2.2 Anatomy and biomechanics of the pelvis
(Adapted from Chapter 8 of Movement, Stability and Lumbopelvic Pain (Vleeming & Stoeckart 2007).)
In all quadrupeds and bipeds, the pelvic girdle forms a firm connection between the spine and the lower extremities. In bipeds, the pelvis has to serve as a basic platform with three large levers acting on it (the spine and the legs). To allow bipedal gait in humans, evolutionary adaptations of the pelvis have been necessary, i.e. changing the shape of the ilia, flaring out into the sagittal plane, providing a more optimal lateral attachment site for the gluteus medius as an important muscle for hip pelvic stability. In particular, a dramatically increased attachment site for the gluteus maximus muscle has changed this muscle – a relatively minor muscle in the chimpanzee – into one of the largest muscles of the human body (Lovejoy 1988).
Additional evolutionary changes in humans are the muscular and ligamentous connections between the sacrum and ilia: (1) muscles, like the lower lumbar multifidi, that insert into the sacrum and also into the medial cranial aspects of the ilium; (2) changes in the position of the coccygeus and the piriform muscles, and of the gluteus maximus muscle originating from the sacrum and sacrotuberous ligaments; (3) extensive fibrous connections adapted to the typical anatomy of the sacroiliac joints (SIJs), like the interosseous ligaments, surrounding an iliac protrusion fitting in a dorsal sacral cavity, called the axial joint just behind the auricular surfaces of the SIJ (Bakland & Hansen 1984); (4) ventral and dorsal SIJ ligaments, sacrotuberous and sacrospinous ligaments between sacrum and lumbar spine (anterior longitudinal ligaments). In addition, direct fibrous connections exist between the iliac bone and L4 and L5, the iliolumbar ligaments. A recent study has described the influence of the iliolumbar ligaments on SIJ stability (Pool-Goudzwaard et al. 2003). Due to the above-mentioned muscular and ligamentous connections, movement of the sacrum with respect to the iliac bones, or vice versa, affects the joints between L5–S1 and between the higher lumbar levels. Anatomical and functional disturbances of the pelvis or lumbar region influence each other. Due to the tightness of the fibrous connections and the specific architecture of the SIJ, mobility in the SIJ is normally very limited, but movement does occur and has not been scientifically challenged (Weisl 1955, Solonen 1957, Egund et al. 1978, Lavignolle et al. 1983, Miller et al. 1987, Sturesson et al. 1989, 2000a, 2000b, Vleeming et al. 1990a, 1990b, 1996) (Figure 2.2.1).
The main movements in the SIJ are forward rotation of the sacrum relative to the iliac bones (nutation) and backward rotation of the sacrum relative to the ilia (counternutation) (Figure 2.2.2). It was shown that even at advanced age (>72 years) the combined movement of nutation and counternutation can amount to 4°; normally movements are less than 2° (Vleeming et al. 1992a). In the latter study, the SIJ with the lowest mobility showed radiologically marked arthrosis. Ankylosis of the SIJ was found to be an exception, even at advanced age. This finding is in agreement with the studies of Stewart (1984) and Miller et al. (1987).
Nutation is increased in load-bearing situations, e.g. standing and sitting. In lying prone, nutation is also increased compared to supine positions (Weisl 1955, Egund et al. 1978, Sturesson et al. 1989, 2000a, 2000b). Counternutation normally occurs in unloaded situations like lying down (supine). Counternutation in supine positions can be altered to nutation by maximal flexion in the hips, using the legs as levers to posteriorly rotate the iliac bones relative to the sacrum, as in a labour position, creating space for the head of the baby during delivery.
The anatomy of the sacroiliac joint
1. Why did nature create a seemingly flat SIJ?
2. What specific adaptations are available to prevent shear in the SIJs?
3. Why is the SIJ not perpendicularly orientated to the forces of gravitation?
Why did nature create a seemingly flat SIJ?
A large transfer of forces is required in the human SIJ, and indeed flat joints are theoretically well-suited to transfer large forces (Snijders et al. 1993a, 1993b). An alternative for effective load transfer by these flat joints would be a fixed connection between sacrum and iliac bones, for instance by ankylosis of the SIJ. The SIJs in humans serve a purpose: to economize gait, to allow shock and shear absorption, and to alleviate birth of (in the evolutionary sense) abnormally large babies. The principal function of the SIJs is to act as stress relievers, ensuring that the pelvic girdle is not a solid ring of bone that could easily crack under the stresses to which it is subjected (Adams et al. 2002).
What specific adaptations are available to prevent shear in the SIJs?
The SIJs are abnormal compared to other joints because of cartilage changes that are present already before birth. These occur especially at the iliac side of the joint and were misinterpreted as degenerative arthrosis (Sashin 1930, Bowen & Cassidy 1981). These cartilage changes are more prominent in men than in women and, according to Salsabili et al. (1995), the sacral cartilage is relatively thick in females. This gender difference might be related to childbearing and possibly to a different localization of the centre of gravity in relation to the SIJ (Dijkstra et al. 1989, Vleeming et al. 1990a, 1990b). Vleeming et al. (1990a, 1990b) considered these changes to reflect a functional adaptation. The features seem to be promoted by the increase in body weight during the pubertal growth spurt and concern a coarse cartilage texture and a wedge and propeller-like form of the joint surfaces.
Studies of frontal slides of intact joints of embalmed specimens show the presence of cartilage-covered bone extensions protruding into the joint. These protrusions seemed irregular but are in fact complementary ridges and grooves. Joint samples taken from normal SIJ with both coarse texture and complementary ridges and grooves were characterized by high-friction coefficients (Vleeming et al. 1990b). All these features are expected to reflect adaptation to human bipedality, contributing to a high coefficient of friction and enhancing the stability of the joint against shear (Vleeming et al. 1990a). As a consequence, less muscle and ligament force is required to bear the upper part of the body (Figure 2.2.3).
The ‘keystone-like’ bony architecture of the sacrum further contributes to its stability within the pelvic ring. The bone is wider cranially than caudally, and wider anteriorly than posteriorly. Such a configuration permits the sacrum to become ‘wedged’ cranially and dorsally into the ilia within the pelvic ring (Vleeming et al. 1990a, 1990b). The SIJ has evolved from a relatively flat joint into a much more stable construction (Figure 2.2.4).
To illustrate the importance of friction in the SIJ, the principles of form and force closure were introduced (Vleeming et al. 1990a, 1990b). Form closure refers to a theoretical stable situation with closely fitting joint surfaces, where no extra forces are needed to maintain the state of the system, given the actual load situation. If the sacrum would fit in the pelvis with perfect form closure, no lateral forces would be needed. However, such a construction would make mobility practically impossible. With force closure (leading to joint compression) both a lateral force and friction are needed to withstand vertical load. Shear in the SIJ is prevented by the combination of the specific anatomical features (form closure; see Figure 2.2.5) and the compression generated by muscles and ligaments that can be accommodated to the specific loading situation (force closure). Force closure is the effect of changing joint reaction forces generated by tension in ligaments, fasciae and muscles and ground reaction forces (Figure 2.2.5).
Why is the SIJ not perpendicularly orientated to the forces of gravitation?
Force closure ideally generates a perpendicular reaction force to the SIJ to overcome the forces of gravity (Vleeming et al. 1990b). This shear prevention system was named the self-bracing mechanism and such a mechanism is present elsewhere in the body, e.g. in the knee, foot and shoulder. When a larger lever is applied and/or coordination time becomes less, the general effect in the locomotor system will be closure or reduction of the degrees of freedom of the kinematic chain, leading to a reduction in the chain’s mobility or a gain of stability by increasing force closure (Huson 1997).
In self-bracing of the pelvis, nutation of the sacrum is crucial. This movement can be seen as an anticipation for joint loading. Hodges et al. (2003) use the terminology ‘preparatory motion’ for a comparable phenomenon in the lumbar spine. So, nutation is seen as a movement to prepare the pelvis for increased loading by tightening most of the SIJ ligaments, among which are the vast interosseous and short dorsal sacroiliac ligaments. As a consequence the posterior parts of the iliac bones are pressed together, enlarging compression of the SIJ.
Ligaments and their role in self-bracing the pelvis
In self-bracing the pelvis, nutation in the SIJ is crucial (see above); this involves several ligaments. To further explain self-bracing of the pelvis we will discuss two sets of ligaments (Figure 2.2.6): the sacrotuberous ligaments (Vleeming et al. 1989a, 1989b, van Wingerden et al. 1993) and the long dorsal SI ligaments (Vleeming et al. 1996, 2002). In the literature, specific data on the functional and clinical relevance of the long ligaments are not available. In several anatomical atlases and textbooks, the long ligament and the sacrotuberous ligament are portrayed as fully continuous ligaments. The drawings generally convey the impression that the ligaments have identical functions. As shown by the contrasting effects of nutation and contranutation on these ligaments (see below), this is not the case. Essentially, the long ligament connects the sacrum and posterior superior iliac spine (PSIS), whereas the main part of the sacrotuberous ligament connects the sacrum and ischial tuberosity. However, some of the fibres derived from the ischial tuberosity pass to the iliac bone. Generally, they are denoted as part of the sacrotuberous ligament, although ‘tuberoiliac ligament’ would be more appropriate. In the terminologia anatomica such a ligament does not exist. In fact, this also holds for the long (dorsal SI) ligament, reflecting one of the problems of topographical anatomy.
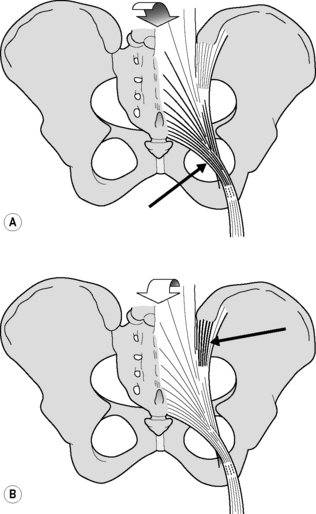
Figure 2.2.6 • (A) Nutation winds up the sacrotuberous ligament. (B) Counternutation winds up the long dorsal sacroiliac ligament
Sacrotuberous ligaments
In embalmed human specimens, we could demonstrate a direct relation between nutation and tension of the sacrotuberous ligament (Figure 2.2.6A). By straining this ligament, we found a decrease of nutation (Vleeming et al. 1989a, 1989b), indicating that these ligaments are well-suited to restrict nutation. It can be expected that the opposite (diminished ligament tension) will increase nutation.
Long dorsal sacroiliac ligaments
In view of the capability of the sacrotuberous ligaments to restrict nutation, we wondered which ligament(s) could restrict counternutation. Because of its connection to the PSIS and to the lateral part of the sacrum (Figure 2.2.6B), we expected that the long dorsal SI ligament could fulfil this function. The ligament can be easily palpated in the area directly caudal to the PSIS and is of special interest since women complaining of lumbopelvic back pain during pregnancy frequently experience pain within the boundaries of this ligament (Mens et al. 1992, Njoo 1996, Vleeming et al. 1996). Pain in this area is also not uncommon in men. The ligament is the most superficially located SIJ ligament and therefore well-suited to mirror asymmetric stress in the SIJ. As this ligament is not well known in medical practice, we will summarize data from an anatomical and biomechanical study (Vleeming et al. 1996) that assessed the function of the ligament by measuring its tension during incremental loading of biomechanically relevant structures.
Biomechanical aspects
This observation implies that the tension of the long ligament can be altered by displacement in the SIJ as well as by action of various muscles. Obviously, nutation in the SIJ induces relaxation of the long ligament, whereas counternutation increases tension. This is in contrast to the effect on the sacrotuberous ligament (see Figure 2.2.5). Increased tension in the sacrotuberous ligament during nutation can be due to SIJ movement itself as well as to increased tension of the biceps femoris and/or gluteus maximus muscle. This mechanism can help to control nutation. As counternutation increases tension in the long ligament, this ligament can assist in controlling counternutation (see Figure 2.2.6).
A comparable complex relation might hold for the long ligament and the erector spinae, or more specifically the multifidus muscle. As the multifidus is connected to the sacrum (MacIntosh & Bogduk 1986, 1991; see Chapter 1), its action induces nutation. As a result, the long ligament will slacken. However, the present study shows an increase in tension in the long ligament after traction to the erector spinae muscle. This counterbalancing effect is due to the connections between the erector spinae muscle and the long ligament, and opposes the slackening. In vivo, this effect might be smaller because the moment of force acting on the sacrum is raised by the pull of the erector spinae muscle and the resulting compression force on the spine (Snijders et al. 1993b). This spinal compression was not applied in this study. Both antagonistic mechanisms – between long and sacrotuberous ligaments and between the long ligament and the erector spinae muscle – might serve to preclude extensive slackening of the long ligament. Such mechanisms could be essential for a relatively flat joint such as the SIJ, which is susceptible to shear forces (Snijders et al. 1993a, 1993b). It can be safely assumed that impairment of a part of this interconnected ligament system will have serious implications for the joint as load transfer from spine to hips and vice versa is primarily transferred via the SIJ (Snijders et al. 1993a, 1993b).
As shown earlier (Vleeming et al. 1996), traction to the biceps femoris tendon hardly influences tension of the long ligament. This is in contrast to the effect of the biceps on the sacrotuberous ligament (Vleeming et al. 1989a, 1989b, van Wingerden et al. 1993). The observations might well be related to the spiralling of the sacrotuberous ligament. Most medial fibres of the ligament tend to attach to the cranial part of the sacrum, whereas most fibres arising from a lateral part of the ischial tuberosity tend to attach to the caudal part of the sacrum (see Figure 2.2.6A). The fibres of the biceps tendon, which approach a relatively lateral part of the ischial tuberosity, pass mainly to the caudal part of the sacrum. As a consequence, the effect of traction to the biceps femoris on the tension of the long ligament can only be limited.
The effect on the long ligament of loading the posterior layer of the thoracolumbar fascia depends on the direction of the forces applied. Artificial traction to the fascia mimicking the action of the transverse abdominal muscle has no effect. Traction in a craniolateral direction, mimicking the action of the latissimus dorsi muscle, results in a significant decrease in tension in the ipsilateral and contralateral long ligaments. As shown in another study (Vleeming et al. 1995), traction to the latissimus dorsi influences the tension in the posterior layer of the thoracolumbar fascia, ipsilaterally as well as contralaterally, especially below the level of L4. Thus slackening of the long ligament could be the result of increased tension in the posterior layer by the latissimus dorsi. This might itself lead to a slight nutation, leading to more compression and force closure of the SIJ. As shown in this study, slackening of the long ligament can also occur due to action of the gluteus maximus muscle, which is ideally suited to compress the SIJ.
It is inviting to draw conclusions when palpation, directly caudal to the PSIS, is painful. However, pain in this area might be due to pain referred from the SIJ itself (Fortin et al. 1994a, 1994b), but also due to counternutation in the SIJ. Counternutation is part of a pattern of flattening the lumbar spine (Egund et al. 1978, Lavignolle et al. 1983, Sturesson et al. 1989) that occurs in particular late in pregnancy when women counterbalance the weight of the fetus (Snijders et al. 1976). However, such a posture combined with counternutation could also result from a pain-withdrawal reaction to impairment elsewhere in the system. Hence, only specific pain within the boundaries of the long ligament can be used as a diagnostic criterion (Vleeming et al. 2002). An example of a pain-withdrawal reaction could be the following: pain of the pubic symphysis following delivery (Mens et al. 1992) could preclude normal lumbar lordosis and hence nutation owing to pain of an irritated symphysis. After all, lumbar lordosis leads to nutation in the SIJ (Weisl 1955, Egund et al. 1978, Lavignolle et al. 1983, Sturesson et al. 1989). Nutation implies that the left and right PSISs approach each other slightly while the pubic symphysis is caudally extended and cranially compressed (Lavignolle et al. 1983, Walheim & Selvik 1984). In this example the patient will avoid nutation and flattens the lower spine, leading to sustained tension and pain in the long ligament. In a study by Mens et al. (1999), it was shown that a positive active straight leg raise test coincides with a counternutated position of the SIJ in many patients.
Before focusing on the role of the muscles, we will draw attention to the thoracolumbar fascia.
The role of the thoracolumbar fascia in stabilizing the lumbopelvic area
To deepen our knowledge of the role of the thoracolumbar fascia the posterior layer of the thoracolumbar fascia was loaded by simulating the action of various muscles (Vleeming et al. 1995).
Anatomical aspects
The posterior layer of the thoracolumbar fascia covers the back muscles from the sacral region, through the thoracic region as far as the fascia nuchae. At the level of L4–L5 and the sacrum, strong connections exist between the superficial and deep lamina. The transverse abdominal and internal oblique muscles are indirectly attached to the thoracolumbar fascia through a dense raphe formed by fusion of the middle layer (Bogduk & MacIntosh 1984) of the thoracolumbar fascia and both laminae of the posterior layer. This ‘lateral raphe’ (Bogduk & MacIntosh 1984, Bogduk & Twomey 1987) is localized laterally to the erector spinae and cranial to the iliac crest.
Superficial lamina (Figure 2.2.7)
Barker & Briggs (1999) showed that the superficial lamina is also continuous superiorly with the rhomboids. They could not confirm the findings of Bogduk et al. (1998) in relation to thickening of the fascia and the presence of posterior accessory ligaments. This is in agreement with the findings of Vleeming et al. (1995).
Barker & Briggs (1999) showed that the deep lamina (Figure 2.2.8) is continuous cranially with the tendons of the splenius cervicis and capitis muscles. At lower lumbar and sacral levels, the fibres of the deep lamina are orientated from craniomedial to caudolateral. At sacral levels, these fibres are fused with those of the superficial lamina. As, in this region, fibres of the deep lamina are continuous with the sacrotuberous ligament, an indirect link exists between this ligament and the superficial lamina. There is also a direct connection with some fibres of the deep lamina. In the pelvic region, the deep lamina is connected to the PSIS, iliac crests and long dorsal SI ligament. This ligament originates from the sacrum and attaches to the PSIS. In the lumbar region, fibres of the deep lamina derive from the interspinous ligaments. They attach to the iliac crest and more cranially to the lateral raphe, to which the internal oblique is attached. In some specimens, fibres of the deep lamina cross to the contralateral side between L5 and S1. In the depression between the median sacral crest and the posterior superior and inferior iliac spines, fibres of the deep lamina fuse with the fascia of the erector. More cranially, in the lumbar region, the deep lamina becomes thinner and freely mobile over the back muscles. In the lower thoracic region, fibres of the serratus posterior inferior muscle and its fascia fuse with fibres of the deep lamina.
Biomechanical aspects
Traction to the superficial lamina
Depending on the site of the traction, quite different results were obtained (Vleeming et al. 1995). Traction to the cranial fascia and muscle fibres of the latissimus dorsi muscle showed limited displacement of the superficial lamina (homolaterally up to 2–4 cm). Traction to the caudal part of the latissimus dorsi caused displacement up to the midline. This midline area is 8–10 cm removed from the site of traction. Between L4–L5 and S1–S2, displacement of the superficial lamina occurred even contralaterally. Traction to the gluteus maximus also caused displacement up to the contralateral side. The distance between the site of traction and visible displacement varied from 4 to 7 cm. The effect of traction to the external oblique muscle varied markedly between the different preparations. In all preparations, traction to the trapezius muscle resulted in a relatively small effect (up to 2 cm).
Traction to the deep lamina
Barker & Briggs (1999) make the interesting comment that the posterior layer is ideally positioned to receive feedback from multiple structures involved in lumbar movements and may regulate ligamentous tension via its extensive muscular attachments to both deep stabilizing and more superficial muscles. They also report that the fascia displays viscoelastic properties and thus is capable of altering its structure to adapt to the stresses placed on it. The posterior layer has been reported to stiffen with successive loading and adaptive fascial thickening is possible.
Barker & Briggs (1999) also comment that when adaptive strengthening of the posterior layer takes place, one might expect to facilitate this by using exercises that strengthen its attaching muscles, both deep and superficial. Adaptive strengthening therefore would be expected to occur with exercises using contralateral limbs such as swimming and walking and torsional training. It also might occur with recovery of muscle bulk and function (erector spinae/multifidus) during lumbopelvic stabilization exercises.
Bogduk et al. (1998) do not agree with the concept that the latissimus dorsi has a role in rotating the spine and comment that the muscle is designed to move the upper limb and its possible contribution to bracing the SIJ via the thoracolumbal fascia is trivial. In contrast to this, Kumar et al. (1996) showed that axial rotation of the trunk involves agonistic activity of the contralateral external obliques, and ipsilateral erector spinae and latissimus dorsi as agonistic muscles to rotate the trunk.
Mooney et al. (2001) used the anatomical relation of the latissimus dorsi and the contralateral gluteus maximus muscles to study their coupled effect during axial rotation exercises and walking. They concluded that in normal individuals, walking a treadmill, the functional relationship between the mentioned muscles could be confirmed. It was apparent that the right gluteus maximus muscle had on average a lower signal amplitude compared to the left (n = 15; 12 right-handed). This reciprocal relationship of muscles correlates with normal reverse rotation of shoulders versus the pelvis in normal gait. They showed that during right rotation of the trunk the right latissimus dorsi muscle is significantly more active than the left, but that the left gluteus maximus muscle is more active than the right. In patients with SIJ problems a strikingly different pattern was noticed. On the symptomatic side the gluteus maximus was far more active compared with the healthy subjects. The reciprocal relation between latissimus and gluteus maximus muscles, however, was still present. After an intense rotational strengthening training programme, the patients showed a marked increase in latissimus dorsi muscle strength and diminished activity of the gluteus muscle on the symptomatic side.
The importance of these findings could be that rotational trunk muscle training is important, particularly for stabilizing the SIJ and lower spine. These findings contradict the conclusion of Bogduk et al. (1998) that the latissimus dorsi muscle has no function besides upper limb movement.
Pelvic girdle pain can be partially relieved by application of a pelvic belt, a device that ‘self-braces’ the SIJ (Vleeming et al. 1992b). By exerting compression on the lower lumbar spine and pelvis, the posterior layer of the thoracolumbar fascia and its attached muscles can accomplish self-bracing physiologically. It is noteworthy that, as shown in this study, the coupled function of the gluteus maximus and the contralateral latissimus dorsi muscles creates a force perpendicular to the SIJ.
Attention is drawn to a possible role of the erector muscle and multifidus in load transfer. Between the lateral raphe and the interspinous ligaments, the deep lamina encloses the erector muscle and multifidus. It can be expected that contraction of the erector/multifidus will longitudinally increase the tension in the deep lamina. In addition, the whole posterior layer of the thoracolumbar fascia will be ‘inflated’ by contraction of the erector spinae/multifidus (Vleeming et al. 1995), comparable to pumping up a ball. Consequently, it can be assumed that the training of muscles such as the gluteus maximus, latissimus dorsi and erector spinae, and the multifidus can assist in increasing force closure also by strengthening the posterior layer of the thoracolumbar fascia.
Muscles and self-bracing
Various muscles are involved in force closure of the SIJ. With respect to SIJ function, we focus here on four muscles: the erector spinae/multifidus, gluteus maximus, latissimus dorsi and biceps femoris (Figure 2.2.9).
The erector spinae/multifidus is the pivotal muscle that loads and extends spine and pelvis. The sacral connections of the erector/multifidus induce nutation in the SIJ, tensing ligaments such as the interosseous, sacrotuberous and sacrospinal. Nutation is anticipatory for preloading the SIJ (preparatory movement; Hodges et al. 2003 and see above). These muscles have a double function because their iliac connections pull the posterior sides of the iliac bones towards each other, constraining nutation. This implies that during nutation, due to action of the erector spinae/multifidus, the cranial side of the SIJ tends to be compressed whereas the caudal side has a tendency to widen. The latter is restricted by the sacrotuberous ligament, tensed by nutation, which has direct fascial connections with the erector spinae. A comparable process will occur in the pubic symphysis where the largest symphyseal ligament passes caudally to the joint (see Figure 2.2.2).
Owing to its perpendicular orientation to the SIJ, the gluteus maximus can compress the joints directly and also indirectly by its vast muscular connections with the sacrotuberous ligament. Gibbons (2004) speculates that the caudal part of the gluteus maximus, originating from the sacrotuberous ligament, can function in conjunction with the pelvic floor. Vleeming et al. (1995) noted in a study on the SIJ that compression, among others, also can be established by coupling of the gluteus maximus with the contralateral latissimus dorsi muscle via the thoracolumbar fascia (Figure 2.2.9).
Tension in the sacrotuberous ligament is increased by caudal traction to the long head of the biceps femoris. This is possible because not all fibres of the long head of the biceps attach to the ischial tuberosity; partly, and occasionally completely, its proximal tendon is continuous with the sacrotuberous ligament (Figure 2.2.9). This tension mechanism of the biceps femoris depends on body position (van Wingerden et al. 1993). In most specimens, a higher percentage of force was transferred from the biceps to the sacrotuberous ligament in a flexed, stooped position than in an erect stance. This could be expected because the flexion torque on the lumbar spine increases when changing from an erect to a flexed stance. Consequently, in stooped positions, larger compression forces are needed to prevent the sacrum from tilting forward. This force can be derived in part from the biceps femoris but also from other muscles attached to the sacrotuberous ligament (the sacral part of the aponeurosis of the erector spinae and gluteus maximus). Barker (2005) showed that besides the biceps muscle also part of the semimembranosus muscle regularly is connected to the sacrotuberous ligament. In her studies, Barker confirmed the biceps connection with the sacrotuberous ligament.
Comparable to the erector muscle, a double function of the hamstrings (including the biceps femoris muscle) can be described. Particularly in stooped positions and in sitting with straight legs, sitting upright, the hamstrings are well positioned to rotate the iliac bones posteriorly relative to the sacrum. This nutating effect (Sturesson et al. 1989) can be constrained by the biceps femoris with its connections to the sacrotuberous ligament. Nutation in stooped positions can help to avoid excessive loading of the posterior part of the lumbar discs.
Self-bracing during forward bending
When stooped, the sacrum assumes a more or less horizontal position. When lifting objects in this posture, the vertical force from the upper part of the body and the object acts almost perpendicularly to the longitudinal axis of the sacrum. In this situation, the joint also becomes loaded in the transverse plane and stability will depend on effective compression of the SIJ in a transverse plane. The transverse diameter is small in comparison with the longitudinal diameter, so additional forces are needed to protect the SIJ. An electromyography (EMG) study found that, during lifting, the activity of the gluteus maximus muscle paralleled that of latissimus dorsi and erector spinae muscles (Noe et al. 1992). These observations indicate that, in this position, self-bracing of the pelvis can be established by contraction of the mentioned muscles and core muscles like the transversus, multifidus, the pelvic floor and the diaphragm (Hodges et al. 2003).
Self-bracing in unconstrained positions
The question of how the SIJ can be stabilized in unconstrained sitting and standing led to the following experiment: we expected that during unconstrained sitting, especially the oblique abdominals would be active to self-brace the pelvis, to deliver the necessary compression force. Using electromyography (EMG) the abdominal muscle activity was recorded in different positions (supine, unconstrained standing, and sitting with and without crossed legs on an office chair with the use of a backrest and armrests). For both the external and internal obliques, the activity was significantly higher in standing than in sitting. The activity of particularly the internal obliques turned out to be significantly higher in sitting than in a supine position (Figure 2.2.10). Surprisingly, the activity of the oblique abdominals was lowered by crossing the legs (Snijders et al. 1995; cross-legged or ankle on knee as preferred by the individual; Figure 2.2.10). By contrast, the activity of the rectus abdominis was not altered by leg-crossing. We concluded that to stabilize the pelvis: (1) unconstrained sitting and standing initiates an oblique ventral muscle–tendon sling; and (2) leg-crossing is a functional habit. Crossing the legs causes rotation in the pelvis and possibly tenses the thoracolumbar fascia. Due to creep of tissues (elongation), leg-crossing is only temporarily functional; as a consequence the legs are crossed to the other side (Snijders et al. 1995).
Failed self-bracing
One method to facilitate pelvic stability and reduce pain is the use of a pelvic belt (Mens et al. 1996). In a loading experiment on embalmed human preparations, nutation of the sacrum decreased by about 20% when applying a belt force of only 50 N. Based on the biomechanical model presented here, such a belt must be applied with a small force, like the laces of a shoe. This will be sufficient to generate a self-bracing effect in the SIJ under heavy load (Vleeming et al. 1992a, 1992b, Snijders et al. 1993a, 1993b). The model indicates that the belt must be positioned just cranial to the greater trochanters; it crosses the SIJ caudally, and can assist in preventing gapping of the caudal part of the SIJ. However, this is not what we propose to our patients. Only in very severe cases do patients wear belts for a short period in the beginning and during training.
We like to emphasize that, according to the model presented here, weakening of the erector spinae/multifidus (insufficient nutation) and the gluteus maximus muscles (insufficient ligament pull and SIJ compression) will lead to diminished straining of the sacrotuberous ligament. Weakness of these muscles has in addition implications for the strength of the thoracolumbar fascia, especially if combined with a weak latissimus dorsi muscle. Weakening or inadequate use of the transversus abdominis muscle precludes, among others, sufficient tension of the thoracolumbar fascia. This leads to diminished self-bracing of the SIJ. With insufficient bracing, the body can be expected to use other strategies, for example tensing the sacrotuberous ligament through activation of the biceps femoris or exaggerated contraction of parts of the gluteus maximus. Although definitive experimental data are lacking, we presume that tension of the biceps and other hamstring muscles can be increased over an extended period. Hungerford et al. (2003) showed altered firing patterns of these muscles in SIJ patients. Higher tension of the hamstrings will force the pelvis to rotate backwards, leading to flattening of the lumbar spine. The biceps femoris muscle, in particular, will strain the sacrotuberous ligament, diminishing nutation. Both processes will be harmful if hamstring tension is continuously increased because, in this relatively counternutated position, load will be unnaturally distributed to the lower lumbar discs. In our opinion, it is important to realize that counternutation of the SIJ seems to be a pain-withdrawal reaction that disengages the normal self-bracing of the pelvis. The patient seeks to compress the SIJ in a new joint position, with less adequate and strong ligaments to be tensed. Hence, the lower spine can become unstable and prone to infringement, leading to LBP.
We tried to define a simple exercise that could assist in preventing LBP and counteract the detrimental effects of modern life. The exercise shown in Figure 2.2.11 facilitates nutation of the SIJ and couples the action of biceps femoris, erector spinae and gluteus maximus. We suppose this to be an effective and natural way to stretch the hamstring. To evaluate its effectiveness, the exercise needs further practical study. This is an exercise that has to be demonstrated carefully to patients with the main movement occurring in the hip joints with a relaxed spine. In case of lumbar impairments, of course, care has to be taken with this exercise.
Optimal and non-optimal pelvic girdle stability
Since the mid-1990s, several clinicians and researchers have studied PGP and its causes. In the past, PGP research focused on the functional and clinical analysis, mainly in women; this was a consequence of former publications emphasizing relaxation of the pelvic girdle as a primary cause for instability of the pelvic ring. By the 1870s, Snelling was of the impression that relaxation of the pelvic articulations becomes apparent either suddenly after parturition or gradually during pregnancy, permitting mobility which hinders locomotion and gives rise to distressing and alarming sensations (in Svensson et al. 1990).
Abramson et al. (1934) described pelvic pain and instability and made the distinction between symptoms related to the pubic joint, to the SIJ, or to a combination. They also described pain in the symphyseal region with radiation to the thighs. The SIJ symptoms are presented as low (lowest) backache and localized pain in the SIJ. Furthermore, they noted a waddling gait and a positive Trendelenburg sign (during walking the patient shows inability to hold the pelvis in the horizontal plane). The authors used, among others, screening techniques like X-rays of the pubic symphysis, even in women who were 8 months pregnant (Abramson et al. 1934). So before the hazards of X-rays were realized, increased mobility and widening of the symphysis in relation to PGP were well-documented. Postmortem studies in former days, when mortality during pregnancy and labor was not exceptional, showed increased mobility of the SIJ and an increased amount of synovial SIJ and symphyseal fluid in pregnant women (Brooke 1924).
After these studies on pelvic instability in the nineteenth and twentieth centuries, analysis of pelvic pain shifted towards a more functional approach. By contrast, the study of lumbar pain after Mixter and Barr’s (1934) study (which explained that radiating pain in the leg is the consequence of a rupture of the intervertebral disc) has been particularly addressed to the study of pain generators that could generate the symptoms. Since the 1980s there have been increasing efforts to study the lumbar and pelvic area as an integrated part of a complex kinematic system.
More recent functional pelvic studies have stated that PGP can be related to non-optimal stability, which is underpinned by the fact that a pelvic belt reduces pain symptoms and influences the laxity of the pelvic ring. Vleeming et al. (1992b) conclude that pelvic belts enhance pelvic stability because they reduce SIJ laxity. Mens et al. (1999) suggested that, after initial provocative pelvic tests, these tests should be repeated with application of a pelvic belt to assess possible differences. In these studies, only a small amount of tension (50 N) is applied to the belt, just above the greater trochanter. Larger forces did not yield better results, as predicted in a biomechanical study (Vleeming et al. 1990a, 1990b). The efficacy of the pelvic belt was primarily determined by the location of the belt and the effects were explained by increased compression of the SIJ.
Buyruk et al. (1995a, 1995b, 1999) applied unilateral oscillations to the anterior-superior iliac spine to assess laxity of the pelvic joints. With sonoelasticity, using Doppler imaging of vibrations (DIV), they measured the stiffness/laxity ratio of artificially unstabilized SIJ in comparison with stabilized pelvices. The new method was objective and repeatable. In vivo studies have used the same technology in healthy subjects (Damen et al. 2002a, 2002b). It was shown that pelvic belts are effective to alter the laxity of the SIJ with an applied force of the pelvic belt of maximally 50 N. Subsequently, they showed that the laxity values of the SIJ decreased after application of a pelvic belt in patients with pelvic pain (Damen et al. 2002a). In another study (Damen et al. 2002b), patients with asymmetric SIJ laxity reported significantly more pain during pregnancy when compared with patients with symmetric laxity. According to the authors, increased general laxity is not associated with pelvic pain. Pregnant women with moderate or severe pelvic pain have the same laxity of the SIJ as pregnant women with no or mild pain. According to these studies, the asymmetry of laxity correlates with the symptomatic individual. In keeping with these data, perhaps manual motion testing should focus more on the (a)symmetry of SIJ motion. However, up to now, there has been no evidence that manual motion tests have a sufficient level of intra- and inter-tester reliability.
In 1999, Mens et al. developed a new diagnostic test. They studied the relation between impaired active straight leg raising (ASLR) and the mobility of pelvic joints with and without the application of a pelvic belt (testing the hypothesis that the pelvis is the basic bony platform that has to be stabilized before levers, like the legs and spine, can be used effectively). They conclude that impairment of the ASLR test correlates highly with the level of laxity of the pelvis, because application of a pelvic belt generally reduces the impairment of the ASLR test. The sensitivity and specificity of the test is high for PGP and the test is suitable to discriminate between PGP patients and healthy individuals (Mens et al. 1999).
The same authors (Mens et al. 1999) showed – by means of X-rays taken after pregnancy – that the pubic bone on the symptomatic side shifts caudally relative to the other side when the symptomatic leg is freely hanging down in a standing position. This procedure differs from the classical Chamberlain X-ray method, which screens the symptomatic loaded side. The authors conclude that this symphyseal shift is the result of an anterior rotation of the iliac bone relative to the sacrum on the symptomatic side (counternutation in the SIJ).
Hungerford et al. (2001) came to the same conclusion. Using an external motion analysis system, they studied (three-dimensionally) the angular and translational displacements in patients with SIJ problems and in healthy persons. They concluded that posterior rotation of the iliac bones relative to the sacrum (nutation) occurs in normals on the weightbearing side. By contrast, the iliac bones rotated anteriorly relative to the sacrum (counternutation; see Figure 2.2.6B) in the patient group. They found the same in the standing flexion test. Only on the loaded (standing) symptomatic side did an anterior rotation of the iliac bone occur.
The conclusion of these recent studies is that a relation exists between pelvic asymmetric laxity and the severity of complaints (Buyruk et al. 1999, Damen et al. 2002b). Damen et al. state that subjects with asymmetric laxity of the SIJ during pregnancy have a threefold higher risk of moderate to severe pelvic pain persisting into the postpartum period, compared to subjects with symmetric laxity during pregnancy. They also conclude that pelvic belt application can diminish the laxity and stiffen the pelvis and influence an impaired ASLR test with application of the DIV method. Based on the studies mentioned here, a dysfunctional SIJ is normally not related to a subluxated position of the joint, but to an altered position within the normal range of motion due to asymmetric forces acting on the joint.
We state that knowledge of the coupling mechanisms between spine, pelvis, legs and arms is essential to understand dysfunction of the human locomotor system, particularly the lower back. It has led us to describe three muscle slings (one longitudinal and two oblique) that can be energized (Figure 2.2.12).
Abramson D., Roberts S.M., Wilson P.D. Relaxation of the pelvic joints in pregnancy. Surg. Gynecol. Obstet.. 1934;58:595-613.
Adams M.A., Dolan P., Burton K., Bodguk N. The biomechanics of back pain. Edinburgh: Churchill Livingstone; 2002.
Ahn A.C., Tewari M., Poon C.S., Phillips R.S. The limits of reductionism in medicine: could systems biology offer an alternative? PLoS Med.. 2006;3(6):e208.
Bakland O., Hansen J.H. The axial sacroiliac joint. Anat. Clin.. 1984;6:29-36.
Barker J. The thoralumbar fascia. Thesis. Australia: University of Melbourne; 2005.
Barker J., Briggs C.A. Attachments of the posterior layer of the lumbar fascia. Spine. 1999;24(17):1757-1764.
Bogduk N., MacIntosh J.E. The applied anatomy of the thoracolumbar fascia. Spine. 1984;9(2):164-170.
Bogduk N., Twomey L.T. Clinical anatomy of the lumbar spine. Melbourne: Churchill Livingstone; 1987.
Bogduk N., Johnson G., Spalding D. The morphology and biomechanics of the latissimus dorsi. Clin. Biomech.. 1998;13:377-385.
Bowen V., Cassidy J.D. Macroscopic and microscopic anatomy of the sacroiliac joints from embryonic life until the eighth decade. Spine. 1981;6:620.
Brooke R. The sacroiliac joint. J. Anat.. 1924;58:299-305.
Buyruk H.M., Stam H.J., Snijders C.J., et al. The use of colour Doppler imaging for the assessment of sacroiliac joint stiffness: a study on embalmed human pelvises. Eur. J. Radiol.. 1995;21:112-116.
Buyruk H.M., Snijders C.J., Vleeming A., et al. The measurements of sacroiliac joint stiffness with colour Doppler imaging: a study on healthy subjects. Eur. J. Radiol.. 1995;21:117-121.
Buyruk H.M., Stam H.J., Snijders C.J., et al. Measurement of sacroiliac joint stiffness in peripartum pelvic pain patients with Doppler imaging of vibrations (DIV). Eur. J. Obstet. Gynecol. Reprod. Biol.. 1999;83(2):159-163.
Cholewicki J., Panjabi M.M., Khachatryan A. Stabilizing function of trunk flexor-extensor muscles around a neutral spine posture. Spine. 1997;22(19):2207-2212.
Cholewicki J., Simons A.P., Radebold A. Effects of external trunk loads on lumbar spine stability. J. Biomech.. 2000;33(11):1377-1385.
Crisco J.J., Panjabi M.M., Yamamoto I., Oxland T.R. Euler stability of the human ligamentous lumbar spine. Part II: experiment. Clin. Biomech.. 1992;7(1):27-32.
Damen L., Spoor C.W., Snijders C.J., Stam H.J. Does a pelvic belt influence sacroiliac laxity? Clin. Biomech.. 2002;17(7):495-498.
Damen L., Buyruk H.M., Guler Uysal F., et al. The prognostic value of asymmetric laxity of the sacroiliac joint in pregnancy related pelvic pain. Spine. 2002;27(24):2820-2824.
Dijkstra P.F., Vleeming A., Stoeckart R. Complex motion tomography of the sacroiliac joint: an anatomical and ro entgenological study. Fortschr. Geb. Rontgenstr. Nuklearmed.. 1989;150:635-642.
Egund N., Ollson T.H., Schmid H., Selvik G. Movements in the sacroiliac joints demonstrated with roentgen stereophotogrammetry. Acta Radiol. Diagn.. 1978;19:833.
Fortin J.D., Dwyer A.P., West S., Pier J. Sacroiliac joint: pain referral maps upon applying a new injection/arthrography technique. 1: Asymptomatic volunteers. Spine. 1994;19:1475-1482.
Fortin J.D., Aprill C.N., Ponthieux B., Pier J. Sacroiliac joint: pain referral maps upon applying a new injection/arthrography technique. 2: Clinical evaluation. Spine. 1994;19:1483-1489.
Gibbons S. The caudomedial part of the gluteus maximus and its relation to the sacrotuberous ligament. Fifth Interdisciplinary World Congress on Low Back Pain. 2004. Melbourne, Australia
Hodges P.W., Cholewicki J. Functional Control of the Spine. In: Vleeming A., Mooney V., Stoeckart R., editors. Movement, Stability and Lumbopelvic Pain. Elsevier; 2007:489-512.
Hodges P.W., Kaigle A., Holm S., et al. Intervertebral stiffness of the spine is increased by evoked contraction of transversus abdominus and the diaphragm: in vivo porcine studies. Spine. 2003;28(23):2594-2601.
Hungerford B., Gilleard W., Lee D. Alteration of sacroiliac joint motion patterns in subjects with pelvic motion asymmetry. Proceedings from the Fourth World Interdisciplinary Congress on Low Back and Pelvic Pain. Montreal, Canada. 2001.
Hungerford B., Gilleard W., Hodges P.W. Evidence of altered lumbo–pelvic muscle recruitment in the presence of sacroiliac joint pain. Spine. 2003;28(14):1593-1600.
Huson A. Kinematic models and the human pelvis. In: Vleeming A., et al, editors. Movement stability and low back pain. Edinburgh: Churchill Livingstone; 1997:123-131.
Kumar S., Narayan B.S., Zedka M. An electromyographic study of unresisted trunk rotation with normal velocity among healthy subjects. Spine. 1996;21(13):1500-1512.
Lavignolle B., Vital J.M., Senegas J., et al. An approach to the functional anatomy of the sacroiliac joints in vivo. Anat. Clin.. 1983;5:169.
Lovejoy C.O. Evolution of human walking. Sci. Am.. 1988;259:118-125.
MacIntosh J.E., Bogduk N. The biomechanics of the lumbar multifidus. Clin. Biomech.. 1986;1:205-213.
MacIntosh J.E., Bogduk N. The attachments of the lumbar erector. Spine. 1991;16:783-792.
Mens J.M.A., Stam H.J., Stoeckart R., et al. Peripartum pelvic pain: a report of the analysis of an inquiry among patients of a Dutch patient society. Vleeming A., et al, editors, First Interdisciplinary World Congress on Low Back Pain and its Relation to the Sacroiliac Joint. 1992:521-533. San Diego, CA, 5–6 November
Mens J.M.A., Vleeming A., Stoeckart R., et al. Understanding peripartum pelvic pain: implications of a patient survey. Spine. 1996;21(11):1303-1369.
Mens J.M.A., Vleeming A., Snijders C.J., et al. The active straight leg raising test and mobility of the pelvic joints. Eur. Spine J.. 1999;8:468-473.
Miller J.A., Schultz A.B., Andersson G.B. Load displacement behavior of sacroiliac joint. J. Orthop. Res.. 1987;5:92.
Mixter W.J., Barr J.S. Rupture of the intervertebral disc with involvement of the spinal canal. N. Engl. J. Med.. 1934;211:210-215.
Mooney V., Pozos R., Vleeming A., et al. Exercise treatment for sacroiliac joint pain. Orthopedics. 2001;24(1):29-32.
Moseley G.L. Reconceptualising pain according to modern pain science. Phys. Ther. Rev.. 2007;12(3):169-178.
Njoo K.H. Nonspecific LBP in general practice: a delicate point. Thesis. Rotterdam: Erasmus University; 1996.
Noe D.A., Mostardi R.A., Jackson M.E., et al. Myoelectric activity and sequencing of selected trunk muscles during isokinetic lifting. Spine. 1992;17(2):225.
Pool-Goudzwaard A., Hoek van Dijke G., Mulder P., et al. The iliolumbar ligament: its influence on stability of the sacroiliac joint. Clin. Biomech.. 2003;18(2):99-105.
Reeves N.P., Cholewicki J. Modeling the human lumbar spine for assessing spinal loads, stability, and risk of injury. [Review] [238 refs]. Crit. Rev. Biomed. Eng.. 2003;31;(1–2):73-139.
Reeves N.P., Narendra K.S., Cholewicki J. Spine stability: the six blind men and the elephant. [Review] [58 refs]. Clin. Biomech.. 2007;22;(3):266-274.
Salsabili N., Valojerdy M.R., Hogg D.A. Variations in thickness of articular cartilage in the human sacroiliac joint. Clin. Anat.. 1995;8:388-390.
Sashin D. A critical analysis of the anatomy and the pathological changes of the sacroiliac joints. J. Bone Joint Surg.. 1930;12:891.
Solonen K.A. The sacroiliac joint in the light of anatomical, roentgenological and clinical studies. Acta Orthop. Scand.. 1957;27:1-127.
Snijders C.J., Seroo J.M., Snijder J.G.N., Hoedt H.T. Change in form of the spine as a consequence of pregnancy. Digest of the Eleventh International Conference on Medical and Biological Engineering. 1976:670-671. May 1976, Ottawa, Canada
Snijders C.J., Vleeming A., Stoeckart R. Transfer of lumbosacral load to iliac bones and legs. 1: Biomechanics of self-bracing of the sacroiliac joints and its significance for treatment and exercise. Clin. Biomech.. 1993;8:285-294.
Snijders C.J., Vleeming A., Stoeckart R. Transfer of lumbosacral load to iliac bones and legs. 2: Loading of the sacroiliac joints when lifting in a stooped posture. Clin. Biomech.. 1993;8:295-301.
Snijders C.J., Slagter A.H.E., Strik van R., et al. Why leg-crossing? The influence of common postures on abdominal muscle activity. Spine. 1995;20(18):1989-1993.
Stewart T.D. Pathologic changes in aging sacroiliac joints. Clin. Orthop. Relat. Res.. 1984;183:188.
Sturesson B., Selvik G., Udén A. Movements of the sacroiliac joints. A roentgen stereophotogrammetric analysis. Spine. 1989;14:162-165.
Sturesson B., Udén A., Vleeming A. A radiostereometric analysis of movements of the sacroiliac joints during the standing hip flexion test. Spine. 2000;25(3):364-368.
Sturesson B., Udén A., Vleeming A. A radiostereometric analysis of the movements of the sacroiliac joints in the reciprocal straddle position. Spine. 2000;25(2):214-217.
Svensson H.O., Andersson G.B.J., Hagstad A., Jansson P.O. The relationship of low-back pain to pregnancy and gynaecologic factors. Spine. 1990;15:371-375.
van Wingerden J.P., Vleeming A., Snijders C.J., Stoeckart R. A functional–anatomical approach to the spine–pelvis mechanism: interaction between the biceps femoris muscle and the sacrotuberous ligament. Eur. Spine J.. 1993;2:140-144.
Vleeming A., Stoeckart R. The role of the pelvic girdle in coupling the spine and the legs: a clinical-anatomical perspective on pelvic stability. In: Vleeming A., Mooney V., Stoeckart R., editors. Movement, Stability and Lumbopelvic Pain. Elsevier; 2007:113-137.
Vleeming A., Stoeckart R., Snijders C.J. The sacrotuberous ligament: a conceptual approach to its dynamic role in stabilizing the sacroiliac joint. Clin. Biomech.. 1989;4:201-203.
Vleeming A., Wingerden van J.P., Snijders C.J., et al. Load application to the sacrotuberous ligament: influences on sacroiliac joint mechanics. Clin. Biomech.. 1989;4:204-209.
Vleeming A., Stoeckart R., Volkers A.C.W., Snijders C.J. Relation between form and function in the sacroiliac joint. 1: Clinical anatomical aspects. Spine. 1990;15:130-132.
Vleeming A., Volkers A.C.W., Snijders C.J., Stoeckart R. Relation between form and function in the sacroiliac joint. 2: Biomechanical aspects. Spine. 1990;15(2):133-136.
Vleeming A., Wingerden van J.P., Dijkstra P.F., et al. Mobility in the SI-joints in old people: a kinematic and radiologic study. Clin. Biomech.. 1992;7:170-176.
Vleeming A., Buyruk H.M., Stoeckart R., et al. An integrated therapy for peripartum pelvic instability. Am. J. Obstet. Gynecol.. 1992;166(4):1243-1247.
Vleeming A., Pool-Goudzwaard A.L., Stoeckart R., et al. The posterior layer of the thoracolumbar fascia: its function in load transfer from spine to legs. Spine. 1995;20:753-758.
Vleeming A., Pool-Goudzwaard A., Hammudoghlu D., et al. The function of the long dorsal sacroiliac ligament: its implication for understanding LBP. Spine. 1996;21(5):556-562.
Vleeming A., de Vries H.J., Mens J.M.A., van Wingerden J.P. Possible role of the long dorsal sacroiliac ligament in women with peripartum pelvic pain. Acta Obstet. Gynecol. Scand.. 2002;81:430-436.
Vleeming A., Albert H.B., Ostgaard H.C., Sturesson B., Stuge B. European guidelines for the diagnosis and treatment of pelvic girdle pain. [Review] [155 refs]. Eur. Spine J.. 2008;17;(6):794-819.
Walheim G.G., Selvik G. Mobility of the pubic symphysis. vivo measurements with an electromechanic method and a roentgen stereophotogrammetric method. Clin. Orthop. Relat. Res. 1984;191:129-135.
Weisl H. The movements of the sacroiliac joints. Acta Anat. (Basel). 1955;23:80.
Willard F.H. The muscular, ligamentous and neural structure of the lumbosacrum and its relationship to low back pain. In: Vleeming A., Mooney V., Stoeckart R., editors. Movement, Stability and Lumbopelvic Pain. Elsevier; 2007:5-45.