Antibodies and In Vivo Therapy
Learning Objectives
• Discuss the problems associated with the use of animal antisera
• Explain the immunologic mechanisms involved in the evolution of serum sickness
• Recognize the constituents of fractionated immunoglobulin (Ig)
• Identify the conditions and diseases treated with intravenous immunoglobulin (IVIG)
• Identify the signs and symptoms of idiopathic thrombocytopenic purpura (ITP)
• Recognize the immunologic mechanism involved in ITP
• Explain the role of IVIG in inhibiting the progression of ITP
• Discuss the pathophysiology of Kawasaki disease
• Explain the relationship between IVIG and complement in Kawasaki disease
• Recall the role of pooled Ig in treatment of Rh blood group incompatibility
• Identify the role of IVIG in the downregulation of T cell–mediated autoimmune diseases
• Compare and contrast the advantages and limitations of pooled human Ig, and monoclonal antibodies
• Compare and contrast DNA de novo synthesis and the nucleic acid salvage pathways
• Explain the biologic basis for HAT (hypoxanthine-aminopterin-thymidine) selection
• Compare and contrast murine, chimeric, humanized, and human monoclonal antibodies
• Recall the guidelines used in the generic names for monoclonal antibodies used in immunotherapy
• Define bi-specific antibodies
• Recognize the characteristics of bi-functional and flex antibodies
• Define single-chain variable fragments (scFv)
• Restate the three critical issues in the development of radiolabeled antibodies
• Identify two antibodies used for the treatment of non-Hodgkin’s lymphoma
Key Terms
Active immunity
Chimeric antibodies
Classic nucleic acid pathway
Erythroblastosis fetalis
HAT selection
Hybridoma
Humanized monoclonal antibodies
Idiopathic thrombocytopenic purpura
Kawasaki disease
Monoclonal antibody
Nucleic acid salvage pathway
Passive immunity
Serum sickness
Single-chain variable fragment (scFv) protein
Introduction
Immunity can be generated by active or passive means. Active immunity results from exposure to microbes such as Streptococcus and Pneumococcus or vaccination with dead or weakened microbes. In contrast, the administration of antibodies to correct an immunodeficiency or provide short-term immunity against microbial infections is called passive immunity. Transfer of antibodies in colostrum from mother to child or administration of pooled immunoglobulins are examples of natural and artificial passive immunity.
Heterologous Antisera to Provide Passive Immunity
The administration of antibodies to provide passive immunity is not a new concept. In the 1890s, Emil von Behring introduced the concept of passive immunity for the treatment of diseases. He demonstrated that equine polyvalent antiserum was efficacious in the treatment of diphtheria infections in children. In the early 1900s, equine antiserum therapy was used to treat streptococcal pneumonia, bacterial meningitis, and Haemophilus infections. By 1950, antibody therapy had been supplanted by antibiotics, which were cheaper to produce. However, equine antisera are still used for the neutralization of anthrax, tetanus, gas gangrene and diphtheria exotoxins; snake venoms; and the rabies virus. Immunogenicity is a major problem associated with heterologous antisera. Anaphylaxis and serum sickness have been frequently encountered in their use.
Serum Sickness
Serum sickness is caused by the administration of large amounts of any foreign protein. Within 7 to 10 days after administration, an immunoglobulin M (IgM) antibody response to the foreign protein develops. When equal molar concentrations of antigens and antibodies are generated, large complexes settle out in the internal elastic lamina of arteries and perivascular regions. Activation of the complement cascade and the release of cytotoxic factors from endothelial cells cause the necrosis of the capillary vessels in the skin and kidneys.
Patients develop clinical manifestations of fever, arthralgia, lymphadenopathy, and skin eruptions. Serious side effects also have been reported as a consequence of antibody therapy. High concentrations of infused antibody (0.5 to 2.0 mg/kg) can cause renal failure, aseptic meningitis, and thrombosis.
Pooled Human Immunoglobulin
To reduce the morbidity associated with heterologous antisera, fractionated human immunoglobulin is now used as a therapeutic agent. Pooled human immunoglobulin is prepared from a panel of 3000 to 10,000 donors and contains 95% intact, unmodified IgG with trace amounts of IgA and IgM. Preparations also contain the macrophage–granulocyte stimulating factor, interleukin 1 (IL-1), and complement regulators.
Initially, pooled human immunoglobulin was administered by the intramuscular (IM) route. Although effective in reducing the frequency of infections, it was difficult to maintain optimal antibody levels in blood. In 1981, intravenous preparations of human immunoglobulin (IVIG) became available. IVIG allows the infusion of large volumes of antibodies while reducing the cost and the pain associated with intramuscular administration.
IVIG has been used to treat immunodeficiencies, human immunodeficiency virus (HIV) infection in infants, lymphoproliferative malignancies, and infections in low-birth-weight infants. It is also used to treat a wide range of autoimmune diseases such as myasthenia gravis, systemic lupus erythematosus, and autoimmune neuropathies (Box 13-1).
IVIG is particularly useful in the treatment of diseases such as idiopathic thrombocytopenic purpura (ITP) and Kawasaki disease and for the prevention of erythroblastosis fetalis.
Idiopathic Thrombocytopenic Purpura
ITP is a pediatric disease that is associated with low platelet counts. Children often present with bruises all over the body, bleeding gums, and, occasionally, a bleeding nose. Patients with persistently low platelet counts are also at high risk of intracranial hemorrhage.
ITP is an autoimmune disease characterized by antibody-coated platelets. In most cases, coated platelets bind to Fc receptors on macrophages in the spleen and liver. Following phagocytosis, platelets are destroyed by the oxidative killing mechanism. In a small proportion of patients, the liver fails to remove the platelets, and circulating antibody–platelet immune complexes are created. Complexes localize in arterioles and capillaries, occluding vessels and causing tissue ischemia. Vessels in the heart, kidney, brain, pancreas, and spleen are common targets for immune complex deposition.
Treatment of Idiopathic Thrombocytopenic Purpura
Administration of IVIG reduces autoantibody levels and disease symptoms. The mechanism is both simple and elegant. Pooled IVIG saturates specialized neonatal Fc receptors (FcRn) on endothelial cells. When the receptor is engaged, IgG is internalized by pinocytosis and recycled to the cell surface, thus preventing lysosomal degradation (Figure 13-1).
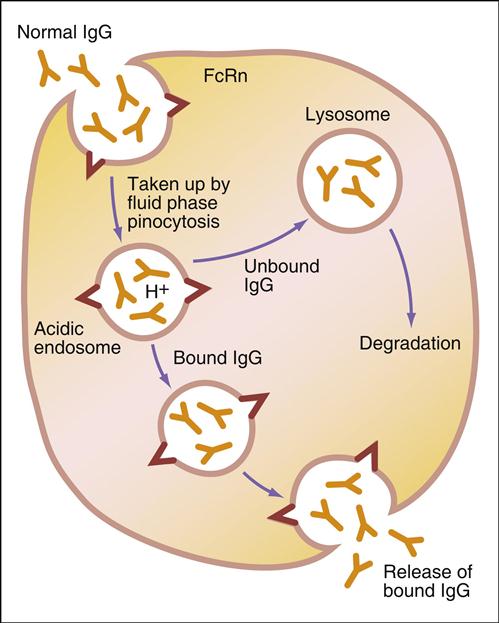
In contrast, serum autoantibodies binding to other Fc receptors on the same cells are internalized and destroyed, thereby arresting the disease process but not curing the disease.
Kawasaki Disease
Kawasaki disease is a self-limiting, febrile disease that is characterized by vasculitis of peripheral and coronary vessels. Patients present with a persistent rash, conjunctivitis, and lymphadenopathy. Individuals with Kawasaki disease have a high risk of heart failure, pericarditis, mitral or aortic valve insufficiency, and rupture of the coronary artery. In the last 5 years, Kawasaki disease has emerged as the leading cause of heart disease in the United States. Although the etiology of the disease is unclear, an immune response to a viral (parvovirus B19 or cytomegalovirus) or bacterial (Yersinia) infection is believed to activate an autoimmune response to vascular endothelial cells and cardiac myosin.
Treatment of Kawasaki Disease
Infusion of IVIG reduces the symptoms by inhibiting the formation of a C3 convertase. Microaggregates within the IVIG displace C3b from tissue-bound immune complexes to the fluid phase, which downregulates the inflammatory response. IVIG also downregulates an inflammatory response by scavenging the anaphylatoxins (C3a and C5b) via low-affinity F(ab′)2 receptors in microaggregated immunoglobulin. In children with early disease, one regimen of IVIG may reduce the symptoms and progression of the disease for several years.
Erythroblastosis Fetalis
Pooled immunoglobulin is also used to prevent erythroblastosis fetalis in neonates caused by Rh factor incompatibility between mother and child (see Chapter 3). Generally, the mother is Rh negative (Rh–) and the fetus is Rh positive (Rh+). Spillage of fetal blood into the maternal circulation elicits an immune response that destroys the infant’s blood cells. When the fetal bone marrow cannot compensate for the destruction of red blood cells, severe anemia occurs. Administration of RhoGAM (anti-Rh) is sufficient to prevent the stimulation of the maternal immune response to Rh+ red blood cells from the fetus.
Intravenous Immunoglobulin and T Cell–Mediated Autoimmune Diseases
Although Fc saturation and complement inhibition explain the IVIG immunomodulation of antibody-mediated autoimmune diseases, it does not account for the downregulation of autoimmune diseases mediated by auto-reactive T cells. In animal models of diseases mediated by T cells, infusion of immunoglobulin increases the number and function of CD4 regulatory cells or downregulates the function of T cells by inhibiting the expression of B7-1 by dendritic cells. Engagement of B7-1 and CD28 on T cells provides the second signal required for T cell activation (see Chapter 6).