Chapter 99 Disorders of Micturition and Defecation
Disorders of Micturition
Micturition is an intricate function that results from a coordinated activity of the muscles of the urinary bladder and urethral sphincter. Knowledge, particularly of normal mechanisms, is accruing primarily from animal studies. Volitional and automatic functions are integrated [Bradley, 1986]. The automatic activities are controlled by the autonomic nervous system. In infants, autonomic control is primary, and as development proceeds, voluntary control over micturition is exerted. Voiding is frequent and largely uninhibited for the first 16 months of life. However, recent studies have found that there is cortical arousal in response to a full bladder, even in newborns [Yeung et al., 1995]. Voiding frequency gradually decreases, and between 1 and 2 years of age, the child becomes aware of the sensation of a full bladder. By 2 years of age, children begin to retain their urine for brief periods, although most children cannot willfully initiate voiding. By 3 years of age, diurnal control is established, and most children acquire nocturnal control by age 4 years. By 5 years of age, 85 percent of children have achieved complete bladder control [Himsel and Hurwitz, 1991]. Bowel control precedes bladder control with the sequence of control as follows:
Toilet training is necessary for achieving continence. As a general rule, children are ready for toilet training when they can appreciate the sensation of voiding and recognize a wet or soiled diaper. Similarly, ability to remain largely dry through an afternoon nap is a sign that a child is ready to stop wearing a diaper at night. Toilet training is a complex process and includes not only the recognition of an urge to void but also the ability to undress, void, wipe, dress again, flush, and wash hands [Stadtler et al., 1999]. Isolated day wetting or wetting both day and night is urinary incontinence. Nocturnal enuresis, or more commonly enuresis (derived from the Greek word enourein, “to void urine”), is usually used to denote bedwetting only in sleep. In nocturnal enuresis, there is a mismatch between the nocturnal bladder capacity and the amount of urine produced during the night, as well as an inability of the child to wake up in response to a full bladder. In one study of micturition habits of healthy children, 12 percent of boys and 7 percent of girls at 7 years of age, and 0.3 percent of boys and 0.6 percent of girls at 17 years of age, reported bedwetting [Hellström et al., 1995]. In another study, daytime urinary incontinence (at least once a month) occurred in 6.3 percent of first graders and 4.3 percent of fourth graders. Bedwetting (at least once a month) was reported in 7.1 and 2.7 percent, respectively. In first graders, fecal incontinence was present in 9.8 percent, and in fourth graders, 5.6 percent. Daytime urinary incontinence was associated strongly with fecal incontinence [Soderstrom et al., 2004].
Anatomy and Embryology
The urinary bladder, a hollow viscus, receives urine from the kidneys through the two ureteral orifices, and discharges urine through a solitary urethral orifice [Elbadawi, 1996]. The mucous membrane of the bladder is lined by transitional epithelium, which is supported by an underlying loose submucous coat. A three-layer muscular coat of smooth muscle fibers forms the bulk of the bladder wall. There are inner longitudinal, middle circular, and outer longitudinal layers of muscle fibers (i.e., detrusor muscle). The inner longitudinal layer is the thinnest. The middle circular layer, strongest of the three layers, forms a ring ventrally around the internal urethral orifice. The outer longitudinal layer ventrally forms a collarlike structure called the collare vesicae; around the bladder neck, this structure is called the nodus vesicae [Dorschner et al., 1994]. The detrusor muscle is innervated bilaterally and functions as a syncytium [Zimmern et al., 1996]. Gap-type junctions (electrical synapses) occur frequently; axoaxonal-type synapses are also present. Stretch receptors are arranged in series with the detrusor muscle fibers [Bradley, 1986].
The urinary bladder originates from the enlarged terminal portion of the hindgut (the cloaca), which forms during the early somite stage. Ventrally, the entodermal cloacal lining is in direct contact with the surface ectoderm, forming the cloacal membrane. During the fourth to seventh weeks of gestation, the cloaca subdivides into an anterior primitive urogenital sinus and a posterior anorectal canal. This subdivision is accomplished by the caudad growth of the urorectal septum. The entrance of the mesonephric ducts into this primitive urogenital sinus divides the sinus into two portions – the vesicourethral canal above and the definitive urogenital sinus below. The urinary bladder and the upper portion of the urethra are formed from the vesicourethral canal. Therefore, the epithelial lining of the bladder is derived from the embryonic entoderm. Some controversy exists regarding the origin of the trigone of the bladder. A recent study revealed that the trigone is formed primarily from the bladder smooth muscle, with a lesser contribution from ureteral longitudinal fibers [Viana et al., 2007]. The detrusor muscle arises from the visceral mesoderm.
Nerve Supply
The pathways that provide cerebral control of the detrusor neurons have been delineated with horseradish peroxidase and immunohistochemical techniques. In the rat, the urinary bladder appears to be innervated by axons from the caudal portion of the nucleus laterodorsalis tegmenti, which connects with the medial frontal cortex [Sakanaka et al., 1983]. The projections in the rat appear to be ipsilateral and also extend to the ipsilateral septal area; the latter area is involved with emotional and motivational activities [Bradley, 1986]. In humans, the cerebrocortical areas concerned with pudendal and detrusor activation are located in the medial aspect of the area immediately anterior to the rolandic fissure [Haldeman et al., 1982a, b].
Bradley [1986] has divided the various neural interconnections into loops (paths). There are four major paths. Path 1, along with path 4a, provides for cortical control or modification of the detrusor and pudendal pathways. Path 1 is a bidirectional pathway between brainstem and cerebral cortex that includes connections to subcortical nuclei (e.g., thalamus, basal ganglia, and amygdaloid nucleus) (Figure 99-1).
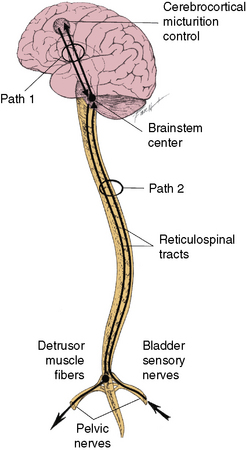
Fig. 99-1 Paths 1 and 2.
(Redrawn from Bradley WE. Physiology of the urinary bladder. In: Walsh PC et al., eds. Campbell’s urology. Philadelphia: WB Saunders, 1986.)
Path 2 provides for brainstem control of the detrusor pathways. It is also bidirectional, traversed by ascending impulses from the detrusor muscle to the brainstem detrusor nucleus, and by descending impulses from the brainstem detrusor nucleus to the sacral spinal cord (see Figure 99-1). Path 3 provides for segmental control of pudendal pathways. Path 3 comprises the following:
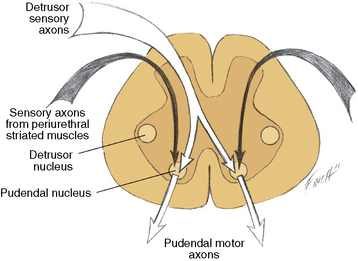
Fig. 99-2 Paths 3 and 4b.
(Redrawn from Bradley WE. Physiology of the urinary bladder. In: Walsh PC et al., eds. Campbell’s urology. Philadelphia: WB Saunders, 1986.)
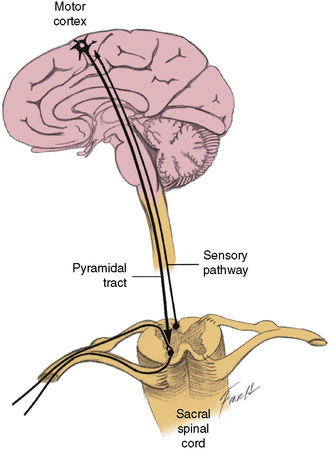
Fig. 99-3 Paths 4a and 4b.
(Redrawn from Bradley WE. Physiology of the urinary bladder. In: Walsh PC et al., eds. Campbell’s urology. Philadelphia: WB Saunders, 1986.)
The efferent portion of path 4b comprises the following:
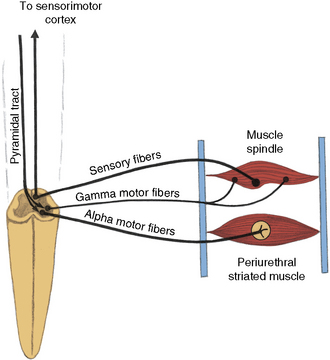
Fig. 99-4 Path 4b.
(Redrawn from Bradley WE. Physiology of the urinary bladder. In: Walsh PC et al., eds. Campbell’s urology. Philadelphia: WB Saunders, 1986.)
Three other minor pathways have been described, which include proximal urethra to detrusor muscle, periurethral striated muscle to detrusor (which inhibits contraction), and sacral afferents to the lumbar cord that cause excitation of lumbar efferents to the pelvic ganglia, which in turn inhibit synaptic transmission in the pelvic ganglia [Bradley, 1986].
The spinal cord detrusor nucleus is located in the region of the parasympathetic nerve cells at S3 in the intermediolateral cell column. S2 and S4 also contribute to the nerve supply (Figure 99-5) [Saper, 1995]. The axons from these cell bodies, after traversing the ventral roots, form the pelvic nerve that innervates the detrusor muscle; both ipsilateral and contralateral innervation occurs.
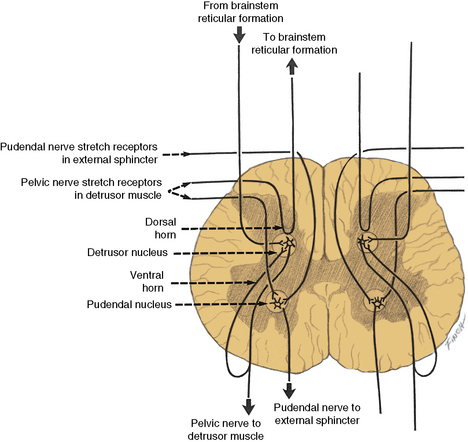
Fig. 99-5 Input–output relationships of conus medullaris.
Recurrent inhibition pathways are observed in the pelvic motor nerves.
(Redrawn from Bradley WE. Physiology of the urinary bladder. In: Walsh PC et al., eds. Campbell’s urology. Philadelphia: WB Saunders, 1986.)
The sympathetic motor nerve supply to the bladder originates in the cell bodies of the intermediolateral column of the spinal cord extending from T11 to L2. The preganglionic nerve fibers from these cell bodies synapse in the sympathetic ganglia of the hypogastric plexus and emerge as postganglionic fibers in the hypogastric nerve. These sympathetic fibers innervate the trigone region of the bladder [Chai and Steers, 1996; Wein and Barrett, 1992].
The cerebrospinopudendal pathway subserves volitional control of micturition. The pudendal nerve arises from the pudendal nucleus (Onuf’s nucleus), which is located in the anterior horn cell column of S3 and S4 (see Figure 99-5). The nerve innervates the external urethral sphincter, as well as the accessory urethral and perineal muscles. The pudendal nucleus is innervated through the pyramidal tract from the upper motor neuron cells situated on the medial surface of the frontal lobe anterior to the rolandic fissure (the paracentral lobule). Somatic afferent impulses, which are transmitted in the pudendal nerve, enter the dorsal roots of S2 to S4. Griffiths and Tadic [2008] have postulated a model of supraspinal bladder control utilizing functional magnetic resonance imaging (fMRI) in adults. During the period of urine storage, afferent signals from the bladder and urethral spincter synapse at the periaqueductal gray (PAG). These are mapped with fMRI to the insula, which is involved in the sensation of bladder filling and the desire to void. The anterior cingulate gyrus is involved in monitoring autonomic function [Critchley et al., 2003], and may send inhibitory impulses to the pontine micturition center via the PAG. The prefrontal cortex is involved in the decision to initiate voiding voluntarily.
Pathophysiology
Most children with lower urinary tract dysfunction suffer from neurologic compromise [Fidas et al., 1987; Sensirivatana et al., 1987]. The micturition reflex is primarily a brainstem reflex and requires intact brainstem reticular formation pathways to the spinal cord (reticulospinal tracts), although other portions of the brain exert significant influence on the micturition reflex. Important areas of brain control of micturition include the frontal sensorimotor cortex (medial surface anterior to the rolandic fissure), the midbrain, and the dorsal tegmental area of the pons (brainstem detrusor motor nucleus); they exert either a facilitatory or an inhibitory effect on the micturition reflex [Carpenter and Sutin, 1983; Chai and Steers, 1996; Wein and Barrett, 1992]. The precise role of the thalamus, hypothalamus, limbic system, and basal ganglia remains largely unknown, although the general contributions of these regions have been delineated partially. The activities in the cerebral cortex, basal ganglia, anterior hypothalamus, and anterior midbrain inhibit the reflex detrusor contraction that is induced by bladder filling. Although connections between the cerebellum and urinary bladder have been established and possible cerebellar influence on micturition has been hypothesized [Bradley, 1986], little specific information is available concerning this relationship.
The brainstem detrusor motor nucleus is located in the caudal portion of the dorsal tegmental area of the pons, rostral to the nucleus locus ceruleus [Tohyama et al., 1978]. Two projections emanate from this nucleus – one to the lateral hypothalamic area and one to the sacral spinal cord [Saper, 1995]. Bilateral lesions involving the pontine tegmentum, resulting in an inability to empty the bladder and urinary retention, have been described [Manente et al., 1996].
The stretch receptors in the detrusor muscle are activated by bladder filling and initiate the micturition reflex [Bradley, 1986]. Some of these stretch receptor-initiated impulses travel in the pelvic nerve afferents, which synapse with the pudendal neurons in the sacral cord (path 3; see Figure 99-2). Impulses inhibit the pudendal neurons, with resultant relaxation of the striated muscles of the internal and external urethral sphincters. Other afferents are directed over a long pathway to the brainstem pudendal nucleus; from there, they travel down the reticulospinal tract to terminate on the sacral detrusor neurons, with resultant muscle contraction (path 2).
The voluntary control of the urethral sphincter is mediated through path 4a, which originates in the neurons of the corticospinal tract (pyramidal tract) in the medial anterior frontal cortex. The axons of the pyramidal tract terminate on the pudendal neurons in the sacral cord and, by effecting activity in path 4b, exert voluntary control over the sphincter muscle (cerebrospinopudendal pathway) [Nakagawa, 1980]. Tonic activity in the pudendal nerves innervating the urethral sphincter maintains closure of the sphincter during both waking and sleeping states. The muscle stretch receptors in the sphincter-striated muscle modulate and maintain this tonic activity. Commanding the patient to contract the sphincter provides a test for evaluating these neural connections. As the innervation of the urethral and anal sphincter is the same for purposes of analysis, anal sphincter monitoring is useful for assessing urethral sphincter function. An anourethral reflex also has been described [Shafik, 1992]. Urethral sphincter function also may be assessed electromyographically by examination of the pelvic floor muscles [Siroky, 1996].
Bilateral pathologic involvement of the pyramidal tract, usually in the spinal cord, results in impaired volitional control of the urethral sphincter; therefore, the urethral sphincter may undergo inappropriate relaxation, or increased sphincter activity may occur during detrusor contraction. This imbalance is termed detrusor-urethral sphincter dyssynergia [Hinman, 1980].
Anatomic and functional classifications of the disorders of micturition, including incontinence, are provided in Box 99-1 and Box 99-2.
Patient Evaluation
History
In spinal cord lesions, the level of injury determines the symptom complex. In lesions of the spinal cord at or below T10, the sensation of suprapubic fullness generally is preserved [Bauer, 1992]. If the lesion is rostral to T6, autonomic overactivity may be present and manifested by bradycardia, chills and diaphoresis, piloerection, paroxysmal hypertension, headache, and suffusion of the head and neck. This overactive autonomic response is the result of exaggerated reflex sympathetic activity in the isolated spinal cord. Plasma catecholamine concentrations are elevated, and peripheral sympathetic activity is increased.
Nocturnal enuresis is defined as persistent night-time bedwetting beyond 5 years of age because 85 percent of children are urinary bladder-continent by this age. Nocturnal enuresis has an uneven age and sex distribution; more boys than girls have this complaint at corresponding ages [Mattsson, 1994; Rushton, 1995]. Sleep polygraphic studies suggest immaturity of the central nervous system (CNS) inhibition of micturition reflex in sleep in enuretic children [Robert et al., 1993]. In a child who never achieved complete bladder control, the enuresis is said to be primary. Secondary enuresis is bedwetting after a dry period, indicating successful urinary bladder control. A few children, more females than males, exhibit daytime giggle micturition (enuresis risoria), which should be distinguished from stress incontinence [Elzinga-Plomp et al., 1995]. Diurnal or nocturnal enuresis occurring beyond the expected age of urinary control requires systematic evaluation. In enuretic children, any associated impairment of sensory or motor function, particularly of the legs and perianal areas, should be carefully delineated because of the possibility of cord involvement.
When present, incontinence should be categorized. Frequency of urination, urgency with a need to rush to the bathroom, and urge incontinence (often accompanied by a squatting posture in girls) usually indicate an overactive bladder. The likely mechanism in this instance is uninhibited detrusor contractions during bladder filling. Infrequent voiding may be due to a hypocontractile and distended bladder. Weak urinary stream, difficulty in initiating urination, or necessity to strain during micturition should lead to a suspicion of overactive sphincter, dysfunctional voiding, and structural obstruction. Urge syndrome is the most common voiding dysfunction in children [Saedi and Schulman, 2003]. Overflow incontinence, relatively rare in childhood, may accompany involvement of central or peripheral motor mechanisms. Loss of urine when lifting objects, coughing, or walking indicates stress incontinence, which may result from a peripheral motor lesion compromising either the detrusor muscle or the muscles of the pelvic floor. Abrupt, uncontrolled voiding is associated with neurologic lesions, such as those involving the cerebral hemispheres or the suprasegmental areas of the spinal cord.
Neurologic Examination
Uninhibited neurogenic bladder may occur with bilateral involvement of the medial frontal lobe areas (e.g., superior sagittal sinus thrombosis). Frequency and urgency of urination are increased; bladder sensation is preserved. As the bladder fills, the detrusor contracts and increases intravesical pressure. The bladder may become so contracted that a minute quantity of urine induces micturition. Ultimately, automatic micturition develops [Saper, 1995]. Suprasegmental lesions of the spinal cord above the lumbar segments are accompanied by urgency, incontinence, increased frequency, and nocturia.
The neurologic examination should be directed at excluding focal or progressive spinal cord lesions. The cutaneous area over the spine should be scrutinized for midline abnormalities that may indicate underlying congenital malformations. The spine should be evaluated for tenderness and scoliosis. Skeletal muscle spasticity, hyperreflexia, clonus, and extensor toe signs accompany suprasegmental lesions. Muscle atrophy, muscle weakness, and decreased or absent deep-tendon reflexes accompany lower motor neuron unit lesions originating in the lumbosacral cord; associated sensory changes are often evident. Syndromes of the epiconus, conus medullaris, and cauda equina are distinguishable by clinical findings and are summarized in Table 99-1 [Wright, 1982].
When the epiconus (L4, L5, S1, and S2) is involved, profound motor involvement of the lower legs and feet occurs [Saper, 1995]. External rotation and dorsiflexion of the thigh are most affected. Abduction at the hip, flexion at the knee, and flexion and extension at the ankle are also involved. The Achilles tendon reflex is absent.
When the conus medullaris (S3 to Coc1) is involved (conus syndrome), paralytic bladder incontinence and, usually, bladder distention occur [Bauer, 1992; Saper, 1995]. Bowel incontinence is also present. There is loss of perianal sensation consonant with impairment of the lower sacral (S3 and S4) and coccygeal cord segments. Rectal sphincter reflex (anal wink) is absent, and denervation of the voluntary anal sphincter causes loss of anal sphincter tone. No motor abnormalities of the legs and feet occur. The Achilles tendon reflex is uninvolved. In a study of traumatic conus lesions, the prognosis was somewhat better than has been appreciated widely [Taylor and Coolican, 1988]. In the cauda equina syndrome, there is involvement of nerve roots from spinal segments L3 to Coc1. It may be difficult to distinguish cauda equina from conus lesions. All forms of sensibility are involved. Spontaneous pain, especially in the perineum over the sacrum and bladder, may dominate the clinical pattern [Saper, 1995]. Peroneal weakness, including footdrop, may occur. Fibrillary twitching in affected muscles is common. When preferential interruption of motor innervation occurs, detrusor contraction is compromised greatly, although bladder sensation is preserved (motor paralytic bladder).
Clinical Laboratory Tests
Studies are chosen to clarify the nature of the bladder dysfunction [Borzyskowski, 1996]. Urinalysis and urine culture, blood urea nitrogen, and serum creatinine are necessary studies. Results of these tests may indicate primary renal or other non-neurologic conditions. Ultrasonography of the kidneys and bladder has been of great value in rapidly assessing structural changes. In one series of children studied with ultrasonography, 32 percent of 74 children with voiding problems had one or more of the following: thickened detrusor muscle; large bladder capacity, with or without residual urine; fecal impaction; suspected bladder neck obstruction, which later required internal urethrotomy; or small bladder capacity [Maizels et al., 1987]. A wide bladder neck anomaly has been recognized as a common cause of incontinence in children [Murray et al., 1987]. Abnormal cystic masses, bladder diverticula, and other structural abnormalities may be recognized; however, interpretation occasionally may be difficult and lead to erroneous diagnoses [Vick et al., 1983].
Urodynamic study of the free-flow curve provides valuable data without invasive instrumentation or an extensive urodynamic study. If the free-flow curve is normal and there is no residual urine, a significant voiding abnormality, whether functional or anatomic in origin, is unlikely [Griffiths and Scholtmeijer, 1984]. Study methods particularly adapted to children and norms for children have been developed [Di Scipio et al., 1986; Pompino and Hoffmann, 1983]. Video urodynamic studies of children with grossly intact neurologic function often reveal detrusor dysfunction and abnormal sphincter activity [Webster et al., 1984]. Computer-monitored voiding studies can provide useful information concerning detrusor muscle function and allow categorization of the dysfunction [van Mastrigt and Griffiths, 1986]. Urodynamic evaluation and follow-up care are important in the diagnosis and optimal management of many children with micturition problems [Churchill et al., 1987].
Plain radiographs of the lumbosacral area, intravenous pyelography, and a voiding cystourethrogram all provide further information. Bladder prolapse, with the bladder neck situated below the upper margin of the pubic symphysis, has been described as a urographic radiologic sign of urethral sphincter denervation in children with myelodysplasia [Zerin et al., 1990]. MRI of the lumbosacral cord should be performed if there is clinical suspicion for tethered cord or other pathology involving the epiconus, conus medularis, or cauda equina.
Conventional invasive urologic examinations, now less commonly required because of modern imaging techniques, should be performed when other examinations have not provided sufficient data [Ewalt and Bauer, 1996; Walther and Kaplan, 1979].
Detrusor muscle function can be evaluated by cystometry if other methods are insufficient. A slow-fill cystometrogram triggers bladder contraction after dilatation with instilled fluid. Detrusor tone, reflex threshold, sensation, and volitional ability to inhibit detrusor contraction can be assessed. Reflex abnormalities of the detrusor (i.e., hyperreflexia, hyporeflexia, or areflexia) may be documented [Bauer, 1992; Rushton, 1995]. Voiding cystourethrography and other radiologic techniques provide useful additional information [Bisset et al., 1987; Lebowitz, 1985].
Although infrequently performed, percutaneous stimulation of the sacral roots can be carried out, in conjunction with transducer placement in the bladder, to monitor peripheral reflex pathways. Intact preganglionic pathways to the bladder are necessary for optimal detrusor response after percutaneous stimulation. Currently, sacral-evoked responses are used to evaluate these pathways, typically by determining the latency of the bulbocavernosus reflex [Vodusek, 1996; Wein and Barrett, 1992].
Testing of the postganglionic pathways is more complex. The effect of percutaneous stimulation is assessed, as well as the detrusor contraction in response to direct electrical stimulation applied through a cystoscope, and the intravesical pressure response to bethanechol chloride [Chai and Steers, 1996; Ewalt and Bauer, 1996; Wein and Barrett, 1992]. Muted or absent response to percutaneous and direct electrical stimulation, and denervation hypersensitivity to bethanechol suggest impaired postganglionic pathways. Lack of a contractile response to direct stimulation and lack of denervation hypersensitivity may indicate myopathic detrusor changes, which result from bladder overdistention or infection.
Differential Diagnosis
Nocturnal enuresis is the most common disorder of micturition in childhood. Neurologic disease must be excluded in any child who either does not develop or subsequently loses urinary continence. Box 99-3 presents a list of differential diagnoses in disorders of micturition. Several dysfunctional voiding syndromes without associated neurologic dysfunction (non-neurogenic voiding disorders) have been described and respond to a variety of therapeutic interventions [Homsy, 1994; Jayanthi et al., 1997; Yang and Mayo, 1997]. Chronic constipation may sometimes be associated with urinary incontinence in children [Loening-Baucke, 1997].
Management
Individuals with impaired bladder and bowel control report a low self-concept and low self-esteem, with lower perceived quality of life on several domains, and have greater difficulty in creating new social relationships [Hicken et al., 2001; Moore et al., 2004]. Precise diagnosis is required for a systematic and effective therapeutic approach. Neurogenic bladder dysfunction must be countermanded so that urinary control and normal renal function are allowed to continue [Chandra, 1995]. The goals of treatment are to preserve renal function, prevent urinary infection, and promote urinary continence in older children [Fernandes et al., 1994]. Optimal neurogenic therapy prevents infections (Table 99-2). Patients require regular monitoring. Medications commonly employed in treating bladder dysfunction are listed in Table 99-2.
Hyperreflexic bladder, the result of impairment of suprasegmental governance of the detrusor muscle, is associated with increased resting bladder tone, uncontrollable bladder contraction with incontinence, frequency and urgency of urination, and diminished bladder volume. Therapeutic measures are directed at these cardinal features [Chua et al., 1996; Sakakibara et al., 1996; Wyndaele, 1995].
Administration of anticholinergic drugs decreases contractility. In a randomized controlled trial of oxybutynin versus tolterodine for detrusor instability, percentage reduction of urge incontinence was similar between the two medications, while tolterodine was better tolerated [Kilic et al., 2006]. Common side effects of anticholinergic medications included dry mouth, decreased sweating, hyperpyrexia, and constipation. Potential central adverse effects of anticholinergic medications include sedation, confusion, delerium, agitation, and hallucinations [Gish et al., 2009]. Unfortunately, anticholinergic therapy may also be associated with urinary retention and an increase in residual urine; therefore, residual urine volume should be estimated and urinary output monitored. Intermittent catheterization may prove necessary if retention occurs [Cass et al., 1984]. The Credé or Valsalva maneuver sometimes may be sufficient to empty the bladder completely [Bauer, 1992]. Renal rupture after the Credé maneuver has been described [Reinberg et al., 1994]. Drugs that augment the noradrenergic system appear to facilitate continence; in addition, drugs that depress or block dopamine activity may produce urinary incontinence. Dopamine agonists and specific catecholamine agents may prove valuable in managing urinary incontinence [Ambrosini, 1984].
Neurogenic bladder outlet obstruction may respond to α-adrenergic blocking agents. Alpha-blocker therapy with doxazosin also has been used successfully to reduce postvoid residual urine volume in children with complex neuropathic and non-neuropathic voiding dysfunction [Cain et al., 2003]. The phentolamine test can be used to predict which patients are likely to benefit from phenoxybenzamine therapy [Smey et al., 1980]. Orthostatic hypotension, dizziness, and headaches are potential side effects of α-adrenergic blockers. Use of oral baclofen may be of limited benefit in a few patients. It probably relaxes the external sphincter and may be used as an adjunct. Intravesical medications may be used in children who either cannot tolerate or have a poor and inadequate response to oral therapy [Greenfield and Fera, 1991; Kato et al., 1991]. Intrathecal baclofen has also been used [Frost et al., 1989]. Botulinum A toxin injection into the external urethral sphincter has been used for the treatment of detrusor-sphincter dyssynergia in patients with spinal cord injury [Schurch et al., 1997]. Functional bladder-sphincter dyssynergia may be successfully treated by using electromyographic biofeedback with exercises, which consist of alternate tensing and relaxing of lower pelvic muscles [Libo et al., 1983]. Other biofeedback training programs also have been effective in managing children with probable non-neurogenic bladder disturbances [Hellström et al., 1987; Jerkins et al., 1987].
Mechanical compression (Credé or Valsalva’maneuver), intermittent self-catheterization [Bauer, 1992; Cass et al., 1984; Chai et al., 1995], and various surgical techniques may be of value. Clean, intermittent catheterization has been used widely in children. Early implementation of this method helps preserve renal function, especially in children with myelodysplasia [Kochakarn et al., 2004]. It may be used in combination with anticholinergic drugs in infants [Baskin et al., 1990; Joseph et al., 1989; Lapides et al., 1972]. Surgical techniques include transurethral incision of the external urethral sphincter, urinary stream diversion operations, implantation of an artificial urinary sphincter [Khoury and Churchill, 1987; Light, 1985; Simeoni et al., 1996], bladder augmentation [Krishna et al., 1995], bladder replacement [Hensle and Burbige, 1985; King et al., 1987], free autogenous muscle transplantation [Hakelius et al., 1984], and differential sacral nerve root rhizotomy and electrical stimulation [Sindou et al., 1994; Tanagho et al., 1989]. Nonsurgical therapies should be exhausted before surgical therapy is undertaken. Early intervention may sometimes be necessary to preserve normal function or avoid progressive dysfunction, especially in children with spinal dysraphism [Bauer, 1992; Keating et al., 1988].
Several incontinence products are available for use in children with urinary incontinence [Sanders, 2002].
Disorders of Defecation
Defecation is the conscious act of emptying the bowel, and continence is the ability to control defecation voluntarily. Fecal soiling is the presence of stool in the underwear, independent of whether an organic or anatomic lesion is present. Fecal incontinence is fecal soiling when there is an organic or anatomic lesion, such as meningomyelocele or other neuromuscular disorders, anal malformations, anal surgery, or traumatic lesions [Loening-Baucke, 1996]. Reflex neuromuscular mechanisms and the anatomy of the rectum, anus, and sphincters contribute to anal continence in a normal child. Bowel control usually precedes bladder control in children [Perlmutter, 1985]. Conscious bowel control is achieved at 28 months of age on average [Brazelton, 1962]. Toilet training is a complex multistep process, as mentioned in the preceding discussion.
Anatomy and Embryology
The course of the rectum is aligned at an approximate 90-degree angle to the axis of the anal canal. The sharp angular bend favors anal continence. The pelvic floor is formed from the levator ani muscles, which elevate the anorectal junction and thrust it anteriorly during contraction. The resultant straightening of the path between the anal canal and rectum facilitates defecation. Fecal continence is the result of the interrelationships among the two anal sphincters and the levator ani muscles [Rao, 1996, 1997].
Nerve Supply
In a simplistic sense, the sympathetic nervous system modulates rectal filling, and the parasympathetic system controls bowel emptying. Activation of the parasympathetic system results in contraction of the sigmoid and rectal smooth muscles, with concomitant relaxation of the internal sphincter. Activation of the sympathetic system causes the reverse pattern – relaxation of the sigmoid and rectum, and contractions of the internal sphincter [Rao, 1996, 1997].
Interaction of tone and reflex activity is essential in the physiology of continence. Reflex and voluntary contractions of the puborectalis muscle and internal and external sphincters are important components of continence during sleep and waking. Due to the intricate relationship of the sphincters, determining their contribution to maintenance of resting pressure is difficult; however, the resting pressure appears primarily to be the result of contraction of the internal sphincter [Pemberton and Kelly, 1986].
Incontinence occurs when the pressure induced by the peristaltic movement in the distal rectum overcomes the anorectal pressure. There are three types of incontinence – true incontinence, partial incontinence, and overflow incontinence [Wright, 1982]. Deficient anorectal muscles (e.g., imperforate anus) or impaired neuromuscular function result in true incontinence. There may be insufficient anorectal pressure or impaired sensation of peristaltic movement and bowel distention. Fecal soiling is the result of partial incontinence because the final stage of defecation is inadequate and is followed by continuing passage of small amounts of stool. Overflow incontinence, not a true form of incontinence, results from chronic fecal impaction and leakage of liquid feces through the dilated anorectal ring.
Patient Evaluation
Neurologic Examination
In lesions above the sacral cord (suprasegmental lesions), voluntary control over the sphincter ani is lost. Sphincter tone is increased and fecal retention occurs, leading to chronic fecal impaction and consequent soiling. During rectal examination, the patient should be able to contract and relax the sphincter on request. Sphincter tone is often increased and contracted with suprasegmental lesions [Wright, 1982]. Paralysis of the sphincter ani accompanies sacral cord disturbance. The anus is patulous, the anal sphincter reflex is lacking, and the patient is unable to contract the sphincter on command. In contrast with bladder function, the extent of preservation or return of anal and rectal sensation in conus medullaris lesions is important in achieving bowel control. The involuntary passage of flatus is a distressing symptom in such patients if they cannot distinguish it from feces, for which sensory input is essential [Taylor and Coolican, 1988]. Further information on the examination of the patient with lower cord and cauda equina lesions can be found in the section on urinary incontinence.
Clinical Laboratory Tests
Proctoscopy and fiberoptic studies of the lower bowel are efficient methods of excluding the presence of structural abnormalities. Barium enema studies sometimes are necessary, as are defecography studies, which monitor the functional events visually during defecation [Mezwa et al., 1993].
Special studies include anal sphincter electromyography [Lewis and Rudolph, 1997; Rao, 1996, 1997; Shafik, 1992]. Denervation potentials indicate a recent lower motor neuron process. Pudendal nerve terminal motor latency is especially useful when neuropathy is suspected. Overall electrodiagnostic studies are helpful in the evaluation of fecal incontinence, although less so in very young children [Cheong et al., 1995]. A manometric study may also be useful [Rao, 1996]. The correlation between the clinical findings and the manometric assessment of sphincters in response to rectal distention often has been thought to be poor; recent re-evaluation suggests otherwise [Rao and Patel, 1997]. Computed tomography allows imaging of the puborectal muscle and external sphincters, and identification of associated anomalies [Ikawa et al., 1985; Kohda et al., 1985]. Sacral ratios less than 0.52 correlate well with spinal cord anomalies and with unfavorable prognosis in children with anorectal malformations. Sacral ratio is determined by dividing the distance from the lowest point of the sacrum (or of the coccyx, if present) to the line joining the two posterior iliac spines by the distance from that latter line to the line joining the upper limits of the iliac crests [Torre et al., 2001].
Differential Diagnosis
Bowel dysfunction is produced by the conditions listed in Box 99-3. Lumbosacral defects may be associated with reduced or absent external sphincter function, which is demonstrated by sphincter electromyography. Denervation potentials or reduced large-amplitude potentials may be observed. In Hirschsprung’s disease, manometric studies demonstrate an absence of the rectoanal inhibitory reflex; that is, the internal sphincter fails to relax in response to a transient rectal distention. The relaxation wave is absent.
The most common cause of bowel incontinence is encopresis, which is not associated with neurologic dysfunction. Encopresis is fecal incontinence without organic cause for more than a month in children 4 years of age or older. Males outnumber females by 3.5 to 1 [Bellman, 1966]. The cardinal feature of encopresis is withholding of stools: This condition often is not recognized by parents. Neurogenic and other organic conditions causing bowel dysfunction must be excluded [Loening-Baucke, 1996; Nolan and Oberklaid, 1993].
Management
Assessment of contributing factors, including psychosocial milieu, anorectal examination, and appropriate neurologic evaluation, is necessary for adequate management. Therapeutic approaches include dietary, behavioral, pharmacologic, and surgical methods [Wald, 1986]. Education is important in achieving good outcome [King et al., 1994].
Dietary measures to harden stools may be attempted for some patients. The stools can then be evacuated by manual pressure over the abdomen and by straining of the abdominal muscles. Some children may perceive the urge to defecate when the stools are bulkier, which can be achieved by using stool-bulking agents. High-fiber diet may be appropriate in some cases. Some of the commercially available fiber supplements include Metamucil, Benefiber, Citrucel, FiberCon, and FiberChoice. Rectal suppositories (bisacodyl) or a saline enema may empty the rectum before it fills and cause reflex relaxation of the internal sphincter. This approach may prevent soiling in some children. Excessive flatus and soiling also may be controlled by avoiding certain foods, such as legumes, and by paying attention to strict dietary and bowel habits. Antegrade colonic enemas have been used in children who have failed usual medical treatment [Griffiths and Malone, 1995; Squire et al., 1993].
Biofeedback training [Owen-Smith and Chesterfield, 1986], behavior modification [Whitehead et al., 1986], muscle training, and medications often are beneficial [Scharli, 1987]. New devices to control both fecal and urinary incontinence have shown promise [Numanoglu, 1987]. Surgical intervention may be considered if conservative treatment fails [Bass and Yazbeck, 1987; Chen and Zhang, 1987; Kottmeier et al., 1986; Peña, 1994]. Artificial anal sphincter, gracilis muscle transposition, and dynamic graciloplasty have also been used with good outcome in children who fail medical management [Baeten et al., 1995; Han et al., 1995; Wong et al., 1996].
References
The complete list of references for this chapter is available online at www.expertconsult.com.
Ambrosini P.J. A pharmacological paradigm for urinary incontinence and enuresis. J Clin Psychopharmacol. 1984;4:247.
Baeten C.G.M.I., Geerdes B.P., Adang E.M.M., et al. Anal dynamic graciloplasty in the treatment of intractable fecal incontinence. N Engl J Med. 1995;332:1600.
Baskin L.S., Kogan B.A., Benard F. Treatment of infants with neurogenic bladder dysfunction using anticholinergic drugs and intermittent catheterization. Br J Urol. 1990;66:532.
Bass J., Yazbeck S. Reoperation by anterior perineal approach for missed puborectalis. J Pediatr Surg. 1987;22:761.
Bauer S.B. Neuropathology of the lower urinary tract. In Kelalis P.P., King L.R., Belman A.B., editors: Clinical pediatric urology, ed 3, Philadelphia: WB Saunders, 1992.
Bellman M. Studies in encopresis. Acta Paediatr Scand. 1966;170(Suppl):1.
Bisset G.S.III, Strife J.L., Dunbar J.S. Urography and voiding cystourethrography. Findings in girls with urinary tract infection. Am J Roentgenol. 1987;148:479.
Borzyskowski M. An update on the investigation of the child with a neuropathic bladder. Dev Med Child Neurol. 1996;38:744.
Bradley W.E. Physiology of the urinary bladder. In: Walsh P.C., Gittes B.F., Perlmutter A.D., et al, editors. Campbell’s urology. Philadelphia: WB Saunders, 1986.
Brazelton T.B. A child oriented approach to toilet training. Pediatrics. 1962;29:121.
Cain M.P., Wu S.D., Austin P.F., et al. Alpha blocker therapy for children with dysfunctional voiding and urinary retention. J Urol. 2003;170:1514.
Carpenter M.B., Sutin J. Human neuroanatomy. Baltimore: Williams & Wilkins; 1983.
Cass A.S., Luxenberg M., Gleich P., et al. Clean intermittent catheterization in the management of the neurogenic bladder in children. J Urol. 1984;132:526.
Chai T., Chung A.K., Belville W.D., et al. Compliance and complications of clean intermittent catheterization in the spinal cord injured patient. Paraplegia. 1995;33:161.
Chai T.C., Steers W.D. Neurophysiology of micturition and continence. Urol Clin North Am. 1996;23:221.
Chandra M. Reflux nephropathy, urinary tract infection, and voiding disorders. Curr Opin Pediatr. 1995;7:164.
Chen Y.L., Zhang X.H. Reconstruction of rectal sphincter by transposition of gluteus muscle for fecal incontinence. J Pediatr Surg. 1987;22:62.
Cheong D.M.O., Vaccaro C.A., Salanga V.D., et al. Electrodiagnostic evaluation of fecal incontinence. Muscle Nerve. 1995;18:612.
Chua H.C., Tow A., Tan E.S. The neurogenic bladder in spinal cord injury: Pattern and management. Ann Acad Med Singapore. 1996;25:553.
Churchill B.M., Gilmour R.F., Williot P. Urodynamics. Pediatr Clin North Am. 1987;34:1133.
Critchley H.D., Mathias C.J., Josephs O., et al. Human cingulate cortex and autonomic control: converging neuroimaging and clinical evidence. Brain. 2003;126:2139.
Di Scipio W.J., Smey P., Kogan S.J., et al. Impromptu micturitional flow parameters in normal boys. J Urol. 1986;136:1049.
Dorschner W., Stolzenburg J.U., Leutert G. A new theory of micturition and urinary continence based on histomorphological studies. Urol Int. 1994;52:61.
Elbadawi A. Functional anatomy of the organs of micturition. Urol Clin North Am. 1996;23:177.
Elzinga-Plomp A., Boemers T.M.L., Messer A.P., et al. Treatment of enuresis risoria in children by self-administered electric and imaginary shock. Br J Urol. 1995;76:775.
Ewalt D.H., Bauer S.B. Pediatric neurourology. Urol Clin North Am. 1996;23:501.
Fernandes E.T., Reinberg Y., Vernier R., et al. Neurogenic bladder dysfunction in children: Review of pathophysiology and current management. J Pediatr. 1994;124:1.
Fidas A., Elton R.A., McInnes A., et al. Neurophysiological measurement of the voiding reflex arcs in patients with functional disorders of the lower urinary tract. Br J Urol. 1987;60:205.
Frost F., Nanninga J., Penn R., et al. Intrathecal baclofen infusion effect on bladder management programs in patients with myelopathy. Am J Phys Med Rehabil. 1989;68:112.
Gish P., Mosholder A.D., Truffa M., et al. Spectrum of central anticholinergic adverse effects associated with oxybutynin: comparison of pediatric and adult cases. J Pediatr. 2009;155(3):432.
Greenfield S.P., Fera M. The use of intravesical oxybutynin chloride in children with neurogenic bladder. J Urol. 1991;146:532.
Griffiths D.J., Scholtmeijer R.J. Place of the free flow curve in the urodynamic investigation of children. Br J Urol. 1984;56:474.
Griffiths D., Tadic S.D. Bladder control, urgency, and urge incontinence: evidence from functional brain imaging. Neurourol Urodyn. 2008;27:446.
Griffiths D.M., Malone P.S. The Malone antegrade continence enema. J Pediatr Surg. 1995;30:68.
Hakelius L., Gierup J., Grotte G. Urinary incontinence in children. Treatment with free autogenous muscle transplantation. Prog Pediatr Surg. 1984;17:155.
Haldeman S., Bradley W.E., Bhatia N.N., et al. Pudendal somatosensory evoked potentials. Arch Neurol. 1982;39:280.
Haldeman S., Bradley W.E., Bhatia N.N., et al. Evoked responses from pudendal nerve. J Urol. 1982;128:974.
Han S.J., Park H.J., Kmi C.B., et al. Long-term follow-up of gracilis muscle transposition in children. Yonsei Med J. 1995;36:372.
Hellström A., Hanson E., Hansson S., et al. Micturition habits and incontinence at age 17 reinvestigation of a cohort studies at age 7. Br J Urol. 1995;76:231.
Hellström A.L., Hjalmask K., Jodal U. Rehabilitation of the dysfunctional bladder in children: method and 3-year follow up. J Urol. 1987;138:847.
Hensle T.W., Burbige K.A. Bladder replacement in children and young adults. J Urol. 1985;133:1004.
Herndon C.D.A., Joseph D.B. Urinary incontinence. Pediatr Clin North Am. 2006;53:363.
Hicken B.L., Putzke J.D., Richards J.S. Bladder management and quality of life after spinal cord injury. Am J Phys Med Rehabil. 2001;80:916.
Himsel K.K., Hurwitz R.S. Pediatric urinary incontinence. Urol Clin North Am. 1991;18:283.
Hinman F. Syndromes of vesical incoordination. Urol Clin North Am. 1980;7:311.
Homsy Y.L. Dysfunctional voiding syndromes and vesicoureteral reflux. Pediatr Nephrol. 1994;8:116.
Ikawa H., Yokoyama J., Sanbonmatsu T., et al. The use of computerized tomography to evaluate anorectal anomalies. J Pediatr Surg. 1985;20:640.
Jayanthi V.R., Khoury A.E., McLorie G.A., et al. The nonneurogenic neurogenic bladder of early infancy. J Urol. 1997;158:1281.
Jerkins G.R., Noe H.N., Vaughn W.R., et al. Biofeedback training for children with bladder sphincter incoordination. J Urol. 1987;138:1113.
Joseph D.B., Bauer S.B., Colodny A.H., et al. Clean, intermittent catheterization of infants with neurogenic bladder. Pediatrics. 1989;84:78.
Kato K., Kondo A., Saito M., et al. In vitro intravesical instillation of anticholinergic, antispasmodic and calcium blocking agents to decrease bladder contractility. Urol Int. 1991;47(Suppl 1):36.
Keating M.A., Rink R.C., Bauer S.B., et al. Neurological implications of the changing approach in management of occult spinal lesions. J Urol. 1988;140:1299.
Khoury A.E., Churchill B.M. The artificial urinary sphincter. Pediatr Clin North Am. 1987;34:1175.
King J.C., Currie D.M., Wright E. Bowel training in spina bifida: Importance of education, patient compliance, age, and anal reflexes. Arch Phys Med Rehabil. 1994;5:243.
Kilic N., Balkan E., Akgoz S., et al. Comparison of the effectiveness and side-effects of tolterodine and oxybutnin in children with detrusor instability. Int J Urol. 2006;13(2):105.
King L.R., Webster G.D., Bertram R.A. Experiences with bladder reconstruction in children. J Urol. 1987;138:1002.
Kochakarn W., Ratana-Olarn K., Lertsithichai P., et al. Follow-up of long-term treatment with clean intermittent catheterization for neurogenic bladder in children. Asian J Surg. 2004;27:134.
Kohda E., Fujioka M., Ikawa H., et al. Congenital anorectal anomaly. CT evaluation. Radiology. 1985;157:349.
Kottmeier P.K., Velcek F.T., Klotz D.H., et al. Results of levatorplasty for anal incontinence. J Pediatr Surg. 1986;21:647.
Krishna A., Gough D.C.S., Fishwick J., et al. Ileocystoplasty in children: Assessing safety and success. Eur Urol. 1995;27:62.
Lapides J., Diokno A.C., Silber S.J., et al. Clean, intermittent self catheterization in the treatment of urinary tract disease. J Urol. 1972;107:458.
Lebowitz R.L. Pediatric uroradiology. Pediatr Clin North Am. 1985;32:1353.
Lewis G., Rudolph C.D. Practical approach to defecation disorders in children. Pediatr Ann. 1997;26:260.
Libo L.M., Arnold G.E., Woodside J.R., et al. EMG biofeedback for functional bladder-sphincter dyssynergia. A case study. Biofeedback Self Regul. 1983;8:243.
Light J.K. The artificial urinary sphincter in children. Experience with AS800 series and bowel reconstruction. Urol Clin North Am. 1985;12:103.
Loening-Baucke V. Encopresis and soiling. Pediatr Clin North Am. 1996;43:279.
Loening-Baucke V. Urinary incontinence and urinary tract infection and their resolution with treatment of chronic constipation of childhood. Pediatrics. 1997;100:228.
Maizels M., Zaontz M.R., Houlihan D.L., et al. In-office ultrasonography to image the kidneys and bladder of children. J Urol. 1987;138:1031.
Manente G., Melchionda D., Uncini A. Urinary retention in bilateral pontine tumour: Evidence for a pontine micturition center in humans. J Neurol Neurosurg Psychiatry. 1996;61:528.
Mattsson S. Urinary incontinence and nocturia in healthy schoolchildren. Acta Paediatr. 1994;83:950.
Mezwa D.G., Feczko P.J., Bosanko C. Radiologic evaluation of constipation and anorectal disorders. Radiol Clin North Am. 1993;31:1375.
Moore C., Kogan B.A., Parekh A. Impact of urinary incontinence on self concept in children with spina bifida. J Urol. 2004;17:1659.
Murray K., Nurse D., Borzykowski M., et al. The “congenital” wide bladder neck anomaly. A common cause of incontinence in children. Br J Urol. 1987;59:533.
Nakagawa S. Onuf’s nucleus of the sacral cord in a South American monkey (Saimiri): Its location and bilateral cortical input from area 4. Brain Res. 1980;191:337.
Nolan T., Oberklaid F. New concepts in the management of encopresis. Pediatr Rev. 1993;14:447.
Numanoglu I. Anal and urinary control device. Artif Organs. 1987;11:420.
Owen-Smith V.H., Chesterfield B.W. Computerized biofeedback achieving continence in high anal atresia. Arch Dis Child. 1986;1:1033.
Pemberton J.H., Kelly K.A. Achieving enteric continence: Principles and applications. Mayo Clin Proc. 1986;61:586.
Peña A. The posterior sagittal approach: Implication in adult colorectal surgery. Dis Colon Rectum. 1994;37:1.
Perlmutter A.D. Enuresis. In: Kelalis P.P., King L.R., Belman A.B., editors. Clinical pediatric urology. Philadelphia: WB Saunders, 1985.
Pompino H.J., Hoffmann D. Normal urinary flow for girls aged 3-14 years. Z Kinderchir. 1983;38:177.
Rao S.S. Manometric evaluation of constipation. Part I. Gastroenterologist. 1996;4:145.
Rao S.S. Manometric evaluation of defecation disorders: Part II. Fecal incontinence. Gastroenterologist. 1997;5:99.
Rao S.S.C., Patel R.S. How useful are manometric tests of anorectal function in the management of defecation disorders? Am J Gastroenterol. 1997;92:469.
Reinberg Y., Fleming T., Gonzales R. Renal rupture after the Credé maneuver. J Pediatr. 1994;124:279.
Robert M., Averous M., Besset A., et al. Sleep polygraphic studies using cystomanometry in twenty patients with enuresis. Eur Urol. 1993;24:97.
Rushton H.G. Wetting and functional voiding disorders. Urol Clin North Am. 1995;22:75.
Saedi N.A., Schulman S.L. Natural history of voiding dysfunction. Pediatr Nephrol. 2003;18:894.
Sakakibara R., Takamichi H., Yasuda K., et al. Micturition disturbance in acute transverse myelitis. Spinal Cord. 1996;34:481.
Sakanaka M., Shiosaka S., Takatsuki K., et al. Evidence for the existence of substance P containing pathway from the nucleus laterodorsalis tegmenti (castaldi) to the medial frontal cortex of the rat. Brain Res. 1983;259:123.
Sanders C. Choosing incontinence products for children. Nurs Stand. 2002;16:39.
Saper C.B. Bing and Haymaker’s neurological diagnosis. St. Louis: Mosby; 1995.
Scharli A.F. Anorectal incontinence: Diagnosis and treatment. J Pediatr Surg. 1987;22:693.
Schurch B., Hodler J., Rodic B. Botulinum A toxin as a treatment of detrusor-sphincter dyssynergia in patients with spinal cord injury: MRI controlled transperineal injections. J Neurol Neurosurg Psychiatry. 1997;63:474.
Sensirivatana R., Watana D., Sornmani W., et al. Diagnostic study of urinary frequency in children. Urology. 1987;30:50.
Shafik A. Anourethral reflex. Description of a reflex and its clinical significance: Preliminary study. Paraplegia. 1992;30:210.
Simeoni J., Guys J.M., Mollard P., et al. Artificial urinary sphincter implantation for neurogenic bladder: A multi-institutional study in 107 children. Br J Urol. 1996;78:287.
Sindou M., Turano G., Pantieri R., et al. Intraoperative monitoring of spinal cord SEPs during microsurgical DREZotomy (MDT) for pain, spasticity, and hyperactive bladder. Stereotact Funct Neurosurg. 1994;62:164.
Siroky M.B. Electromyography of the perineal floor. Urol Clin North Am. 1996;23:299.
Smey P., King L.R., Firlit C.F. Dysfunctional voiding in children secondary to internal sphincter dyssynergia: Treatment with phenoxybenzamine. Urol Clin North Am. 1980;7:337.
Soderstrom U., Hoelcke M., Alenius L., et al. Urinary and faecal incontinence: A population-based study. Acta Paediatr. 2004;93:386.
Squire R., Kiely E.M., Carr B., et al. The clinical application of the Malone antegrade colonic enema. J Pediatr Surg. 1993;28:1012.
Stadtler A.C., Gorski P.A., Brazelton T.B. Toilet training methods, clinical interventions, and recommendations. American Academy of Pediatrics. Pediatrics. 1999;103:1359.
Tanagho E.A., Schmidt R.A., Orvis B.R. Neural stimulation for control of voiding dysfunction: A preliminary report in 22 patients with serious neuropathic voiding disorders. J Urol. 1989;142:340.
Taylor T.K.F., Coolican M.J.R. Injuries of the conus medullaris. Paraplegia. 1988;26:393.
Tohyama M., Satoh K., Sakaumoto T. Organization and projections of the neurons in the dorsal tegmental area of the rat. J Hirnforsch. 1978;19:165.
Torre M., Martucciello G., Jasonni V. Sacral development in anorectal malformations and in normal population. Pediatr Radiol. 2001;31:858.
Van Mastrigt R., Griffiths D.J. An evaluation of contractility parameters determined from isometric contractions and micturition studies. Urol Res. 1986;14:45.
Viana R., Batourina E., Huang H., et al. The development of the bladder trigone, the center of the anti-reflux mechanism. Development. 2007;134:3763.
Vick C.W., Viscomi G.N., Mannes E., et al. Pitfalls related to the urinary bladder in pelvic sonography: A review. Urol Radiol. 1983;5:253.
Vodusek D.B. Evoked potential testing. Urol Clin North Am. 1996;23:427.
Wald A. Fecal incontinence. Effective nonsurgical treatments. Postgrad Med. 1986;80:123.
Walther P.C., Kaplan G.W. Cystoscopy in children: Indications for its use in common urologic problems. J Urol. 1979;122:717.
Webster G.D., Koefoot R.B.Jr, Sihelnik S. Urodynamic abnormalities in neurologically normal children with micturition dysfunction. J Urol. 1984;132:74.
Wein A.J., Barrett D.M. Physiology of micturition and urodynamics. In Kelalis P.P., King L.R., Belman A.B., editors: Clinical pediatric urology, ed 3, Philadelphia: WB Saunders, 1992.
Whitehead W.E., Parker L., Bosmajian L., et al. Treatment of fecal incontinence in children with spina bifida. Comparison of biofeedback and behavior modification. Arch Phys Med Rehabil. 1986;67:218.
Wong W.D., Jensen L.L., Bartolo D.C.C., et al. Artificial anal sphincter. Dis Colon Rectum. 1996;39:1345.
Wright F.S. Disorders of micturition and defecation. In Swaiman K.F., Wright F.S., editors: The practice of pediatric neurology, ed 2, St. Louis: Mosby, 1982.
Wyndaele J.J. Development and evaluation of the management of the neuropathic bladder. Paraplegia. 1995;33:305.
Yang C.C., Mayo M.E. Morbidity of dysfunctional voiding syndrome. Urology. 1997;49:445.
Yeung C.K., Godley M.L., Ho C.K.W., et al. Some new insights into bladder function in infancy. Br J Urol. 1995;76:235.
Zerin M.J., Lebowitz R.L., Bauer S.B. Descent of the bladder neck:A urographic finding in denervation of the urethral sphincter in children with myelodysplasia. Radiology. 1990;174:833.
Zimmern P.E., Lin V.K., McConnell J.D. Smooth-muscle physiology. Urol Clin North Am. 1996;23:211.