Developmental Disorders
Causes, Mechanisms, and Patterns
Early representations of some malformed infants are remarkable in their anatomical accuracy, and it is often possible to diagnose specific conditions or syndromes from the ancient art (Fig. 8.1A). By the Middle Ages, however, representations of malformations were much more imaginative, with hybrids of humans and other animals often represented (Fig. 8.1B).
Thalidomide is a very effective sedative that was widely used in West Germany, Australia, and other countries during the late 1950s. Soon, physicians began to see infants born with extremely rare birth defects. One example is phocomelia (which means “seal limb”), a condition in which the hands and feet seem to arise almost directly from the shoulder and hip (Fig. 8.2). Another is amelia, in which a limb is entirely missing. Thalidomide was identified as the certain cause after some careful epidemiological detective work involving the collection of individual case reports and sorting of the drugs taken by mothers during the early period of their pregnancies. Thalidomide, which is an inhibitor of tumor necrosis factor-α, is still a drug of choice in the treatment of leprosy and multiple myeloma. With the intense investigations that followed the thalidomide disaster, modern teratology came of age. Despite much effort, however, the causes of most congenital malformations are still unknown.
General Principles
Birth defects present themselves in a variety of forms and associations, ranging from simple abnormalities of a single structure to often grotesque deformities that may affect an entire body region. Some of the common classes of malformations are listed in Table 8.1.
Table 8.1
Abnormalities of Individual Structures | |
Malformation | A structural defect of part of or an entire organ or larger part of a body region that is caused by an abnormal process intrinsic to its development (e.g., coloboma) (see p. 285) |
Disruption | A defect in an organ or body part caused by process that interferes with an originally normal developmental process (e.g., thalidomide-induced phocomelia) (see p. 149) |
Deformation | A structural abnormality caused by mechanical forces (e.g., amniotic band constriction) (see Fig. 8.16) |
Dysplasia | An abnormality of a tissue due to an abnormal intrinsic developmental process (e.g., ectodermal dysplasia) (see p. 150) |
Defects Involving More Than One Structure | |
Sequence | A pattern of multiple malformations stemming from a disturbance of a prior developmental process or mechanical factor (e.g., Potter sequence) (see p. 384) |
Syndrome | A group of malformations of different structures due to a single primary cause, but acting through multiple developmental pathways (e.g., trisomy 13 syndrome) (see Fig. 8.10) |
Association | A group of anomalies seen in more than one individual that cannot yet be attributed to a definitive cause |
Based on Spranger J and others: J Pediatr 100:160-165, 1982.
Well-known correlations exist between parental age and the incidence of certain malformations. A classic correlation is the increased incidence of Down syndrome (Fig. 8.3; see Fig. 8.9) in children born to women older than 35 years of age. Other conditions are related to paternal age (see Fig. 8.3).
Some types of anomalies have a higher incidence among infants born at certain seasons of the year. Anencephaly (Fig. 8.4) occurs more frequently in January. Recognizing that the primary factors leading to anencephaly occur during the first month of embryonic life, researchers must seek the potential environmental causes that are more prevalent in April. Anencephaly has been shown to be highly correlated with maternal folic acid deficiency. The high incidence of this anomaly in pregnancies beginning in the early spring may relate to nutritional deficiencies of mothers during the late winter months. Folic acid supplementation in the diet of women of childbearing age significantly reduces the incidence of neural tube defects, such as anencephaly.
The relationship between the country of residence and an increased incidence of specific malformations can be related to various factors, including racial tendencies, local environmental factors, and even governmental policies. A classic example of the last is the incidence of severely malformed infants as a result of exposure to thalidomide. These cases were concentrated in West Germany and Australia because the drug was commonly sold in these locations. Because thalidomide was not approved by the Food and Drug Administration, the United States was spared from this epidemic of birth defects. Another classic example of the influence of country as a factor in the incidence of malformations is seen in neural tube defects (Table 8.2). The reason neural tube defects (especially anencephaly) were historically so common in Ireland has been the topic of much speculation. In light of the recognition of the importance of folic acid in the prevention of neural tube defects, it is possible that the high incidence of anencephaly in Ireland resulted from poor nutrition in pregnant women during the winter. A greater than threefold decrease in the incidence of neural tube defects in Ireland from 1980 to 1994 may be related to both better nutrition and folic acid supplementation by a certain percentage of pregnant women.
Periods of Susceptibility to Abnormal Development
Typically, a developing organ has a curve of susceptibility to teratogenic influences similar to that illustrated in Figure 8.5. Before the critical period, exposure to a known teratogen has little influence on development. During the first days of the critical period, the susceptibility, measured as incidence or severity of malformation, increases sharply and then declines over a much longer period.
Different organs have different periods of susceptibility during embryogenesis (Fig. 8.6). Organs that form the earliest (e.g., heart) tend to be sensitive to the effects of teratogens earlier than organs that form later (e.g., external genitalia). Some very complex organs, especially the brain and major sense organs, show prolonged periods of high susceptibility to disruption of normal development.
Not all teratogenic influences act in the same developmental periods (Table 8.3). Some influences cause anomalies if the embryo is exposed to them early in development, but they are innocuous at later periods of pregnancy. Others affect only later developmental periods. A good example of the former is thalidomide, which has a very narrow and well-defined danger zone during the embryonic period (4 to 6 weeks). In contrast, tetracycline, which stains bony structures and teeth, exerts its effects after hard skeletal structures in the fetus have formed.
Table 8.3
Developmental Times at Which Various Human Teratogens Exert Their Effects
Teratogens | Critical Periods (Gestational Days) | Common Malformations |
Rubella virus | 0-60 | Cataract or heart malformations |
0-120+ | Deafness | |
Thalidomide | 21-40 | Reduction defects of limbs |
Androgenic steroids | Earlier than 90 | Clitoral hypertrophy and labial fusion |
Later than 90 | Clitoral hypertrophy only | |
Warfarin (Coumadin) anticoagulants | Earlier than 100 | Nasal hypoplasia |
Later than 100 | Possible mental retardation | |
Radioiodine therapy | Later than 65-70 | Fetal thyroid deficiency |
Tetracycline | Later than 120 | Staining of dental enamel in primary teeth |
Later than 250 | Staining of crowns of permanent teeth |
Adapted from Persaud TVN, Chudley AE, Skalko RG, eds: Basic concepts in teratology, New York, 1985, Liss.
Causes of Malformations
Despite considerable research since the 1960s, the cause of at least 50% of human congenital malformations remains unknown (Fig. 8.7). Roughly 18% of malformations can be attributed to genetic causes (chromosomal defects or mutations based on mendelian genetics), and 7% of malformations are caused by environmental factors, such as physical or chemical teratogens. Of all malformations, 25% are multifactorial, for example, caused by environmental factors acting on genetic susceptibility.
Genetic Factors
Abnormal Chromosome Numbers
Monosomy and Trisomy
Monosomy (the lack of one member of a chromosome pair) and trisomy (a triplet instead of the normal chromosome pair) are typically the result of nondisjunction during meiosis (see Fig. 1.7). When this happens, one gamete shows monosomy, and the other shows trisomy of the same chromosome.
In most cases, embryos with monosomy of the autosomes or sex chromosomes are not viable. Some individuals with monosomy of the sex chromosomes (45XO genotype) can survive, however (Fig. 8.8). Such individuals, who are said to have Turner’s syndrome, exhibit a female phenotype, but the gonads are sterile.
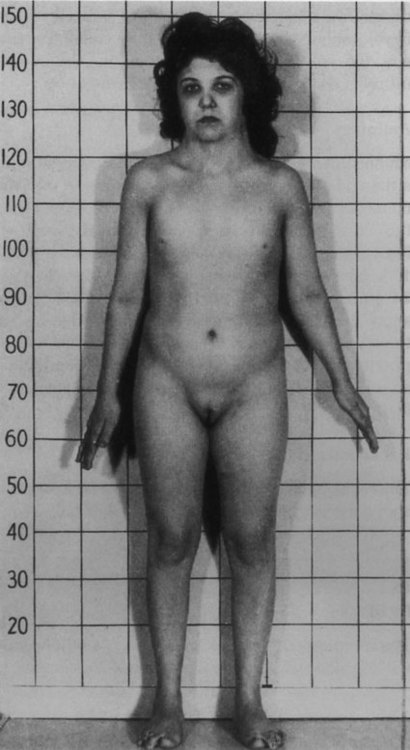
Note the short stature, webbed neck, and infantile sexual characteristics. (From Connor J, Ferguson-Smith M: Essential medical genetics, ed 2, Oxford, 1987, Blackwell Scientific.)
Three autosomal trisomies produce infants with characteristic associations of anomalies. The best known is trisomy 21, also called Down syndrome. Individuals with Down syndrome are typically mentally retarded and have a characteristic broad face with a flat nasal bridge, wide-set eyes, and prominent epicanthic folds. The hands are also broad, and the palmar surface is marked by a characteristic transverse simian crease (Fig. 8.9). Heart defects, especially atrial and ventricular septal defects, are common, with an incidence approaching 50%. Duodenal atresia and other intestinal anomalies are also seen in patients with Down syndrome. Individuals with Down syndrome are prone to the early appearance of Alzheimer’s disease and typically have a shortened life span.
Trisomies of chromosomes 13 and 18 result in severely malformed fetuses, many of which do not survive to birth. Infants with trisomy 13 and trisomy 18 show severe mental retardation and other defects of the central nervous system. Cleft lip and cleft palate are common. Polydactyly is often seen in trisomy 13, and infants with both syndromes exhibit other anomalies of the extremities, such as “rocker bottom feet,” meaning a rounding under and protrusion of the heels (Fig. 8.10). Most infants born with trisomy 13 or trisomy 18 die within the first 1 or 2 months after birth.
Abnormal numbers of the sex chromosomes are relatively common and can be detected by examination of the sex chromatin (X chromosome) or the fluorescence reactions of the Y chromosomes. Table 8.4 summarizes some of the various types of deletions and duplications of the sex chromosomes.
Table 8.4
Variations in Numbers of Sex Chromosomes