis. J Bone Joint Surg Am. 1999;81:519-528.
225 Baba H, Furusawa N, Imura S, et al. Late radiographic findings after anterior cervical fusion for spondylotic myeloradiculopathy. Spine. 1993;18:2167-2173.
226 Gore DR, Sepic SB. Anterior discectomy and fusion for painful cervical disc disease. A report of 50 patients with an average follow-up of 21 years. Spine. 1998;23:2047-2051.
227 Gore DR, Sepic SB. Anterior cervical fusion for degenerated or protruded discs. A review of one hundred forty-six patients. Spine. 1984;9:667-671.
228 Wai EK, Santos ER, Morcom RA, et al. Magnetic resonance imaging 20 years after anterior lumbar interbody fusion. Spine. 2006;31:1952-1956.
229 Ghiselli G, Wang JC, Bhatia NN, et al. Adjacent segment degeneration in the lumbar spine. J Bone Joint Surg Am. 2004;86:1497-1503.
230 Lehmann TR, Spratt KF, Tozzi JE, et al. Long-term follow-up of lower lumbar fusion patients. Spine. 1987;12:97-104.
231 Lee CK. Accelerated degeneration of the segment adjacent to a lumbar fusion. Spine. 1988;13:375-377.
232 Okuda S, Iwasaki M, Miyauchi A, et al. Risk factors for adjacent segment degeneration after PLIF. Spine. 2004;29:1535-1540.
233 Cheh G, Bridwell KH, Lenke LG, et al. Adjacent segment disease following lumbar/thoracolumbar fusion with pedicle screw instrumentation: a minimum 5-year follow-up. Spine. 2007;32:2253-2257.
234 Dohler JR, Kahn MR, Hughes SP. Instability of the cervical spine after anterior interbody fusion. A study on its incidence and clinical significance in 21 patients. Arch Orthop Trauma Surg. 1985;104:247-250.
235 Goffin J, Geusens E, Vantomme N, et al. Long-term follow-up after interbody fusion of the cervical spine. J Spinal Disord Tech. 2004;17:79-85.
236 Hunter LY, Braunstein EM, Bailey RW. Radiographic changes following anterior cervical fusion. Spine. 1980;5:399-401.
237 Kulkarni V, Rajshekhar V, Raghuram L. Accelerated spondylotic changes adjacent to the fused segment following central cervical corpectomy: magnetic resonance imaging study evidence. J Neurosurg. 2004;100(1 suppl Spine):2-6.
238 Park P, Garton HJ, Gala VC, et al. Adjacent segment disease after lumbar or lumbosacral fusion: review of the literature. Spine. 2004;29:1938-1944.
239 Ishihara H, Kanamori M, Kawaguchi Y, et al. Adjacent segment disease after anterior cervical interbody fusion. Spine J. 2004;4:624-628.
240 Eck JC, Humphreys SC, Lim TH, et al. Biomechanical study on the effect of cervical spine fusion on adjacent-level intradiscal pressure and segmental motion. Spine. 2002;27:2431-2434.
241 Katsuura A, Hukuda S, Saruhashi Y, et al. Kyphotic malalignment after anterior cervical fusion is one of the factors promoting the degenerative process in adjacent intervertebral levels. Eur Spine J. 2001;10:320-324.
242 Cheng JS, Liu F, Komistek RD, et al. Comparison of cervical spine kinematics using a fluoroscopic model for adjacent segment degeneration. Invited submission from the Joint Section on Disorders of the Spine and Peripheral Nerves, March 2007. J Neurosurg Spine. 2007;7:509-513.
243 Park DH, Ramakrishnan P, Cho TH, et al. Effect of lower two-level anterior cervical fusion on the superior adjacent level. J Neurosurg Spine. 2007;7:336-340.
244 Levin DA, Hale JJ, Bendo JA. Adjacent segment degeneration following spinal fusion for degenerative disc disease. Bull NYU Hosp Jt Dis. 2007;65:29-36.
245 Dmitriev AE, Kuklo TR, Lehman RAJr, et al. Stabilizing potential of anterior, posterior, and circumferential fixation for multilevel cervical arthrodesis: an in vitro human cadaveric study of the operative and adjacent segment kinematics. Spine. 2007;32:E188-E196.
246 Hilibrand AS, Robbins M. Adjacent segment degeneration and adjacent segment disease: the consequences of spinal fusion? Spine J. 2004;4(6 suppl):190S-194S.
247 Axelsson P, Johnsson R, Stromqvist B. Adjacent segment hypermobility after lumbar spine fusion: no association with progressive degeneration of the segment 5 years after surgery. Acta Orthop. 2007;78:834-839.
248 Herkowitz HN, Kurz LT, Overholt DP. Surgical management of cervical soft disc herniation. A comparison between the anterior and posterior approach. Spine. 1990;15:1026-1030.
249 Yue WM, Brodner W, Highland TR. Long-term results after anterior cervical discectomy and fusion with allograft and plating: a 5- to 11-year radiologic and clinical follow-up study. Spine. 2005;30:2138-2144.
250 Bohlman HH, Emery SE, Goodfellow DB, et al. Robinson anterior cervical discectomy and arthrodesis for cervical radiculopathy. Long-term follow-up of one hundred and twenty-two patients. J Bone Joint Surg Am. 1993;75:1298-1307.
251 Gillet P. The fate of the adjacent motion segments after lumbar fusion. J Spinal Disord Tech. 2003;16:338-345.
252 Kumar MN, Jacquot F, Hall H. Long-term follow-up of functional outcomes and radiographic changes at adjacent levels following lumbar spine fusion for degenerative disc disease. Eur Spine J. 2001;10:309-313.
253 Ishihara H, Osada R, Kanamori M, et al. Minimum 10-year follow-up study of anterior lumbar interbody fusion for isthmic spondylolisthesis. J Spinal Disord. 2001;14:91-99.
254 Ghiselli G, Wang JC, Hsu WK, et al. L5-S1 segment survivorship and clinical outcome analysis after L4-L5 isolated fusion. Spine. 2003;28:1275-1280.
255 Kuslich SD, Danielson G, Dowdle JD, et al. Four-year follow-up results of lumbar spine arthrodesis using the Bagby and Kuslich lumbar fusion cage. Spine. 2000;25:2656-2662.
256 Etebar S, Cahill DW. Risk factors for adjacent-segment failure following lumbar fixation with rigid instrumentation for degenerative instability. J Neurosurg. 1999;90(2 suppl):163-169.
257 Schlegel JD, Smith JA, Schleusener RL. Lumbar motion segment pathology adjacent to thoracolumbar, lumbar, and lumbosacral fusions. Spine. 1996;21:970-981.
258 Hilibrand AS, Yoo JU, Carlson GD, et al. The success of anterior cervical arthrodesis adjacent to a previous fusion. Spine. 1997;22:1574-1579.
259 Bernard TNJr, Whitecloud TS3rd. Cervical spondylotic myelopathy and myeloradiculopathy. Anterior decompression and stabilization with autogenous fibula strut graft. Clin Orthop Relat Res. 1987;221:149-160.
260 Whitecloud TS3rd, Davis JM, Olive PM. Operative treatment of the degenerated segment adjacent to a lumbar fusion. Spine. 1994;19:531-536.