CHAPTER 30 Anesthesia for the Pediatric Trauma Patient
Trauma is the forceful disruption of bodily homeostasis that affects physical, psychological, and family functioning, and it remains the number one pediatric public health problem worldwide (Jurkovich et al., 2004). Each year injuries kill more children in the United States than the combined number of pediatric deaths from cancer, congenital anomalies, heart and chronic respiratory diseases, influenza, pneumonia, septicemia, and cerebrovascular disease (Centers for Disease Control and Prevention [CDC], 2002). Over 6 million children are evaluated and treated annually in emergency departments for their injuries, and of these, 500,000 children require hospitalization. Approximately 92,000 children annually become permanently physically disabled as a result of their injuries (Baker et al., 1992; Department of Rehabilitation Medicine, 1995; Tuggle, 1998; CDC, 2002; National Center for Injury Control and Prevention, 2005). Many children who survive their injuries are burdened with lifelong physical limitations or disabilities. For every 400 pediatric injuries, 250 require treatment in the emergency room, 16 are admitted to the hospital, and 1 dies as a result of the injuries (National Center for Injury Control and Prevention, 2005). Trauma care for children includes prevention, treatment and resuscitation, and rehabilitation.
Risk of death from injury can occur during any of three critical times. The first occurs at the time of injury, the second is within the first few hours after trauma, and the third is some time later as a result of complications from the injuries sustained at the time of the traumatic event. Development of effective regional trauma systems and educational programs, such as the Advanced Trauma Life Support for Doctors course, has contributed significantly to the reduction in mortality during the second and third of these critical periods (Tuggle, 1998; Rogers et al., 1999). Unfortunately, however, over 70% of mortality from trauma occurs at the time of injury.
Epidemiology
In 2006, approximately 6 million children received treatment in emergency departments for nonfatal injuries, and of these over 500,000 children needed hospitalization (Child Trends Data Bank, 2005). Injuries can be classified as either intentional or unintentional; most children’s injuries are unintentional. Falls (32.4%) are the leading cause of nonfatal, unintentional injuries among hospitalized children 1 to 14 years of age, followed by injuries sustained as a result of being an occupant in a motor vehicle crash (12.3%) (National Center for Injury Control and Prevention, 2006). The rate of injury and the morbidity and mortality rates remain relatively stable until age 14, when they begin to increase substantially. Males are injured more commonly than females. Motor vehicle accidents are responsible for 75% of pediatric traumatic deaths (DiScala, 2002). Intracranial injuries are the cause of most pediatric traumatic deaths. Unintentional injuries are more prevalent among children who are male, poor, and black or Native American (Safe Kids USA, 2005). In 2004, there were 654,647 (715/100,000 population) nonfatal intentional injuries and 4738 (5.8/100,000 population) fatal intentional injuries in children 19 years and younger. Abuse (physical and sexual), followed by self harm were the major causes of intentional injuries (National Center for Injury Control and Prevention, 2005).
Injuries can be classified as either blunt or penetrating. Blunt injures far outnumber penetrating injuries (12:1), and whereas most are unintentional, up to 7% of injuries are a result of physical assault or abuse (DiScala, 2002). See related video online at www.expertconsult.com.
Pediatric trauma systems
Historical Development
In North America, improvements to trauma care began in the 1960s and 1970s (Trunkey, 1983; Haller, 1995; Mullins, 1999; Morrison et al., 2002). Pediatric trauma systems did not develop in isolation but in concert with adult care. Trauma care was advanced in both the Korean and Vietnam wars, with the military experiences of treating injured soldiers. A report by Howard (1966) highlighted the enormity of the injury problem and stressed its significance as a neglected public health problem. Furthermore, a series of “preventable death studies” was published that provided fodder for community and political support of coordinated care for injured patients. Funding for emergency medical services first became available in 1966 with the National Highway Safety Act, and further support followed with the Emergency Medical Services System Act in 1973 (Mullins, 1999). In 1968, Cook County hospital in Chicago (for adult care) and Kings County hospital in Brooklyn (for pediatrics) were recognized as the first specialized centers for civilian trauma care in the United States. In 1969, the University of Maryland and the Maryland State police developed a coordinated transport system for injured patients to preferentially take them to a hospital with specific interest in caring for injured patients (Cowley et al., 1973; Cowley and Scanlan, 1979). Illinois is credited as the first state to develop a comprehensive state-wide trauma system that included categorization of trauma hospitals and the establishment of a communication system and a trauma registry (Boyd et al., 1973). In 1984, the Department of Health and Human Services, in conjunction with the National Highway Traffic Safety Administration, began the Emergency Medical Services for Children Program in the United States. This funding has been a critical resource for developing programs and research to improve emergency service systems for children.
The American College of Surgeons Committee on Trauma (ACSCOT) has also strived to improve trauma care in North America. This has been accomplished through the publication of standards for trauma systems, the creation of educational courses for health care professionals, and the establishment of and verification programs to ensure published standards are met. In 1976, the first version of the Optimal Resources for Care of Seriously Injured was released (Committee on Trauma: American College of Surgeons, 1999). The most recent version of this publication (2006) is currently in use and has been retitled “Resources for the Optimal Care of Injured Patient.” It remains a dynamic and important document. This publication established criteria for defining levels of trauma centers (I through III) and trauma systems (I and II), and it defined resource requirements for prehospital care through discharge, as well as specific pediatric needs. Level I centers offer the widest range of services for the most severely injured patient, whereas level III centers allow for stabilization and triage. With respect to trauma system development, eight criteria were defined and have become accepted benchmarks for trauma care (Boxes 30-1 and 30-2). Recently, designation of a level IV trauma center has occurred in some states where the resources do not exist for a level III trauma center. Level IV trauma centers are able to provide initial evaluation, stabilization, preliminary diagnoses, and transportation to a center with a higher level of care. These centers may also provide surgery and critical care services (as defined in the scope of services of trauma care). A trained trauma nurse and physicians are available on the patients’ arrival to the emergency department.
Box 30-1 American College of Surgeons Trauma Center Levels and Descriptions
From MacKenzie EJ, Hoyt DB, Sacra JC, et al: National Inventory of Hospital Trauma Centers, JAMA 289(12):1515–1522, 2003.
Box 30-2 American College of Surgeons Criteria for Trauma System Development
From Adler P: Directorate for epidemiology, Washington, DC, 1994, U.S. Consumer Product Safety Commission.
Another valuable contribution by the ACSCOT is the Advanced Trauma Life Support course (ATLS). First released in 1979, the course is now taught all over the world (American College of Surgeons Committee on Trauma, 1997). The first pediatric chapter was introduced in 1983. Although initially designed for surgeons, a wide variety of health care professionals involved in trauma care participate today. The ATLS course provides a common language, framework, and approach to injured patients that facilitate communication between health care professionals in order to optimize and prioritize care. Regular recertification is mandated to keep physicians current with new advances in trauma management. Similar to other ACSCOT programs, the ATLS course is constantly evolving. The current version is the seventh edition.
Trauma System Components
A trauma system is not simply an isolated hospital that cares for injured patients but a broad coalition of participants that includes an integrated approach to trauma. This includes prevention, prehospital care, and hospital care through rehabilitation (Ehrlich et al., 2001, 2002).
A primary step in developing an effective trauma system is a needs assessment of the community that it serves. For example, rural trauma systems differ from those in urban settings—each have different mortality rates, injury patterns, geographic areas, available resources, and expertise (Rogers et al., 1999; Ehrlich et al., 2004). Community responsibility and involvement (particularly with injury prevention) are essential for political support and funding. Studies support the concept that community-based injury-prevention programs play a substantial role in reducing morbidity and mortality (Hulka et al., 1997; Nathens et al., 2000).
A second important consideration in developing a regional trauma system is to understand that all facilities have a role in treating injured patients. A tiered approach (e.g., Levels I through IV) with specific hospitals designated to care for the varied complexity of injured patients is essential. Level I hospitals are tertiary or quaternary centers, with levels II and III centers having fewer capabilities and resources. Level IV hospitals are distinct because of their remote nature (rural trauma). A list of recommended requirements for each level of care can be found in the ACSCOT publication Resources for the Optimal Care of the Injured patient: 2006 (Committee on Trauma: American College of Surgeons, 1999). A basic tenet and overarching requirement for this tiered system is a central communication (central command) structure and a well-developed triage system so that the most severely injured patients go first to the appropriately designated hospital. In addition, a trauma registry system is essential for outcomes analysis and quality-assurance programs.
Anesthesiologists play a variety of roles in trauma response. These roles span from assisting as primary responders in airway management and vascular access to coordinating operating-room resources to ensure that patients are treated in a timely and effective fashion. A pediatric anesthesiologist is an invaluable resource when treating injured children. A 1993 report from the Institute of Medicine identified specific pediatric emergency medical service deficiencies in rural states (Institute of Medicine, 1993), including the pediatric airway management, vascular access, and special resuscitation needs of injured children. Airway control is a primary tenet of trauma care, and proper endotracheal intubation (ETI) is recognized as a definitive method to achieve airway control. ETI, however, in injured children is required less often than in adults; hence, maintaining skills can be difficult for health care providers (Baker et al., 2009). Several studies have looked at airway management in pediatric trauma and examined significant aspects, including how success and complication rates of ETI vary by location (e.g., field, transferring hospital, or trauma center) and personnel, whether bag-mask ventilation is effective with respect to oxygenation and ventilation before ETI, and whether multiple attempts at intubation adversely affect patient care or outcomes (Cooper et al., 1992; Gausche et al., 2000; Glaeser, 2000; Ehrlich et al., 2004). These studies have demonstrated that field intubation success rates are poor. Successful pediatric intubations relate to the skill of the person performing the intubation, and anesthesiologists’ airway management skills are considered superior to those of all other health care professionals. Failed attempts at ETI appear to produce a spiral effect that results in multiple failed attempts and delayed transfer to definitive care. Complications resulting from ETI seem far more common when the intubation is attempted in the field and the risk of complication is exacerbated with each attempt. Increased complication rates do impact patient recovery, as shown by longer durations of hospitalization (Cooper et al., 1992; Gausche et al., 2000; Glaeser, 2000).
Trauma scoring systems
Triage Scores: Where Is the Injured Child Best Treated?
An ideal triage scoring system would have few data points, be easy to apply, have limited subjective assessments, and have a high sensitivity and specificity. Unfortunately, no triage score accomplishes all of these tasks, but each system does have strengths and limitations. For example, studies have shown that assessments made in the field by paramedics are less accurate and reliable for pediatric trauma patients when compared with adults (Engum, 2000). Alternatively, comparisons between adult and pediatric trauma triage scores have not shown that a pediatric specific trauma score provides a significant advantage over the original adult measure.
Glasgow Coma Scale
In 1974, Teasdale and Jennett first introduced the GCS. A lower score reflects a lower level of consciousness and therefore a potentially more serious head injury. Scores can range from 3 to a maximum of 15 (normal). The GCS measures three specific components of consciousness (eye movement, verbal, and motor responses) with higher scores (up to 5) given to the best response. A patient with a GCS of 13 to 14 is considered to have a mild head injury; 9 to 12 indicates a moderate injury; and a score of 8 or lower indicates a severe insult. Adaptations of the GCS for the pediatric population have occurred, and a Pediatric GCS is now widely used (Table 30-1).
GCS scores determined in the field are less predictive of outcome than those generated at a hospital (Meredith et al., 1995). When each individual component of the GCS is evaluated, the motor component is the strongest predictor of outcome.
Revised Trauma Score
The revised trauma score (RTS) is a physiologic-based triage score (Table 30-2). The RTS was derived from two earlier versions of a triage scores, the Triage Index and the Trauma Score (Champion et al., 1980, 1981, 1989). The RTS has three variables—respiratory rate, systolic blood pressure, and GCS. The RTS is the sum of each variable multiplied by a weighted coefficient.
Clinical Measurement | Parameter | Score |
Respiratory rate | 10−24 25−35 >35 <10 0 |
4 3 2 10 |
Systolic blood pressure | >90 70−89 50−69 <50 0 |
4 3 2 1 0 |
Glasgow Coma Scale | 14−15 11−13 8−10 5−7 3−4 |
4 3 2 1 0 |
* The Revised Trauma score is the sum of the weighted variables (see formula in text). The higher the score, the better the prognosis.
From Centers for Disease Control and Prevention: Traumatic brain injury in the United States: a report to Congress, Atlanta, 1999, Department of Health and Human Services National Center for Injury Control and Prevention.
where GCS is the Glasgow Coma Scale; SBP is systolic blood pressure; and RR is respiratory rate. These variables were determined to correlate statistically with survival and mortality. A higher RTS is associated with a better chance of survival. An injured patient with a RTS score of 11 or lower is recommended to be treated at a designated trauma center. Eichelberger established and developed pediatric coefficients for the RTS that was then validated in the pediatric population (Engum et al., 2000). The RTS is also recommended as one of the triage tools in the American College of Surgeons trauma guidelines, Resources for the Optimal Care of the Injured Patient (Committee on Trauma: American College of Surgeons, 1999).
Pediatric Trauma Score
The Pediatric Trauma Score (PTS) was first introduced by Tepas in 1987 (Tepas et al., 1987; Nayduch et al., 1991). Its design was thought to be more reflective of pediatric injuries (Table 30-3). Six variables comprise both physiologic and injury attributes. After a number for each variable is totaled, a score of 8 or lower is considered to be a marker for children requiring care at a designated trauma center. A limitation of the PTS is that some variables are subjectively scored, particularly the central nervous system (CNS) assessment. Therefore, there is a risk of interrater and intrarater variability. The PTS has been compared with the RTS with surprisingly few apparent differences or inherent advantages (Tepas et al., 1987, 1988; Nayduch et al., 1991).
Clinical | Parameter | Score* |
Weight (kg) | >20 10-19 <10 |
2 1 –1 |
Airway | Normal Maintainable Unmaintainable |
2 1 –1 |
Systolic blood pressure | >90 50-89 <50 |
2 1 –1 |
Central nervous system | Awake Obtunded or loss of consciousness Coma or decerebrate |
2 1 –1 |
Open wound | None Minor Major or penetrating |
2 1 –1 |
Skeletal | None Closed fracture Open or multiple fractures |
2 1 –1 |
* A total score of 8 or less suggests care at a designated trauma center.
Data from Tepas JJ III, Mollitt DL, Talbert JL, et al: The pediatric trauma score as a predictor of injury severity in the injured child, J Pediatr Surg 22:14–18, 1987; Tobias JD, Ross AK: Intraosseous infusions: a review for the anesthesiologist with a focus on pediatric use, Anesth Analg 110:391–401, 2010.
Age-Specific PTS
The most recent proposed triage score is the Age Specific PTS (Table 30-4). First described by Potoka et al. in 2001, the main addition is the use of age-specific physiologic variables. To date, long-term data are lacking and only a single study suggests that it is better than traditional adult scores; therefore, the clinical utility as a triage score still remains to be proven. This score is, however, a pediatric-specific tool that addresses areas where other adult scores become less accurate (e.g., extremes of age).
Abbreviated Injury Scale
The Abbreviated Injury Scale (AIS) emerged from the automotive industry in 1969 (JAMA, 1971; Association for the Advancement of Automotive Medicine, 1990). It was initially designed as an epidemiologic tool to describe motorvehicle crashes but has been adapted to all types of trauma (JAMA, 1971; Association for the Advancement of Automotive Medicine, 1990). Revised and updated several times over the years, the first version published was the AIS-90 (for the year 1990) in 1998 (Association for the Advancement of Automotive Medicine, 1990). This score evaluates nine body regions. A scale from 1 to 6 is used to define injuries (1, minor; 2, moderate; 3, serious; 4, severe; 5, critical; and 6, maximal.) The underlying premise is an association of injury with threat to life. A body region with a score of 6 is considered an unsurvivable injury. The scores are determined retrospectively and based primarily on ICD-9-CM codes. A significant amount of expertise is required to assign these ratings, and interrater and intrarater variability is common. To help limit this phenomenon, software has been developed to convert the ICD-9-CM codes directly to an AIS score. Unfortunately, ICD-9-CM coding itself is inherently variable (MacKenzie et al., 1985, 1989).
Injury Severity Score
The Injury Severity Score (ISS) is one of the most widely used scoring systems in the trauma literature. It correlates well with several important trauma outcomes such as mortality and duration of hospitalization. Developed in 1974, the ISS is a method of characterizing the trauma patient with multiple injuries (Baker et al., 1974; Baker and O’Neill, 1976). The ISS is based on the AIS, which describes the severity of injury to different body regions (see the previous section). For example:
Survival Probability: Trauma Score and Injury Severity Score
The Trauma Score and Injury Severity Score (TRISS) is not a score but rather a method of predicting mortality. It was first proposed in 1987 and combines the physiologic variables of the RTS with the anatomic severity-of-injury scores generated by the ISS (Boyd et al., 1987). The final result is that a probability of survival (Ps) is generated.
Where e is the base of the natural log 2.72183, and b is derived from the following formula:
The age factor is 0 for those younger than 55 years of age, and 1 for those who are 55 years of age. The variables b0, b1, b2, and b3 are blunt and penetrating trauma coefficients for adult and pediatric populations (Eichelberger et al., 1993).
TRISS is the most widely used predictor of survival in the trauma literature. As with other statistical processes, limitations exist. These are specifically noted in patients older than 55 years of age (extremes of age), and with trauma patients whose ISS is greater than 25. In fact, some authors suggest that because of the TRISS deficiencies, this method of calculating survival probabilities should be abandoned completely, particularly in urban centers (Cayten et al., 1991; Demetriades et al., 1998).
Application of the TRISS Results (W and Z Scores)
A common application of the TRISS and Ps is to compare practice-based outcomes with population-based outcomes. Typically, this comparison uses a group of national norms that were identified in the Major Trauma Outcomes Study (MTOS) (Champion et al., 1990b). The MTOS data set is derived from 160,000 hospitalized patients at 139 centers between 1982 and 1989. Children represented 11% of this population. From 80,544 adult patients in this data set, regression coefficients for predicting mortality were derived based on the revised trauma score and ISS (TRISS methodology). A single data set can be compared with the MTOS. A “Z score” is generated, whereby
W Scores
W is used when the Z score is found to be significant. W measures the statistical differences between the actual (A) and expected (E) survivors in a patient group. Sample size plays an important role in delineating the clinical significance of the difference between the actual and expected numbers of survivors (Taylor et al., 1986). Consider the following formula:
Both the W and Z scores are limited by the same factors that limit TRISS data.
A Severity Characterization of Trauma Score
A Severity Characterization of Trauma (ASCOT) score is an attempt to revise and fix the limitations found using TRISS methodology (i.e., severely injured patients and extremes of age) (Champion et al., 1990b). ASCOT uses individual components of the RTS (not totaled) and incorporates a modification of AIS entitled The Anatomic Profile. Furthermore, it excludes those patients with either very severe or nonserious injuries (a maximum AIS score of less than 2 and an RTS score of greater than 0; an AIS score of 6 and an RTS score equal to 0). The Anatomic Profile categorizes the AIS scores that are greater than 2 into three groups: head, brain, and spinal cord injuries; thorax or neck injuries; and all other serious injuries. A comparison of ASCOT vs. TRISS with a pediatric population did not provide more reliable or accurate scoring over the TRISS.
Primary and secondary surveys
The first priority is to save a life through identifying and treating all life-threatening illnesses and injuries. To understand and acquire the necessary clinical skills for the management of injured adults and children, it is best to become certified by completing the ATLS course designed by the American Colleges of Surgeons (Schall et al., 2002). The ATLS course is a well-recognized certification for trauma care and is now used worldwide to teach physicians and other health care providers. The ATLS course gives a common approach and language to the care of injured patients, thus allowing a framework for physicians and allied health personnel to communicate.
The initial management of the injured child can be divided into two phases. The first is the primary survey that incorporates the “ABCs” and where all life-threatening injuries are identified and treated. The second phase is the secondary survey, where other injuries that contribute significantly to illness and deaths are identified and treatment is instituted. The ABCs are defined in the following list, and the priorities of the primary survey are further detailed in Box 30-3.
Box 30-3 ABCs of Resuscitation
Data from Tepas JJ 3rd, Mollitt DL, Talbert JL, et al: The pediatric trauma score as a predictor of injury severity in the injured child, J Pediatr Surg 22:14–18, 1987; Tobias JD, Ross AK: Intraosseous infusions: a review for the anesthesiologist with a focus on pediatric use, Anesth Analg 110:391–401, 2010.
During the resuscitation of the injured child, many processes are conducted concurrently (Fig. 30-1). It is important to continually assess, intervene, and reassess within the priorities of the primary survey. The final components of the primary survey are the placement of a Foley catheter and gastric tube unless contraindicated. The contraindications to placing a Foley catheter include pelvic fracture and blood at the tip of the meatus.
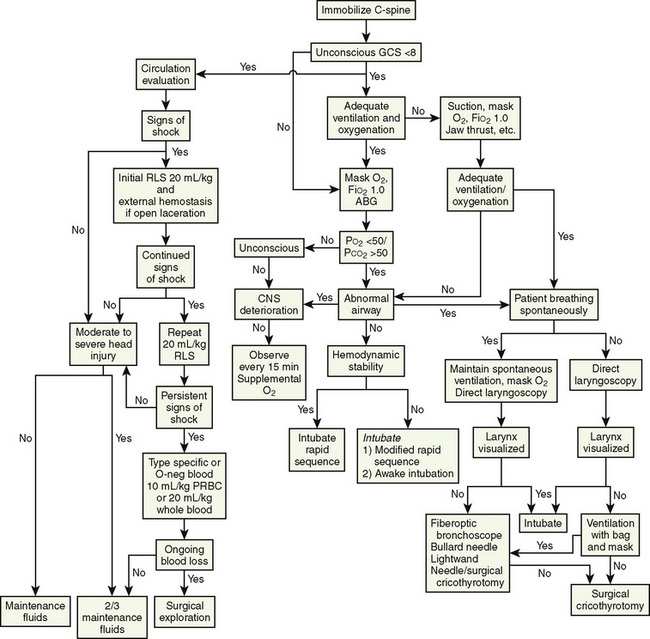
FIGURE 30-1 Guidelines for airway and cardiovascular assessment in the traumatized pediatric patient.
The secondary survey is comprised of a complete physical examination, patient history, laboratory tests, and radiologic imaging. This phase may be delayed or completed in the operating room in patients who require urgent interventions. Historical information about the patient and the event should be collected during the secondary survey. The acronym SAMPLE (standing for Symptoms, Allergies, Medications, Past illnesses, Last meal, Events, and Environment) may be helpful in guiding the trauma team. Definitive care occurs in the intensive care unit or operating room and often involves care by pediatric surgical subspecialists (e.g., neurosurgeons or orthopedic surgeons). Patients may also require transfer to comprehensive trauma centers during this phase (Krantz, 1996).
Airway
The initial management of the pediatric airway involves bag-valve-mask ventilation with a jaw-thrust maneuver. Intubation is indicated in patients with respiratory or cardiac compromise or an altered level of consciousness. All pediatric trauma patients should be considered to have a full stomach and possible cervical spine injury. See related video online at www.expertconsult.com.Because of this, the airway should be secured after a rapid-sequence induction (RSI) (that may include cricoid pressure) with manual inline stabilization and an oral ETI. Nasotracheal intubation may be suboptimal and difficult because of the small size and acute angle of the nasopharynx and the more anterior and cephalad position of the glottic opening.
The RSI for pediatric trauma patients can be accomplished with an induction agent that is immediately followed by a muscle relaxant. Standard induction agents for trauma patients include etomidate (0.2 to 0.3 mg/kg) and ketamine (2 to 4 mg/kg) or the combination of fentanyl (2 to 3 mcg/kg), midazolam (0.05 to 0.1 mg/kg) with lidocaine (1 mg/kg). Sodium thiopental (STP) and propofol should be reserved for patients who are not hemodynamically unstable. STP is an ideal induction agent for patients with head trauma, provided they are not hypovolemic. Ketamine is relatively contraindicated in patients with increased intracranial pressure (ICP). Although etomidate provides hemodynamic stability in trauma patients who are hypovolemic, it may decrease survival in patients with sepsis secondary to adrenal suppression (Annane et al., 2002). Muscle relaxation can be achieved with rocuronium (0.8 to 1.2 mg/kg) or succinylcholine (1 to 1.5 mg/kg). Succinylcholine is contraindicated in crush injuries, long-bone fractures, and patients susceptible to malignant hyperthermia. In patients who are hemodynamically stable, the combination of propofol (4 mg/kg) and remifentanil (3 mcg/kg) can be used for rapid-sequence intubation and has an onset and offset similar to propofol and succinylcholine (1 mg/kg) (Crawford et al., 2005).
ETIs have been deemphasized in the prehospital setting, because they are often unsuccessful. Gausche and others (2000) reported a success rate of only 57%. All intubated trauma patients that come to the emergency department should have the placement of their endotracheal tubes (ETTs) confirmed with either end-tidal carbon dioxide (CO2) or direct laryngoscopy.
If initial intubation attempts are unsuccessful after an RSI, the patient should be ventilated with bag-mask ventilation. If a patient cannot be ventilated or if it is very difficult, a laryngeal mask airway (LMA) can be placed to facilitate ventilation and subsequent intubation. However, it must be recognized that the LMA does not protect the airway from aspiration and must be replaced by an ETT as soon as skilled personnel become available. The stomach should be decompressed with a nasogastric or orogastic tube after intubation, and a chest x-ray should be obtained to verify the ETT position (Fig. 30-2).
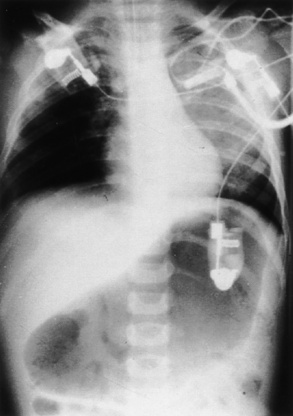
FIGURE 30-2 Gastric dilation often occurs after crying or positive-pressure ventilation by gas and mask.
Depending on the nature of the underlying injury, securing the airway in a patient who has sustained multiple injuries or even isolated facial injuries can be extremely complicated, as illustrated in Figure 30-3. The management of such cases calls upon the resourcefulness and skills of the anesthesiologist and requires careful consideration of damage to surrounding structures such as major blood vessels and the airway structures themselves. The ability to maintain a patent airway via face mask, and the potential for an expanding hematoma that may subsequently compromise an airway that may be patent at the current time, must be anticipated. Additional considerations include the risks of increased ICP with concomitant head trauma, exacerbating an existing cervical spine injury, and aspiration during airway manipulation. The presence of rhinorrhea, otorrhea, or ecchymoses around the eyes should raise suspicion about a possible basilar skull fracture, and any instrumentation of the nasal passages, including passage of a nasal ETT or an N/G tube, should be avoided. Similarly, crepitus at the neck may herald the presence of a tracheal disruption, and intubation under direct vision using a fiberoptic scope should be considered to avoid false passage of the ETT. See related video online at www.expertconsult.com.
In cases in which airway difficulty is anticipated, it may be prudent to transport the child to the operating room with an anesthesiologist and otolaryngologist once the child has been stabilized hemodynamically and additional injuries have been ruled out. The airway may then be secured with preparations to perform an emergent tracheostomy in case of failed laryngoscopy. An inhalational induction may be tolerated by the patient who has been volume resuscitated. This permits direct laryngoscopy or flexible fiberoptic intubation while the patient is breathing spontaneously. In patients who have suffered loss of consciousness or head injury, there should be a high index of suspicion for cervical spine injury, even with the absence of radiologic evidence. When performing laryngoscopy, inline axial stabilization must be performed (Fig. 30-4). Inline axial stabilization is performed by an assistant during laryngoscopy. The assistant should place both arms on either side of the patient’s head while gripping the patient’s shoulders. The assistant’s function is to maintain the patient in a neutral position during laryngoscopy, avoiding flexion, extension, or rotation of the cervical spine. The use of muscle relaxants is best avoided until the airway is secured. If intravenous agents are required to induce anesthesia, it is preferable to use short-acting agents such as propofol and remifentanil that effectively blunt ICP responses to direct laryngoscopy yet permit return of spontaneous respiration in case of failed intubation.
Circulation and Access
Children who sustain multiple injuries often arrive in hypovolemic or hemorrhagic shock that must be promptly recognized and treated. Unlike adults, children maintain an almost normal blood pressure until 25% to 35% of their circulating blood volume is lost (Fig. 30-5). This is likely because of their high sympathetic tone that causes peripheral vasoconstriction in an effort to maintain blood pressure in the face of a diminished blood volume. Therefore, tachycardia is an earlier sign of impending shock than hypotension. Additionally, signs of poor peripheral perfusion such as delayed capillary refill (more than 2 seconds), weak or thready pulses, mottling or cyanosis of the skin, and impaired consciousness are earlier indicators of shock than low blood pressure. The presence of hypotension as a result of hypovolemia should be considered an ominous sign that usually heralds impending cardiovascular collapse. Table 30-5 describes the stages of pediatric shock and clinical signs seen at these stages.
TABLE 30-5 Stages of Pediatric Blood Volume Loss (Shock) and Associated Clinical Signs
Blood Volume Loss | Clinical Signs |
<20% | CV: Tachycardia; weak, thready pulses Skin: Cool to touch, capillary refill 2-3 sec Renal: Slight decrease in urine output, increase in specific gravity CNS: Irritable, may be combative |
25% | CV: Tachycardia; weak, thready distal pulses Skin: Cold extremities, cyanosis, and mottling Renal: Decrease in urine output CNS: Confusion, lethargy |
40% | CV: Frank hypotension; tachycardia may progress to bradycardia Skin: Pale, cold Renal: No urine output CNS: Comatose |
CV, Cardiovascular; CNS, central nervous system.
From Rasmussen GE, Grandes CM: Blood, fluids, and electrolytes in the pediatric trauma patient, Int Anesthesiol Clin 32:79-101, 1994.
It is imperative to rapidly assess the pediatric trauma patient for signs of shock upon arrival in the trauma center and at regular intervals thereafter. The initial fluid bolus administered in the trauma setting is warmed isotonic crystalloid (lactated Ringer’s solution or normal saline) in a bolus of 20 mL/kg, IV (see Fig. 30-1). The pulse, capillary refill, and blood pressure are reassessed. A second bolus of 20 mL/kg is administered if there is no significant response or only a transient improvement in these parameters. A third crystalloid bolus may be given if necessary to maintain appropriate vital signs and circulation. Blood (10 mL/kg) should be administered next if additional fluid resuscitation is required. The need for blood transfusion initially is uncommon and usually signals surgical bleeding that may require an operation.
Pneumothorax is a common complication of blunt chest injury in children, with nearly one fourth of pneumothoraces under tension (Nakayama et al., 1992). Unilateral or bilateral tension pneumothoraces may produce hypotension and hypoxemia. The classic signs of tension pneumothorax are ipsilateral tympany, shift of the trachea to the contralateral side, and distended neck veins.
IO access is placed in the medial surface of the proximal tibia 1 to 3 cm below the tibial tuberosity or the distal femoral metaphysis. IO has been used as a lifesaving measure to establish short-term vascular access in critically ill or injured children (Fig. 30-6).
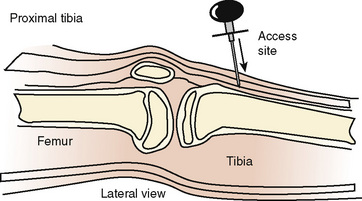
FIGURE 30-6 Appropriate placement of the IO infusion needle on the medial surface, distal to tibial tuberosity.
(From Ellemunter H: Intraosseous lines in preterm and full-term neonates, Arch Dis Child Fetal Neonatal Ed 80:74F, 1999.)
The use of IO infusions in the algorithm of trauma and cardiopulmonary resuscitation has evolved over the years and now takes a more prominent role for providing vascular access to children. The subject of IO has been reviewed by Tobias and Ross (2010).
Access needles for IOs include manual devices such as butterfly needles, spinal needles, bone marrow biopsy needle such as Susfast (Cook Critical Care; Bloomington, Indiana) and the basic Jamshidi needle (Baxter Healthcare Corporation; Deerfield, Illinois). More recently, automated devices have been marketed (Fig. 30-7). The EZ-IO (Vidacare; San Antonio, Texas) functions like a battery-powered drill, with a beveled drill and a preset depth. It is designed to be used in the tibia. The Bone Injection Gun (BIG; Waismed; Kansas City, Missouri) is a spring-locked device also designed to be used in the tibia. It comes in a range of sizes for use in infants, small children, and adults. A device specifically designed for entry into the bone marrow of the sternum is the FAST1 (First Access for Shock and Trauma, PYN6 Medical Corporation; Vancouver, British Columbia). It is approved for patients ages 12 and older. Table 30-6 summarizes these devices. Details regarding instructions on the use of these devices can be viewed on the manufacturers’ websites.
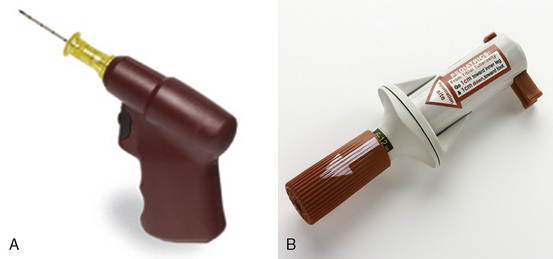
FIGURE 30-7 Automated devices. A, EZ-IO. B, Bone Injection Gun.
(A, Courtesy Vidacare, San Antonio, TX; B, Courtesy Waismed, Kansas City, Mo.)
A review of the use of IO access in pediatric trauma patients up to 10 years of age reported successful placement of access in 28 out of 32 attempts in the prehospital setting and in the trauma care center (Guy et al., 1993). In this study, IO access was placed successfully by paramedics, nurses, and physicians with only one incident of minor extravasation of fluid and no long-term complications in the survivors. The commonest complication of IO access is subperiosteal infiltration that in most cases resolves spontaneously without further problems. The most feared complication—osteomyelitis—occurs in 0.6% cases (Rosetti et al., 1985). Other rare complications include fractures and emboli. Although few complications have been reported with this technique, it must be recognized that the high mortality in patients who require IO access prevents the assessment of long-term complications.
Secondary Survey
Diagnostic Evaluation
The diagnostic evaluation of the injured child involves clinical examination supplemented by radiologic examinations and laboratory testing. Imaging plays a major role in the evaluation of the injured child (Vane, 2002). Improvements in imaging techniques have allowed progress in the nonoperative management of abdominal and thoracic trauma, supplanting exploratory laparotomy and diagnostic peritoneal lavage in many hemodynamically stable patients.