Chapter 2 Cornea and Sclera
The outer connective tissue coat of the eye has the appearance of two joined spheres. The smaller, anterior transparent sphere is the cornea and has a radius of curvature of approximately 8 mm. The larger, posterior opaque sphere is the sclera, which has a radius of approximately 12 mm (Figure 2-1, A). The globe is not symmetric; its approximate diameters are 24 mm anteroposterior, 23 mm vertical, and 23.5 mm horizontal.1
Cornea
Corneal Dimensions
The transparent cornea appears from the front to be oval, as the sclera encroaches on the superior and inferior aspects. The anterior horizontal diameter is 12 mm, and the anterior vertical diameter is 11 mm.1,2 If viewed from behind, the cornea appears circular, with horizontal and vertical diameters of 11.7 mm (Figure 2-1, B).1
In profile, the cornea has an elliptic rather than a spherical shape, the curvature being steeper in the center and flatter near the periphery. The radius of curvature of the central cornea at the anterior surface is 7.8 mm and at the posterior surface is 6.5 mm.1,3 The central corneal thickness is 0.53 mm, whereas the corneal periphery is 0.71 mm thick (Figure 2-1, C).1,3–5 (All values given are approximations.)
Clinical Comment: Astigmatism
Regular astigmatism occurs when the longest radius of curvature and shortest radius of curvature lie 90 degrees apart. The usual presentation occurs when the radius of curvature of the vertical meridian differs from that of the horizontal meridian. The most common situation, called with-the-rule astigmatism (Figure 2-2, A), occurs when the steepest curvature lies in the vertical meridian. Thus the vertical meridian has the shortest radius of curvature. Against-the-rule astigmatism (Figure 2-2, B) is not as common and occurs when the horizontal meridian is the steepest; the greatest refractive power is found in the horizontal meridian. If the meridians that contain the greatest differences are not along the 180- and 90-degree axes (± 30 degrees) but lie along the 45- and 135-degree axes (± 15 degrees), the astigmatism is called oblique. Irregular astigmatism is an uncommon finding in which the meridians corresponding to the greatest differences are not 90 degrees apart.
Corneal Histologic Features
The cornea is the principal refracting component of the eye. Its transparency and avascularity provide optimal light transmittance. The anterior surface of the cornea is covered by the tear film, and the posterior surface borders the aqueous-filled anterior chamber. At its periphery, the cornea is continuous with the conjunctiva and the sclera. From anterior to posterior, the five layers that compose the cornea are epithelium, Bowman’s layer, stroma, Descemet’s membrane, and endothelium (Figure 2-3).
Epithelium
The outermost layer of stratified corneal epithelium is five to seven cells thick and measures approximately 50 μm.1,6 The epithelium thickens in the periphery and is continuous with the conjunctival epithelium at the limbus.
The surface layer of corneal epithelium is two cells thick and displays a very smooth anterior surface. It consists of nonkeratinized squamous cells, each of which contains a flattened nucleus and fewer cellular organelles than deeper cells. Cell size varies but a superficial cell can be 50 μm in diameter and 5 μm in height.7 The plasma membrane of the surface epithelial cells secretes a glycocalyx component that adjoins the mucin layer of the tear film.8–10 Many projections located on the apical surface of the outermost cells increase the surface area, thus enhancing the stability of the tear film. The fingerlike projections are microvilli, and the ridgelike projections are microplicae (Figure 2-4).
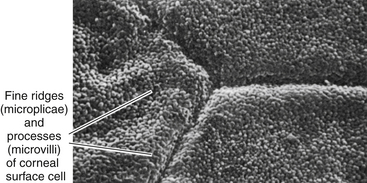
FIGURE 2-4 Scanning electron micrograph of junction of three superficial cells in cornea. (×5000.)
(From Krause WJ, Cutts JH: Concise text of histology, Baltimore, 1981, Williams & Wilkins.)
As the surface cells age, they degenerate. The cytoskeleton disassembles and the cytoplasm condenses. The cells lose their attachments and are sloughed off, being constantly replaced from the layers below. On scanning electron microscopy, the corneal surface consists of variously sized cells, ranging from small to large. The lighter cells are newer replacement cells, whereas the darker cells are those that are degenerating and will soon be sloughed.11
The middle layer of the corneal epithelium is made up of two to three layers of wing cells. These cells have winglike lateral processes, are polyhedral, and have convex anterior surfaces and concave posterior surfaces that fit over the basal cells (Figure 2-5). The diameter of a wing cell is approximately 20 μm.7 Desmosomes and gap junctions join wing cells to each other, and desmosomes join wing cells to surface and basal cells.12
The innermost basal cell layer of the corneal epithelium is a single layer of columnar cells, with diameters ranging from 8 to 10 μm (Figure 2-6).7 These cells contain oval-shaped nuclei displaced toward the apex and oriented at right angles to the surface. The rounded, apical surface of each cell lies adjacent to the wing cells, and the basal surface attaches to the underlying basement membrane (basal lamina). The basal cells secrete this basement membrane, which attaches the cells to the underlying tissue through hemidesmosomes. Anchoring fibrils pass from these junctions through Bowman’s layer into the stroma.13 Although less numerous here than in the wing cell layer, desmosomes and gap junctions join the columnar cells; interdigitations and desmosomes connect the basal cells with the adjacent layer of wing cells. The basal layer is the germinal layer where mitosis occurs.
The basal cells are joined to keratin filaments in the basement membrane by hemidesmosomes. Opposite the plaque, fine anchoring collagen fibrils form a complex branching and anastomosing network that runs from the basement membrane through Bowman’s layer and penetrates 1.5 to 2 μm into the stroma.13–18 The linkage between the hemidesmosome and the anchoring network is likely composed of basement membrane components.5 The anchoring fibrils attach to anchoring laminin-containing plaques of extracellular matrix within the stroma.16,19
Epithelial Replacement
Maintenance of the smooth corneal surface depends on replacement of the surface cells that are continually being shed into the tear film. This renewal of the stratified epithelium involves cell division, migration, differentiation, and senescence. Cell proliferation occurs in the basal layer. Basal cells move up to become wing cells, and wing cells move up to become surface cells. Only the cells in contact with the basement membrane have the ability to divide; the cells that are displaced into the wing cell layers lose this ability.20 Stem cells located in a 0.5- to 1-mm-wide band around the corneal periphery are the source for renewal of the corneal basal cell layer. A slow migration of basal cells occurs from the periphery toward the center of the cornea.21,22 Turnover time for the entire corneal epithelium is approximately 7 days, which is more rapid than for other epithelial tissues.23,24 Repair to corneal epithelial tissue proceeds quickly; minor abrasions heal within hours, and larger ones often heal overnight. If the basement membrane is damaged, however, complete healing with replacement basement membrane and hemidesmosomes can take months.14,15
Despite cells constantly being sloughed, the barrier function is maintained as the cell below moves into position to replace the one that has been shed. Tight junctions are present exclusively between the squamous cells that occupy the superficial position. The protein components necessary to form these junctions are not present in the basal cells but are increasingly present as the cells move up to the surface where the zonula occludens junctions become complete.25
The basal cell layer is continually losing and reestablishing the hemidesmosome junctions as cells divide and move up into the wing cell layers. The plaque sites remain present in the stroma for reattachment.15
Clinical Comment: Recurrent Corneal Erosion
RECURRENT CORNEAL EROSION is a condition in which the corneal epithelium sloughs off either continually or periodically. This condition may occur because of either poor attachment between the epithelium and its basement membrane or poor attachment between the basement membrane and the underlying tissue. Recurrent corneal erosion can occur after incomplete healing of an abrasion in which the hemidesmosomes are malformed, or it may be caused by an epithelial basement membrane dystrophy stemming from defective nutrition or metabolism.5
Age-related changes also can play a role in recurrent corneal erosion. Epithelium continues to secrete basement membrane throughout life; in the corneal epithelium, the thickness of the basement membrane doubles by 60 years of age. In addition, areas of reduplication of the membrane can occur with aging.26 As the basement membrane thickens or as reduplication occurs, the thickness of the membrane can exceed the length of the anchoring fibrils, allowing sloughing of epithelial layers.
Corneal erosions are very painful because the dense network of sensory nerve endings in the epithelium is disrupted. A number of treatments may be used. Acute cases may be patched and antibiotic ointment applied to allow healing of the surface without the shearing effect of opening and closing the eyelids. Bandage soft contact lenses or collagen shields often are applied in chronic situations to alleviate pain.26–29 For cases in which the suspected cause is a faulty basement membrane, treatment might include corneal puncture in which multiple perforations are made through the epithelial layers to induce new basement membrane formation and adhesion30–32 (Figure 2-7). If reduplication is the cause of corneal erosion, the doubled membrane can be removed.32
Bowman’s Layer
The second layer of the cornea is approximately 8 to 14 μm thick.1,6,33 Bowman’s layer is a dense, fibrous sheet of interwoven collagen fibrils randomly arranged in a mucoprotein ground substance. The fibrils have a diameter of 20 to 25 nm, run in various directions, and are not ordered into bundles. Bowman’s layer sometimes is referred to as a “membrane,” but it is more correctly a transition layer to the stroma rather than a true membrane. It differs from the stroma in that it is acellular and contains collagen fibrils of a smaller diameter. Bowman’s layer might provide biomechanical rigidity and shape to the cornea. The pattern of the anterior surface is irregular and reflects the contour of the bases of the basal cells of the epithelium. Posteriorly, as the layer transitions into stroma, the fibrils gradually adopt a more orderly arrangement and begin to merge into bundles that intermingle with those of the stroma (Figure 2-8). The posterior surface is not clearly defined.33
Bowman’s layer is produced prenatally by the epithelium and is not believed to regenerate. Therefore, if injured, the layer usually is replaced by epithelial cells or stromal scar tissue. However, Bowman’s layer is very resistant to damage by shearing, penetration, or infection. Speculation continues regarding the function of Bowman’s layer and whether it is necessary to maintain corneal function. No long-term effects have been documented in patients with Bowman’s layer removed by photorefractive keratoplasty, a procedure performed since the late 1980s.34
Corneal nerves passing through Bowman’s layer typically lose their Schwann cell covering and pass into the epithelium as naked nerves (see Figure 2-5). The layer tapers and ends at the corneal periphery and does not have a counterpart in either the conjunctiva or the sclera.
Stroma or Substantia Propria
The middle layer of the cornea is approximately 500 μm thick, or about 90% of the total corneal thickness7 (see Figure 2-3). The stroma (substantia propria) is composed of collagen fibrils, keratocytes, and extracellular ground substance.
The collagen fibrils have a uniform 25- to 35-nm diameter and run parallel to one another, forming flat bundles called lamellae.33 The 200 to 300 lamellae are distributed throughout the stroma and lie parallel to the corneal surface. Each contains uniformly straight collagen fibrils arranged with regular spacing, sometimes described as a “latticework.” The fibrils are also oriented parallel to the corneal surface. Adjacent lamellae lie at angles to one another, but all fibrils within a lamella run in the same direction (Figure 2-9). Each lamella extends across the entire cornea, and each fibril runs from limbus to limbus. Interweaving occurs between the lamellae.35,36
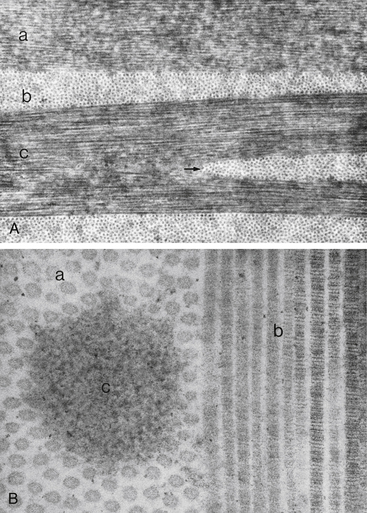
FIGURE 2-9 Corneal stroma.
(From Hogan MJ, Alvarado JA, Weddell JE: Histology of the human eye, Philadelphia, 1971, Saunders.)
The arrangement of the lamellae varies slightly within the stroma. In the anterior one third of the stroma, the lamellae are thin (0.5 to 30 μm wide and 0.2 to 1.2 μm thick), and they branch and interweave more than in the deeper layers.33,37 In the posterior two thirds of the stroma, the arrangement is more regular, and the lamellae become larger (100 to 200 μm wide and 1 to 2.5 μm thick).33 Anterior cornea has a higher incidence of cross-linking and is more rigid, helping to maintain corneal curvature.38
In the innermost layer, adjacent to Descemet’s membrane, the fibrils interlace to form a thin collagenous sheet that contributes to the binding between stroma and Descemet’s membrane.33
Keratocytes (corneal fibroblasts) are flattened cells that lie between and occasionally within the lamellae39 (Figure 2-10). The cells are not distributed randomly; a corkscrew pattern is recognizable from anterior to posterior, with the density higher in anterior stroma.40 Keratocytes have extensive branching processes joined by gap junctions along the lateral extensions, as well as the anteroposterior branches.41,42 These are active cells that maintain the stroma by synthesizing collagen and extracellular matrix components. Other cells may be found between lamellae, including white blood cells, lymphocytes, macrophages, and polymorphonuclear leukocytes, which can increase in number in pathologic conditions.
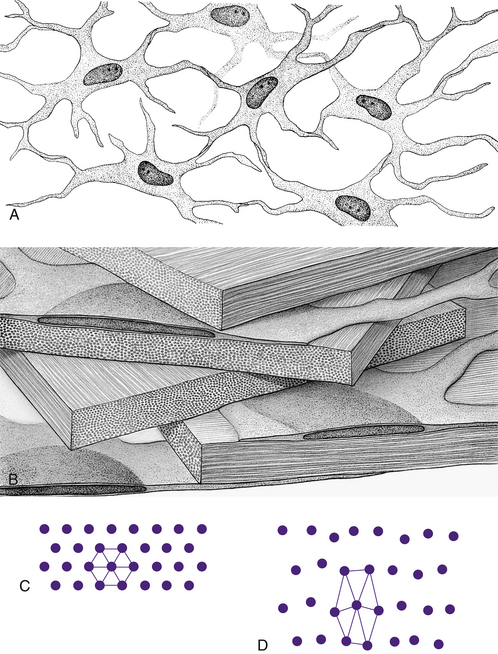
FIGURE 2-10 Summary diagram of corneal stroma.
(From Hogan MJ, Alvarado JA, Weddell JE: Histology of the human eye, Philadelphia, 1971, Saunders.)
Ground substance fills the areas between fibrils, lamellae, and cells. It contains proteoglycans (PG), macromolecules consisting of a core protein with one or more attached glycosaminoglycan (GAG) side chains. The corneal proteoglycans were once classified by their side chains: keratan sulphate (KS) and chondroitin/dermatan sulphate (CS/DS).43 They are now named for their core proteins, decorin is a CS/DS proteoglycan, and lumican, keratocan, and mimican are KS proteoglycans. Decorin is more abundant in anterior stroma; lumican, keratocan, and mimican are more abundant in posterior stroma.44 Lumican controls collagen fibril diameter keeping it within a very limited range.45 PGs have a significant role in maintaining corneal tensile strength and the GAGs contribute to the relatively high stromal hydration.44 Glycosaminoglycans are hydrophilic, negatively charged carbohydrate molecules located at specific sites around each collagen fibril. They attract and bind with water, maintaining the precise spatial relationship between individual fibrils.45
The very regular arrangement of the stromal components, as well as the small diameter of the fibrils, contributes to stromal transparency.46 The index of refraction of the fibrils is 1.411 and that of the extracellular matrix is 1.365. Studies have shown that the distance between areas of different refractive indices can affect transparency. If the change in the index of refraction occurs across a distance that is less than one half the wavelength of visible light (400 to 700 nm), destructive interference occurs, and light scattering is reduced significantly.40,47,48 In the stroma the very specific spacing between the fibrils allows destructive interference of rays reflecting from adjacent fibrils. Although the components of the epithelium, Bowman’s layer, and Descemet’s membrane are arranged irregularly, the scattering particles are separated by such small distances that light scattering is minimal in these layers.37 The cornea scatters less than 1% of the light that enters it.6,49
Clinical Comment: Keratoconus
KERATOCONUS is a corneal dystrophy that first presents with focal disruptions of basement membrane and Bowman’s layer. Metabolic and nutritional disturbances are among the possible causes. Normal corneal shape and strength are maintained by the arrangement and density of the collagen fibrils that lie parallel to each other and the surface. Two mechanisms likely play some role in keratoconus: tissue loss caused by enzymatic degradation and the loss of adhesive forces between collagen fibrils that can cause slippage and displacement of lamellae.50 The process usually begins in central cornea; the stroma eventually degenerates and thins, and the affected area projects outward in a cone shape because of the force exerted on the weakened area by intraocular pressure (Figure 2-11, A). The cone shape is most evident in downgaze when the lower lid conforms to the cone shape; this is known as Munson’s sign (Figure 2-11, B). Folds occur in the posterior stroma and Descemet’s membrane.51,52
Spectacles may be used for a time for correction of refractive error, but with increasing irregular astigmatism, rigid gas-permeable contact lenses usually are necessary to achieve best corrected vision.53 When contact lenses no longer correct vision, penetrating keratoplasty may be performed to replace the defective cornea with a donor cornea.
Currently clinical trials are being conducted to evaluate a procedure called collagen cross-linking. In this treatment, after removing the epithelium, the stroma is saturated with topical riboflavin; it is then exposed to ultraviolet radiation that interacts with the riboflavin creating chemical bonds between and within the collagen fibrils. The corneal collagen stiffens, decreasing the progression of keratoconus.54 Initial results are promising.55,56
Descemet’s Membrane
Descemet’s membrane is considered the basement membrane of the endothelium. It is produced continually and therefore thickens throughout life, such that it has doubled by age 40 years.26 In children it is 5 μm thick and will increase to approximately 15 μm over a lifetime (Figure 2-12).
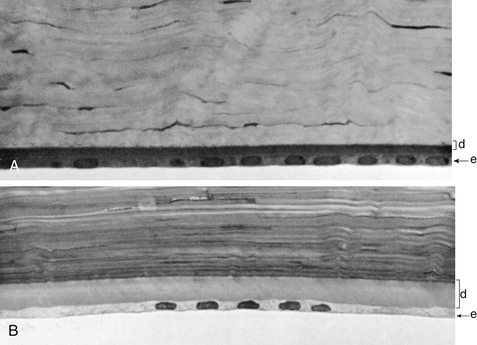
FIGURE 2-12 Thickness of Descemet’s membrane changes with increasing age.
(From Hogan MJ, Alvarado JA, Weddell JE: Histology of the human eye, Philadelphia, 1971, Saunders, p 94.)
Descemet’s membrane consists of two laminae. The anterior lamina, approximately 3 μm thick, exhibits a banded appearance and is a latticework of collagen fibrils secreted during embryonic development. The posterior lamina is nonbanded and homogeneous; it is the portion secreted by the endothelium throughout life.57
The method of attachment between Descemet’s membrane and the neighboring layers is poorly understood. Attachment sites between the stroma and Descemet’s membrane are relatively weak; the membrane can be detached easily from the posterior stroma.19 The anchoring fibrils characteristic of the connective tissue component of the hemidesmosome are not seen in Descemet’s58 and so the adhesions between Descemet’s membrane and the endothelium are not the typical hemidesmosomes.59
Endothelium
The innermost layer of the cornea, the endothelium, lies adjacent to the anterior chamber and is composed of a single layer of flattened cells. It normally is 5 μm thick.6 The basal part of each cell rests on Descemet’s membrane, and the apical surface, from which microvilli extend, lines the anterior chamber (Figure 2-13). Endothelial cells are polyhedral: five-sided and seven-sided cells can be found in normal cornea, but 70% to 80% are hexagonal. The hexagon is considered the most efficacious shape to provide area coverage without gaps.5,60 The very regular arrangement of these cells is described as the endothelial mosaic (Figure 2-14).
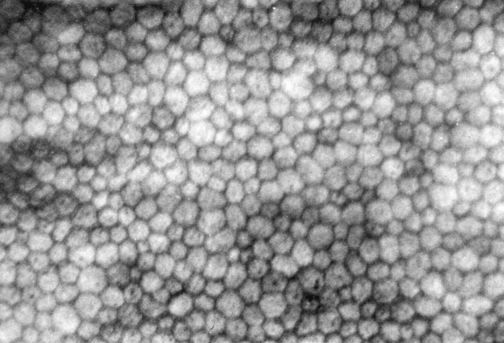
FIGURE 2-14 View with specular reflection through biomicroscope showing the endothelial mosaic.
(Courtesy Patrick Caroline, C.O.T., Pacific University College of Optometry, Forest Grove, Ore.)
Although Descemet’s membrane is considered a basement membrane, the nature of the junctions joining it to the endothelium are undefined.61 Extensive interdigitations join the lateral walls of the cells, and gap junctions provide intercellular communication.12 Tight junctional complexes joining the endothelial cells are located near the cell apex; these are a series of macula occludens rather than zonula occludens.62,63
The barrier formed by these adhesions is slightly leaky; in experiments, large molecules have penetrated the intercellular spaces.64 This incomplete barrier allows the entrance of nutrients, including glucose and amino acids, from the aqueous humor. Excess water that accompanies these nutrients must be moved out of the cornea if proper hydration is to be maintained. Metabolic pump mechanisms are active throughout the cells of the endothelium and function continually to move ions across the cell membranes; lateral infoldings increase the surface area providing space necessary for the number of ionic pumps needed. With changes in solute concentration, water flows down the concentration gradient, thus maintaining a balance of fluid movement across the endothelium. The endothelial cell is rich in cellular organelles; mitochondria reflect high metabolic activity and are more numerous in these cells than in any other cells of the eye, except the retinal photoreceptor cells.6
Endothelial cells do not divide and replicate. Studies now suggest that endothelial cells in the adult possess proliferative capacity but are in an arrested phase in the cell cycle. The cell-to-cell contact may be one factor that maintains this layer in the nonproliferative state.59,61,65 The lack of proliferation may be necessary for the layer to maintain its barrier and pump functions.59 Even in children, cells migrate and spread out to cover a defect, with resultant cell thinning. The cell density (cells per unit area) of the endothelium decreases normally with aging because of cell disintegration; density ranges from 3000 to 4000 cells/mm2 in children to 1000 to 2000 cells/mm2 at age 80 years.5,62,66,67 The minimum cell density necessary for adequate function is in the range of 400 to 700 cells/mm2.68 Disruptions to the endothelial mosaic can include endothelial cell loss or an increase in the variability of cell shape (pleomorphism) or size (polymegathism) (Figure 2-15). The active pump function can be detrimentally affected by polymegathism or morphologic changes, although the endothelial barrier function is not compromised by a moderate loss of cells.69 An excessive loss of cells can disrupt the intercellular junctions and allow excess aqueous to flow into the stroma, and the endothelial pumps may be unable to compensate for this loss of barrier function.
Clinical Comment: Hassall-Henle Bodies and Guttata
The endothelium can produce mounds of basement membrane material, which are seen as periodic thickenings in Descemet’s membrane that bulge into the anterior chamber. Those located near the corneal periphery are called Hassall-Henle bodies. These bodies are a common finding, and their incidence increases with age. Such deposits of basement membrane in the central cornea are called corneal guttata and are indicative of endothelial dysfunction. The endothelium that covers these mounds is thinned and altered, and the endothelial barrier may be compromised. Both Hassall-Henle bodies and guttata are visible as dark areas when viewed with specular reflection with the biomicroscope. These may be interpreted as holes in the endothelium, but the endothelium is merely displaced posteriorly from the plane of reflection (Figure 2-16).
Clinical Comment: Effects of Contact Lenses
Clinical studies indicate that epithelial thinning, stromal thinning, and a decreased number of keratocytes are associated with long-term extended wear of contact lenses.70,71 Numerous studies show that contact lens wear can induce changes in the regularity of the endothelial mosaic.72–76 Pleomorphism and polymegathism have been documented after only six years of either rigid gas-permeable or soft contact lens wear, although cell density remained normal.77,78 Endothelial stress resulting from contact lens wear, disease, surgery, or age can lead to endothelial remodeling, including change in size and shape or both.
Corneal Function
The cornea has two primary functions: to refract light and to transmit light. Factors that affect the amount of corneal refraction include (1) the curvature of the anterior corneal surface, (2) the change in refractive index from air to cornea (actually the tear film), (3) corneal thickness, (4) the curvature of the posterior corneal surface, and (5) the change in refractive index from cornea to aqueous humor. The total refractive power of the eye focused at infinity is between 60 and 65 diopters (D), with 43 to 48 D attributable to the cornea.5
In the transmission of light through the cornea, it is important that minimal scattering and distortion occur. Scattering of incident light is minimized by the smooth optical surface formed by the corneal epithelium and its tear film covering. The regular arrangement of the surface epithelial cells provides a relatively smooth surface, and the tear film fills in slight irregularities between cells producing negligible scatter of incident light. The absence of blood vessels and the maintenance of the correct spatial arrangement of components account for minimal scattering and distortion as light rays pass through the tissue. The cornea scatters less than 1% of the visible incident light6,49 and the majority of that scatter as determined by examination with the confocal microscope occurs due to the epithelium and endothelium.48 The epithelial and endothelial cell cytoplasm contain large amounts of water-soluble proteins, which enable the cytoplasm to appear homogenous and help to diminish light scattering. These proteins are now called corneal crystallins, and they share many of the attributes of the long recognized lens crystallins, important in maintaining the transparency of the lens.77
Because the stroma makes up 90% of the cornea, the regularity of spacing between the collagen fibrils is important in maintaining corneal transparency. The negatively charged molecules located around each collagen fibril maintain this precise arrangement by their bonds with water molecules, and corneal transparency is optimal when the stroma is 75% to 80% water.4,78
Corneal Hydration
This relative corneal deturgescence (78% water content) requires precise control of stromal extracellular water content and is dependent upon: (1) the barrier functions of the epithelium and endothelium, (2) the anionic characteristics of molecules within the stromal matrix that account for the tendency of the stroma to imbibe water, and (3) water and ion transport through the epithelial and endothelial cell membranes (including ion channels, ion cotransporters, and energy-utilizing ion pumps). Fluid is continually entering the cornea through the leaky barrier formed by the junctions joining the endothelial cells. Ion transporters in both the epithelium and the endothelium help to maintain the concentration gradient change that can facilitate water movement from the stroma into the tear film through the epithelium and into the anterior chamber through the endothelium. Net transport of solute into the anterior chamber exceeds that into the tears and corneal deturgescence is primarily reliant on endothelium and minimally on epithelium.79