Chapter 13 Visual Pathway
The visual pathway consists of the series of cells and synapses that carry visual information from the environment to the brain for processing. It includes the retina, optic nerve, optic chiasm, optic tract, lateral geniculate nucleus (LGN), optic radiations, and striate cortex (Figure 13-1). The first cell in the pathway—a special sensory cell, the photoreceptor—converts light energy into a neuronal signal that is passed to the bipolar cell and the amacrine cell and then to the ganglion cell; all these cells and synapses lie within the retina. The axons of the ganglion cells exit the retina via the optic nerve, with the nasal fibers from each eye crossing in the optic chiasm and terminating in the opposite side of the brain. The optic tract carries these fibers from the chiasm to the LGN, where the next synapse occurs. The fibers leave the LGN as the optic radiations that terminate in the visual cortex of the occipital lobe. From various points in this pathway, information about the visual environment is transferred to related neurologic centers and to visual association areas.
This chapter discusses the structures of the visual pathway and orientation of the fibers within each structure, then briefly reviews characteristic field defects associated with specific locations in the visual pathway. Most of the current knowledge of the visual pathway is based on degeneration studies using laboratory animals, particularly monkeys and cats.1–3 This type of investigation is based on the finding that damage to a neuron causes the cell and its processes to degenerate. After a small area of nerve tissue is damaged, researchers make serial sections of the tissue through which the neuronal processes are believed to pass. By examining these sections under the microscope, they identify the pathway by determining the location of the degenerating processes. In some studies, small lesions were made in the retina, and the degeneration was followed through the optic nerve, chiasm, and tract into the LGN.1,3 In other studies, lesions were made in the striate cortex, and the degeneration was followed through the optic radiations toward the LGN.2 Whenever possible, reference to studies on the human pathway are cited.
Anatomy of Visual Pathway Structures
The anatomy of the retina and optic disc are discussed in Chapter 4.
Optic Nerve
The retinal nerve fibers make a 90-degree turn at the optic disc and exit as the optic nerve. This nerve consists of visual fibers, 90% of which will terminate in the LGN. Approximately 10% project to areas controlling pupil responses or the circadian rhythm.4 Various counts of the optic nerve fibers range from 1 million to 2.22 million, with their size ranging from small-diameter macular fibers to larger-caliber extramacular fibers.1,2,5–7
The nerve is 5 to 6 cm long and can be divided into four segments on the basis of location: intraocular (0.7 to 1 mm), intraorbital (30 mm), intracanalicular (6 to 10 mm), and intracranial (10 to 16 mm).6,8,9
The intraocular section of the optic nerve can be divided into prelaminar and laminar sections on the basis of association with the lamina cribrosa. In the prelaminar optic nerve, a glial tissue network provides structural support for the delicate nerve fibers; sheaths of astrocytes bundle the nerve fibers into fascicles, containing approximately 1000 fibers each.8 The optic nerve fibers are separated from the retinal layers by a ring of glial tissue, the intermediary tissue (of Kuhnt). The continuation of this glial tissue, the border tissue (of Jacoby), separates the choroid from the optic nerve fibers, and a ring of collagenous tissue of scleral derivation, the marginal (or border) tissue (of Elschnig), lies outer to the glial sheaths.8 Tight junctions within the glial border tissue may prevent leakage from adjacent choriocapillaris into the optic nerve head.10 These layers are shown in Figure 13-2.
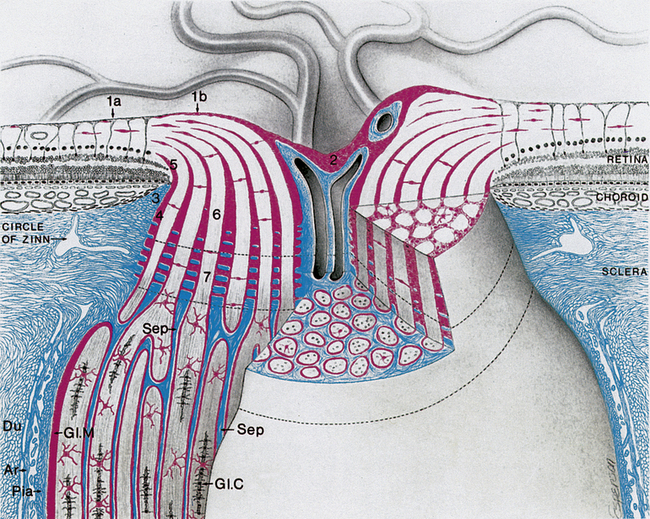
FIGURE 13-2 Intraocular and part of orbital optic nerve.
(From Anderson D, Hoyt W: Ultrastructure of interorbital portion of human and monkey optic nerve, Arch Ophthalmol 82:506, 1969.)
The intraorbital (postlaminar) length exceeds the distance from the globe to the apex of the orbit, giving the nerve a slight sine wave-shaped curve, allowing for full eye excursions without stretching the nerve.6,11 Within the orbit, the nerve is surrounded by the rectus muscles; the sheaths of the superior and medial rectus muscles are adherent to the sheath of the optic nerve (which explains the pain associated with eye movements in optic neuritis).8
The optic nerve is surrounded by three meningeal sheaths continuous with the meningeal coverings of the cranial contents. The outermost sheath, the dura mater, is tough, dense connective tissue containing numerous elastic fibers.8 Next to it, the thin collagenous membrane of the arachnoid sends a fine network of trabeculae through the subarachnoid space and connects to the innermost layer, the pia mater. The subarachnoid space around the optic nerve is continuous with the intracranial subarachnoid space and contains cerebrospinal fluid. The loose, vascular connective tissue of the pia mater branches, sending blood vessels and connective tissue septa into the nerve (see Figure 13-2). All three of these layers fuse and become continuous with the sclera and with the periorbita.8 Of these sheaths, only the pia continues along the intracranial optic nerve.10
As the unmyelinated retinal fibers pass through the scleral perforations of the lamina cribrosa, they become myelinated by oligodendrocytes because no Schwann cells exist in the central nervous system. It is postulated that the lamina cribrosa is a barrier to oligodendrocytes because these cells are not located in retinal tissue and myelination does not normally occur in the retina.10 The sheath of connective tissue branching from and continuous with the pia mater meningeal covering is added to the glial sheath of each fascicle posterior to the lamina (Figure 13-3). These additional tissues double the diameter of the optic nerve as it leaves the eye; the nerve is approximately 1.5 mm in diameter at the level of the retina and 3 mm after its exit from the globe. The septa that separate the fiber fascicles end near the chiasm.8 Astrocytes present in the optic nerve probably function similar to Müller cells of the retina; they provide structure, store glycogen, and regulate the extracellular concentration of certain ions.10
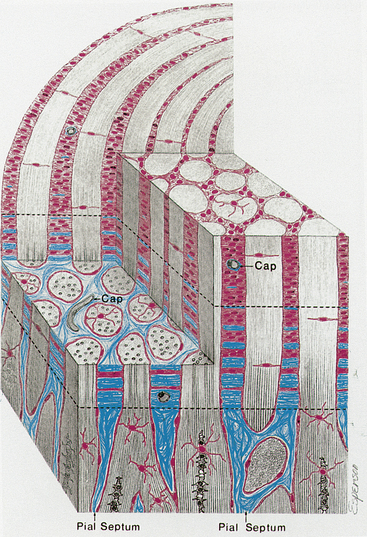
FIGURE 13-3 Prelaminar, laminar, and postlaminar optic nerve.
(From Anderson D: Ultrastructure of human and monkey lamina cribrosa and optic nerve head, Arch Ophthalmol 82:800, 1969.)
The anterior perforated substance, the root of the olfactory tract, and the anterior cerebral artery lie superior to the optic nerve in its intracranial path. The sphenoid sinus is medial, with only a thin plate of bone separating it from the nerve.8 The internal carotid artery is below and then lateral to the nerve, and the ophthalmic artery enters the dural sheath of the optic nerve as it passes through the optic canal.
Optic Chiasm
The optic chiasm is roughly rectangular, approximately 15 mm in its horizontal diameter, 8 mm anterior to posterior, and 4 mm high.6,9,12 As with the optic nerve, the optic chiasm is surrounded by the meningeal sheaths and cerebrospinal fluid.
The chiasm lies within the circle of Willis, a circle of blood vessels that is a common location for aneurysms.6 The circle of Willis is an anastomotic group of anterior and posterior arteries that join the anterior circulation of the internal carotid arteries with the posterior circulation of the basilar artery (Figure 13-4). The internal carotid arteries supply the anterior cranial regions, including most of the cerebral hemispheres and orbital and ocular structures. The vertebral branches of the basilar artery supply the posterior regions, including the brainstem, occipital lobes, and inferomedial temporal lobes, thus supplying most of the ocular motor centers and the cortical visual areas.13 If the circle is complete, the anterior cerebral arteries are joined via the anterior communicating artery, and each internal carotid artery is joined to the ipsilateral posterior cerebral artery by a posterior communicating artery. The anterior cerebral and anterior communicating arteries are anterior to the chiasm, and an internal carotid artery lies on each lateral side of the chiasm.
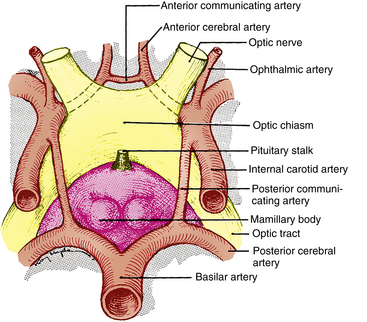
FIGURE 13-4 Relationship of optic chiasm to vessels of circle of Willis.
(From Harrington DO: The visual fields, ed 5, St Louis, 1981, Mosby.)
Above the optic chiasm is the floor of the third ventricle, and approximately 1 cm below the chiasm is the pituitary gland (Figure 13-5). The position of the optic chiasm above the sella turcica (the fossa in which the pituitary gland sits) can vary from being directly above it (in 75% of the population) to a position referred to as prefixed (if the optic nerves are short and the gland lies below the posterior part of the chiasm) or postfixed (if the optic nerves are long and the gland is situated toward the anterior of the chiasm).12 The chiasm is anteriorly displaced in approximately 10% of individuals and posteriorly displaced in 15%.10
Optic Tract
The optic tract is a cylindric, slightly flattened band of fibers approximately 3.5 mm high and 5.1 mm long that runs from the posterolateral corner of the optic chiasm to the LGN.9 Most of the fibers (which are still the axons of retinal ganglion cells) terminate in the LGN. Fibers from the retinal ganglion cells may branch so that the same cell sends fibers to various target structures or some axons may be destined for a specific structure. The afferent fibers of the pupillomotor reflex leave the optic tract before reaching the LGN and pass by way of the superior brachium to the pretectal nucleus in the midbrain. Other fibers project to areas in the hypothalamus involved with the circadian rhythm, and others terminate in the superior colliculus. The rather poorly defined accessory optic system, including the nucleus of the optic tract, is involved in the optokinetic nystagmus response and receives information generated by retinal ganglion cells.4
The optic tract lies along the upper anterior and then the lateral surface of the cerebral peduncle and is parallel to the posterior cerebral artery. The globus pallidus is above, the internal capsule is medial, and the hippocampus is below the optic tract.8
Lateral Geniculate Nucleus
Information from all the sensory systems except the olfactory pass through the thalamus before being transferred to the cerebral cortex; visual information is processed in the LGN and then is relayed to higher cortical centers.14 The lateral geniculate nucleus (LGN, lateral geniculate body) is located on the dorsolateral aspect of the thalamus and resembles an asymmetric cone, the rounded apex of which is oriented laterally. The retinal axons terminate here. Most of the fibers that leave the LGN project to the visual cortex.
The LGN is a layered structure; the layers are piled on each other, with the larger ones draping over smaller ones, and some layers becoming fragmented and irregular. The cells within a layer are all of the same type, and three types have been identified according to size. Magnocellular layers contain large cells, parvocellular layers contain medium-sized cells, and koniocellular layers contain small cells. The number of layers present depends on the location of the plane through the structure. In the classic textbook presentation of the LGN, six layers are seen. Two magnocellular layers are located inferiorly and numbered 1 and 2, and four parvocellular layers are above them and numbered 3, 4, 5, and 6 (Figure 13-6). Below each of these six layers lies a koniocellular layer (Figure 13-7). The retinal ganglion cells that project to each of these layers differ in a number of their characteristics.15
The LGN is not a simple relay station; it also receives input from cortical and subcortical centers and reciprocal innervation from the visual cortex and is a center of complex processing.6,16 It regulates the flow of visual information, ensuring that the most important information is sent to the cortex.17 The optic tract enters the LGN anteriorly; the internal capsule is lateral, the medial geniculate nucleus is medial, and the inferior horn of the lateral ventricle is posterolateral to the LGN.8 The axons leave the LGN as the optic radiations.
Optic Radiations (Geniculocalcarine Tract)
The optic radiations spread out fanwise as they leave the LGN, deep in the white matter of the cerebral hemispheres, sweeping laterally and inferiorly around the anterior tip of the temporal horn of the lateral ventricle (Figure 13-8). Some fibers loop into the temporal lobe en route to the occipital lobe. The fibers within the parietal lobe pass lateral to the occipital horn of the lateral ventricle before terminating in the striate cortex.8,18
Primary Visual Cortex (Striate Cortex)
The primary visual cortex (Brodmann area 17 or, according to more recent nomenclature, V1), is located almost entirely on the medial surface of the occipital lobe; just a small portion (perhaps 1 cm long) extends around the posterior pole onto the lateral surface. The visual cortex also is called the striate cortex because a white myelinated fiber layer, the white stria of Gennari, is characteristic of this area.6 The calcarine fissure extends from the parieto-occipital sulcus to the posterior pole, dividing the visual cortex into an upper portion (the cuneus gyrus) and a lower part (the lingual gyrus) (Figure 13-9); most of the primary visual cortex is buried in the tissue within the calcarine fissure.19
The primary visual cortex has a thickness of about 2 mm and is organized into horizontal layers and vertical columns. Layer I, the most superficial layer, contains a few scattered neurons. Layer II contains neurons that send axons only to deeper cortical layers. Layer III contains neurons that communicate with both near and far cortical locations. Layer IV contains the stria of Gennari and is subdivided into strata, one of which receives information from the magnocellular layers and another that receives information from the parvocellular layers.20,21 Layer IV sends axons to more superficial visual cortex, as well as other visual cortical areas. Layer V sends axons to the superior colliculus and other areas in the brainstem. Layer VI sends projections back to the LGN.17
Certain cortical regions are active during motion stimulation, whereas others are active during color vision.22 The magnocellular areas probably mediate movement detection and low-spatial-frequency contrast sensitivity, and the parvocellular areas likely mediate color and high-spatial-frequency contrast sensitivity, although this generalization oversimplifies the properties.23–26
Cells are also distributed in a vertical organization, according to the eye of origin, forming alternating parallel ocular dominance columns.21,27,28 These columns are lacking in the area of the cortex that represents the physiologic blind spot because this region receives information exclusively from one eye.14 A second system of columns, specific for stimulus orientation, responds on the basis of the direction of a light slit or edge.21 Contour analysis and binocular vision are two functions of the visual cortex, and such processing is a function of both its horizontal and its vertical organization. The cells within the striate cortex are activated only by input from the LGN, although other cortical areas have input into the striate cortex.16,29,30 The striate cortex communicates with the superior colliculus and the frontal eye fields.
The superior colliculus, which has a complete retinotopic map of the contralateral field of vision, also receives communication from fibers exiting the posterior optic tract. It does not analyze sensory information for perception but is important for visual orientation, foveation, and the control of saccadic eye movements with input from the frontal eye fields.14,31 The frontal eye fields, in the frontal lobe, receive fibers from the striate cortex that contribute to the control of conjugate eye movements. Both voluntary and reflex ocular movements are mediated in this area, as are pupillary responses to near objects (see Chapter 14).8
The striate cortex combines and analyzes the visual information relayed from the LGN and transmits this information to the higher visual association areas (the extrastriate cortex), which provide further interpretation.14 These areas surround the striate cortex and are located on the lateral aspects of the occipital cortex. Historically called Brodmann areas 18 and 19 (Figure 13-10), these areas now are known to contain several distinct cortical areas (designated V2, V3, V4, and V5) in which visual processing occurs. A study involving the macaque monkey has identified 32 such areas associated with visual processing.14 The visual and visual association areas in one hemisphere are connected to the corresponding areas in the other hemisphere through the posterior portion of the corpus callosum.6 Magnetic resonance imaging (MRI) techniques that are sensitive to changes in blood flow and oxygenation occurring with neuronal activity can be used to study the human visual system in vivo. Innovative studies are attempting (1) to identify the areas of visual cortex and associated visual areas activated during visual stimulation and visual processing, (2) to detect the storage areas for learned visual patterns, and (3) to establish the pathway of activation in the cortex for recall and recognition of a visual pattern.32–38