Chapter 128 Vertebroplasty and Kyphoplasty
Osteoporotic Vertebral Compression Fractures
Osteoporosis and associated fragility fractures are major threats to the health of aging populations worldwide.1 At 50 to 79 years of age, the incidence of a new vertebral compression fracture (VCF) in Europe is 1% per year in women and 0.6% per year in men, and at 75 to 79 years of age, the incidence is 2.9% per year in women and 1.4% per year in men.2 In the United States, approximately 750,000 people are affected, and only one third receive treatment.3 Despite nonsurgical management, including analgesia, bed rest, physiotherapy, and back bracing, pain sometimes resolves slowly and can persist.4 The resulting vertebral deformity can cause height loss, kyphosis, reduced pulmonary function, and mobility and balance impairment. VCFs are associated with an increased incidence of mortality and morbidity, including back pain, loss of height, kyphotic deformity, increased risk of future fracture, and reduction in quality of life.5 Osteoporotic VCFs are clearly a clinically significant health problem with continuously increasing economic and social ramifications. The National Osteoporosis Foundation predicts that the number of vertebral body compression fractures will double in the next 15 years due to the aging population and increasingly sedentary lifestyles.
Nonsurgical Treatment
In recent rears, various osteoporosis treatments have been developed to reduce the risk of fracture.6,7 As a treatment for VCFs, traditional, nonoperative management includes bedrest, analgesics, and bracing. However, these types of medical management fail to restore spinal alignment, and the lack of mobility itself can result in secondary complications, including worsening osteoporosis, atelectasis, pneumonia, deep vein thrombosis, decubitus ulcer, and pulmonary embolism. An alternative approach is supervised ambulatory mobility by a physiotherapist plus hydrotherapy.8 In one third of patients, severe pain, limited mobility, and poor quality of life persist despite appropriate nonoperative management. Whether the pain has or has not resolved, no patient after a VCF spontaneously achieves a realigned spine, corrected sagittal contour, or restoration of vertebral height.
Surgical Treatment
Historically, the only alternative to nonoperative management for symptomatic vertebral fractures was open surgical decompression (ventral or dorsal decompression and stabilization via internal fixation hardware and bone grafting), and this was usually reserved for those patients with gross spinal deformity or neurologic impairment (<0.5%).9 The reason for this surgical caution was the adverse risk/benefit ratio in this elderly population with poor bone quality and multiple comorbid conditions.
Percutaneous vertebroplasty (PVP) is a minimally invasive method that involves the percutaneous injection of polymethylmethacrylate (PMMA) into a collapsed vertebral body to stabilize the vertebra. Originally developed for osteolytic metastasis, myeloma, and hemangioma, the procedure resulted in quick, effective pain relief and a low complication rate.10–12 PVP is now also increasingly used for the treatment of osteoporotic vertebral fractures.13 However, PVP does not expand the collapsed vertebra, potentially locking the spine in a kyphotic posture. In addition, the PMMA bone filler has associated problems (epidural leakage, thermal necrosis, inability to integrate with bone, handling difficulties, toxicity to patient and operator).14,15
Kyphoplasty is an advanced minimally invasive technique with a number of potential advantages over PVP, including lower risk of cement extravasation and better restoration of vertebral body height.16 A cannula is introduced into the vertebral body, followed by insertion of an inflatable bone tamp, which when deployed, reduces the compression fracture and restores the vertebral body toward its original height, while creating a cavity to be filled with bone cement. The cement augmentation is therefore done with more control into the low-pressure environment of the preformed cavity with viscous, partially cured cement.
Vertebral Augmentation
Percutaneous Vertebroplasty
Background
Percutaneous vertebral augmentation (vertebroplasty, PVP) was first reported by Galibert et al. in 1984 and initially involved the augmentation of the vertebral body with PMMA to treat a hemangioma. PVP was reportedly not performed in the United States until 1994. Originally targeted for osteolytic metastasis, myeloma, and hemangioma, PVP resulted in early appreciable pain relief and a low complication rate.12,17 Its indications subsequently expanded to osteoporotic vertebral collapse with chronic pain, further to include treatment of asymptomatic vertebral collapse and even prophylactic intervention for at-risk vertebral bodies.18 Nevertheless, the treatment of acute fractures in ambulatory patients and prophylactic treatment remain controversial.19 In fact, vertebral augmentation itself is somewhat controversial, with questions concerning a lack of defined indications, expected complications, outcome measures, and the need for long-term follow-up data.14
An open question in PVP is the mechanism of pain relief. The most intuitive explanation involves simple mechanical stabilization of the fracture. However, another possibility is the analgesic result from local chemical, vascular, or thermal effects of PMMA on nerve endings in surrounding tissue.13,20 Supporting this concept is the lack of correlation between cement volume and pain relief.21,22 Further evidence against an effect resulting solely from mechanical stabilization is the fact that PVP typically does not restore lost vertebral body height and therefore does not correct altered biomechanics.19,23
Technique
Injection of opacified PMMA is performed via a transpedicular or paravertebral approach under continuous fluoroscopic guidance to obtain adequate filling and to avoid PMMA leakage. For complex or high-risk cases, CT and fluoroscopic guidance are sometimes combined.10,19 In routine cases, PVP can be performed under local anesthesia with slight sedation in less than 1 hour,1 although general anesthesia is sometimes required because pain may intensify during cement injection.13 Preceding PMMA injection, intraosseous venography is often used to determine the filling pattern and identify sites of potential PMMA leakage (outline the venous drainage pattern, confirm needle placement within the bony trabeculae, and delineate fractures in the bony cortex). However, others have dispensed with routine venography.23
Contraindications to vertebroplasty include coagulopathy, absence of facilities to perform emergency decompressive surgery in the event of a complication, and extreme vertebral collapse (>65–70% reduction in vertebral height).13
Results
Searching the literature with the term percutaneous vertebroplasty in PubMed, there are 766 papers found from 1987 to March 2010.10,12,19,23–37 The first paper on vertebroplasty was published in 1987, treating C2 hemangioma in France.17 Reportedly, pain has been reduced in 70% to 90% of patients.
There are several case control studies published recently. Alvarez et al. conducted a prospective, double-cohort study,38 which consisted of 101 consecutive patients who underwent PVP and 27 patients who refused PVP treatment and were managed conservatively. The results showed that the patients elected for PVP had significantly more pain and functional impairment before the procedure than the patients in the group who did not have PVP (P < .001). The pain, functional, and general health scores of the PVP group were improved from the preoperative mean values (P < .001) in all postoperative periods. Compared with the conservative treatment group, there was a significant difference at 3 months. However, no statistical differences in function were observed between these groups at 6 months and 12 months postoperatively.
Diamond et al. designed a prospective, nonrandomized, “intention-to-treat” 2-year study.39 Participants included 126 consecutive patients (39 men and 87 women, 51 to 95 years of age) with acute osteoporotic vertebral fractures. Of these patients, 88 received treatment by PVP and 38 by conservative therapy. Outcomes in the PVP-treated patients showed 60% reduction in visual analogue pain scores from 20 to 8 (P < .001); a rapid return to normal function (29% improvement in physical functioning from 14 to 18 (P < .001); and lower rates of hospitalization (43% reduction in the mean number of hospital bed-days occupied), which were better than those treated conservatively (P < .001 for the comparison of all variables at 24 hours). Lower pain scores persisted in the vertebroplasty-treated group at 6 weeks (P < .001), but no differences between the two groups were evident at 12 and 24 months. In the vertebroplasty-treated group compared with the control group, the rates of new vertebral fractures (clinically and by radiographic assessment: hazard ratio, 1.13; 95% confidence interval [CI], 0.52–2.46; P = .76) and death (hazard ratio, 1.07; 95% CI, 0.42–2.76; P = .89) showed no significant difference.
Voormolen et al. prospectively assessed the short-term clinical outcome of patients with subacute or chronic painful osteoporotic VCFs treated with PVP compared with optimal pain medication (OPM) (VERTOS study sponsored by Vertos Medical Inc.).40 The investigators randomized the patients into two groups: treatment by PVP or OPM. After 2 weeks, patients from the OPM arm could change to the PVP arm. Patients were evaluated 1 day and 2 weeks after treatment. Their results showed that 18 patients treated with PVP, compared with 16 patients treated with OPM, had a significantly better Visual Analogue Scale (VAS) and used fewer analgesics 1 day after treatment. Two weeks after treatment, the mean VAS was less but not significantly different in patients treated with OPM, whereas these patients used significantly fewer analgesics and had better Quality of Life Questionnaire of the European Foundation for Osteoporosis (QUALEFO) and Roland-Morris Disability (RFD) scores. Scores in the PVP arm were influenced by occurrence of new VCF in two patients. After 2 weeks of OPM, 14 patients requested PVP treatment. All scores, 1 day and 2 weeks after PVP, were significantly better compared with scores during conservative treatment.
In Rousing’s randomized controlled study, 50 patients (41 females) were included from January 2001 until January 2008. Patients with acute (<2 weeks) and subacute (2–8 weeks) osteoporotic fractures were included and randomized to either PVP or conservative treatment. Their results showed reduction in pain from initial visit to 3-month follow-up was comparable in the two groups (P = .33); for approximate VAS, 8.0 to 2.0, intragroup difference was significant (P = .00). Reduction in pain in the PVP group was immediate 12 to 24 hours after the procedure (P = .00). There was no significant difference in the other parameters when comparing the results at inclusion and after 3 months within both groups and between the groups after 3 months. This study found two adjacent fractures in the PVP group and none in the conservative group. The investigators’ conclusions suggested that the majority of patients with acute or subacute painful osteoporotic compression fractures in the spine will recover after a few months of conservative treatment.
Recently, two new studies, reported in the New England Journal of Medicine,41,42 were multicenter, randomized controlled trials that used sham procedure as a control. In the Buchbinder study,41 the number of patients enrolled was 78, and 35 of 38 underwent PVP, and 36 of 40 underwent sham procedure in four Australian centers and completed the 6-month follow-up (91%). At 3 months, the mean reductions in the score for pain in the vertebroplasty and control groups were 2.6 ± 2.9 and 1.9 ± 3.3, respectively. Similar improvements were seen in both groups with respect to pain at night and at rest, physical functioning, quality of life, and perceived improvement. The researchers concluded that there was no beneficial effect of vertebroplasty compared with a sham procedure.
In another randomized, controlled trial, called the Investigational Vertebroplasty Safety and Efficacy Trial (INVEST) by Kallmes et al.,42 68 patients underwent PVP and 63 underwent sham procedure. Both groups had immediate improvement in disability and pain scores after the intervention. Although there was a trend toward a higher rate of clinically meaningful improvement in pain (a 30% decrease from baseline) in the vertebroplasty group (64% vs. 48%, P = .06), the two groups did not differ significantly on any secondary outcome measure at 1 month. The investigators also concluded that improvements in pain and pain-related disability associated with osteoporotic compression fractures in patients treated with vertebroplasty were similar to the improvements in a control group.
Complications
The principal risk of PVP, which involves the forced injection of low-viscosity PMMA cement into the closed space of the collapsed vertebral body, is cement extravasation. Extravasation rates are as high as 65% when cement is used to treat osteoporotic fractures.12,30 The likelihood is greater when using cement with a liquid rather than paste consistency or with higher PMMA volume.27 However, in most settings, the majority of extravasations have no clinical relevance, at least in the short term.23
The consequence of an extravasation depends on its location. In epidural or foraminal extravasation, nerve root compression and radiculopathy is the major risk. This occurred in 11 of 274 patients (4%) treated by Deramond.10 Three of those patients required surgical nerve root decompression. Others have been described with a 5% rate of radiculopathy, as well.9,21,43 Extravasation into perivertebral veins can cause cement embolism to the lungs; deaths attributed to cement embolism have been documented. However, two reported deaths attributed to pulmonary embolism were believed to be unrelated to the procedure; no cement material was detected by chest radiograph of the first patient,13,44 and the second pulmonary embolism arose from deep venous lower extremity thrombosis.10 On the other hand, extravasation into adjacent discs or paravertebral tissue, although common, generally produces no patient symptoms and carries little clinical significance; many such extravasations can be avoided by careful needle positioning.10
Other operative and long-term complications of PVP are specific to PMMA as a filler.18,23,45 The physician may work with PMMA in large batches to keep it liquid and to extend the working time for vertebroplasty. However, its high polymerization temperature (86–107°C within cement core)46 can damage adjacent tissue, including the spinal cord and nerve roots,15 leading to an inflammatory reaction and transitory exacerbation of pain.13 When injecting PMMA monomer, physician vigilance and caution are required. Absorption of PMMA monomer during the injection can induce hypotension by virtue of its cardiotoxic and dysrhythmogenic properties.47 Keeping in mind that placing a material in the spine affords proximity and access to the chest and the heart, vertebral augmentation with PMMA demands meticulous attention to technique.
Overall, the risk of complications that carry clinical significance following PVP for osteoporotic vertebral fracture is thought to be 1% to 3%, and most potential complications can be avoided with good technique.10
Kyphoplasty
Background
Kyphoplasty is an advanced surgical technique having evolved from a marriage of vertebroplasty with balloon angioplasty. It has a number of potential advantages, including lower risk of cement extravasation and better restoration of vertebral body height. A cannula is introduced into the vertebral body, via a transpedicular (Fig. 128-1) or extrapedicular route (Fig. 128-2), followed by insertion of an inflatable bone tamp (IBT; Fig. 128-3), which when deployed, reduces the compression fracture and restores the vertebral body toward its original height. This then creates a cavity to be filled with bone cement. The cement augmentation can now be completed with more control into the low-pressure environment of the preformed cavity with viscous, partially cured cement. Using a cannula for bone filler with a steel stylet as a plunger enables the operator to apply cement at considerably higher viscosity than is possible with injection through a 5-mL syringe and 11-gauge needle. Both the higher cement viscosity and controlled fill reduce the risk of cement extravasation. Filling is performed under continuous lateral fluoroscopic guidance similar to vertebroplasty. The procedure can be performed under general anesthesia or local anesthesia with intravenous sedation, and most of the patients are able to return home the same day of procedure.
Technique
With the patient under general or local anesthesia in the prone position on a radiolucent spinal frame, two C-arms are positioned for anteroposterior and lateral fluoroscopic images. Once positioned the C-arms and patient are not moved, to ensure repeatable images throughout the case. Two 3-mm incisions are made at the vertebral level, parallel to the pedicles in both planes. Then a guidewire or biopsy needle is advanced into the vertebral body via a transpedicular or extrapedicular approach, depending on the fracture configuration and the patient’s anatomy. The guidewire is then exchanged for the working cannula using a series of obturators. Once the working cannula is positioned, the surgeon reams out a corridor to accommodate the IBT and positions the IBT under the collapsed end plate (Fig. 128-4). To deploy the IBT, inflation proceeds slowly under fluoroscopy until maximum fracture reduction is achieved or the balloon reaches a cortical wall (Fig. 128-5). At this point, the surgeon deflates and removes the IBT, mixes the cement, prefills the cement cannulae, and allows the cement to partially cure in the cement cannulae. Once partially cured, PMMA is slowly extruded into the vertebral body through each pedicle under continuous lateral fluoroscopic guidance (Fig. 128-6). This technique permits a controlled fill. In most instances, the volume of cement can slightly exceed that of the bone cavity to interdigitate filler from the central bolus with the surrounding bone. Once filling is complete and the cement has hardened, the surgeon removes the cannula and closes the 3-mm incisions.
Results
Our published data from the Cleveland Clinic showed that kyphoplasty provided a safe and effective treatment for pain and disability in patients with vertebral compression fractures secondary to osteoporosis and multiple myeloma.16,48–51 Outcome data were obtained by administering the 36-Item Short Form Health Survey (SF-36) and the VAS for pain rating; in addition, the patients underwent detailed neurologic and radiographic examinations preoperatively and postoperatively. In our experience, there were no clinically significant cement leaks and no perioperative complications attributable to the IBT or tools. Using SF-36 data (preoperative and postoperative), available on more than 210 patients with average follow-up periods of 10.8 months, SF-36 scores improved in every category, statistically significant in all but the General Health modality. Physical function improved from 15.0 to 30.0 (P < .001). Role physical improved from 9.3 to 27.3 (P = .004). Bodily pain improved from 22.0 to 32.0 (P ≤ .001). Vitality improved from 30.0 to 40.0 (P ≤ .001). Social function improved from 37.5 to 50.0 (P ≤ .001). Role emotional improved from 33.3 to 66.7 (P = .39). Mental health improved from 64.0 to 72.0 (P < .001). General health was unchanged from 52.0 to 55.0 (P = .051). The VAS scores improved from a preoperative level of 7.0 to an initial postoperative level of 3.2 (P < .0001). At the last follow-up examination, the value remained unchanged at 3.4 (P < .0001).
Ledlie et al. reported functional and radiographic outcomes in the first 117 consecutive patients with 151 fractures.52 This report focused on the results for the subset of 77 patients completing follow-up. With regard to pain, rated by the patient using a 10-point VAS, the mean pain score decreased from 8.9 ± 1.5 before surgery to 2.8 ± 2.9 at 1 week postoperatively; this value remained low, at 1.5 ± 1.8 at 2 years postoperatively. The proportion of patients fully ambulatory increased from 45% before surgery to 85% at 1 week postoperatively and 88% at 2 years.
Garfin et al. reported clinical results of a total of 155 subjects with 214 VCFs who underwent balloon kyphoplasty at 19 study centers from August 2000 to June 2001.53 Mean pain ratings decreased from 15.0 (maximum score, 20) before surgery to 6.0 within 7 days after kyphoplasty (P < .001), a 60% reduction, and remained decreased throughout follow-up to 24 months. The mean number of days of the previous 28 days spent in bed decreased from 8.8 at baseline to 1.9, 2.2, 0.7, and 1.4 at 1, 3, 12, and 24 months, respectively, after kyphoplasty.
Wardlaw et al. reported the results of their randomized controlled trial at 21 sites in eight countries.54 In this report, 300 patients randomly assigned by a computer-generated sequence to receive kyphoplasty treatment (n = 149) or nonsurgical care (n = 151). Among them, 138 participants in the kyphoplasty group and 128 controls completed follow-up at 1 month. By use of repeated measures mixed effects modeling, all 300 randomized participants were included in the analysis. The mean SF-36 physical component summary score improved by 7.2 points (95% CI, 5.7–8.8), from 26.0 at baseline to 33.4 at 1 month, in the kyphoplasty group, and by 2.0 points (95% CI, 0.4–3.6), from 25.5 to 27.4, in the nonsurgical group (difference between groups 5.2 points, 95% CI, 2.9–7.4; P < .0001). The frequency of adverse events did not differ between groups. These results suggested that balloon kyphoplasty is an effective and safe procedure for patients with acute vertebral fractures and helps inform decisions regarding its use as an early treatment option.
In addition to good clinical results, height restoration by kyphoplasty has been reported in several studies (Fig. 128-7). Our initial results showed height restoration in 70% of 70 fractured vertebrae treated with kyphoplasty.16 In patients in whom the vertebral fractures were reduced by kyphoplasty, vertebral height increased by a mean of 46.8%.
Ledlie et al. reported their radiographic measurement results for ventral and midline points of the fractured vertebrae using the two nearest normal vertebrae as reference points.55 Pairs of preoperative and 2-year follow-up radiographs for 85 treated fractures were evaluated for vertebral height measurement. Mean preoperative ventral height (Ha), midheight (Hm), and dorsal height (Hp) were 61.3% ± 23.8%, 61.0% ± 20.1%, and 86.5% ± 18.4%, respectively. Compared to preoperative heights, mean Ha, Hm, and Hp were significantly higher (P < .001) at all postoperative intervals. Following kyphoplasty (1–6 weeks), mean Ha, Hm, and Hp were 81.2% ± 20.9%, 86.9% ± 17.9%, and 95.1% ± 10.1%, respectively. At 2-year follow-up, mean Ha, Hm, and Hp were 80.9% ± 19.8%, 88.1% ± 16.6%, and 91.4% ± 12.8%, respectively. There was no correlation between height increase and patient age, gender, fracture level, or fracture age.52
Garfin et al. reported the results of midline height restoration rates. The mean preoperative midvertebral height was 65% of the predicted height. Eighty-two percent of patients had at least 10% lost height restored during the pretreatment and post-treatment interval. The mean percent midvertebral lost height restored was 32% overall and 44% in those with measurable height restoration.53
Complications
Many kyphoplasty studies provided details on the number of cement leakage complications. In a meta-analysis, Taylor showed that a total of 189 (9%) cement leakages were reported in 2239 vertebrae that underwent kyphoplasty.56 This corresponds to 81 cement leaks per 1000 fractures undergoing kyphoplasty per year. One leak (0.001%) was reported by Majd57 to be symptomatic L1 radiculopathy. A few cases also reported inappropriate cement injection and needle placement in the early phase.58 In our series of patients,16,49 cement extravasation was seen in less than 10% of cases. No problems were identified clinically as a result of these extravasations immediately after surgery or at final follow-up. In one patient, a myocardial infarction occurred as a result of fluid overload during the procedure.
Ledlie et al. reported three complications in their series.52 Two weeks after kyphoplasty, a patient with a history of deep vein thrombosis (no evidence of PMMA leakage to the lungs by CT) had a pulmonary embolism. A second patient with preexisting metastatic, cardiac, vascular, severe chronic obstructive pulmonary disease required mechanical ventilation and subsequently died 5 days postoperatively. A third patient had perioperative confusion and generalized weakness that gradually resolved (negative brain CT and neurologic workup). No complication was related to the kyphoplasty technique. Minor cement extravasations were observed in 11.3% (17/151) of treated fractures, but none of the leaks was associated with any clinical consequence.
Garfin et al. also reported that the extravasation of PMMA outside the vertebral body occurred in 21/214 (10%) of treated levels.53 All PMMA extravasations were asymptomatic, the cement remained in the immediate area of the treated vertebrae, and no medical or surgical intervention was required to remove the extravasated PMMA.
Phillips et al. reported that asymptomatic cement leaks were observed in 6 of 61 vertebral fractures (9.8%).59 In this series as well, there were no clinical consequences attributable to the bone tamp or cement deposition.
Two papers reported “redo” vertebral augmentation in case the previous vertebral segmentation failed to relieve pain.60,61 A report by Gaughen et al. concerned six patients whose initial vertebroplasty resulted in substantial improvement in pain. These six patients developed recurrent pain between 8 days and 167 days after initial vertebroplasty and underwent “redo” vertebroplasty. After repeat vertebroplasty, five of the six patients reported a reduction of at least 3 points in their pain rating, with a mean reduction of 6.5 points and a mean postoperative pain level of 3.5 points (11-point scale). Four of six patients reported impaired mobility before repeat vertebroplasty, and all four demonstrated a postoperative improvement in mobility. The mean increase in mobility was 1.50 points, and the mean postoperative mobility impairment was 0.25 points (5-point scale). The investigators concluded that the clinical outcomes of the patients within this case series suggest that repeat percutaneous vertebroplasty performed at previously treated vertebral levels for recurrent pain offers therapeutic benefit. Although the repeating of this procedure is technically demanding, “redo” vertebral augmentation could be performed over a previously treated kyphoplasty or vertebroplasty.
Mechanism of Pain Relief
The etiology of pain after an osteoporotic or an osteolytic vertebral collapse is multivariate (biomechanical, physiologic, or neurogenic). Although a number of reports describing clinical results of vertebral augmentation indicate good pain relief, the mechanism of this relief remains unclear. The most intuitive explanation involves simple mechanical stabilization of the fracture; the cement stabilizes the vertebral bodies and offloads the facet joints. However, another explanation is that analgesia results from local chemical, vascular, or thermal effects of PMMA on nerve endings in surrounding tissue. Supporting this concept is the lack of correlation between cement volume and pain relief. Further evidence against an effect resulting solely from mechanical stabilization is the fact that PVP typically does not restore lost vertebral body height and therefore does not correct altered biomechanics. Another potential mechanism of pain relief involves the normalization of strains through the centrum and away from the cortices of the vertebral body. It has been shown that with osteoporosis, the centrum of the vertebral body becomes deficient and the axial load is transmitted to the vertebral body walls. By augmenting the centrum with or without a sagittal realignment, the force transmission may now be normalized.62 The contribution of metabolic bone disease such as osteomalacia to the pain remains unclear.
Biomechanics
The ability for organ systems to compensate for changes diminishes as humans age. The skeletal system and spinal column also become more vulnerable to fractures with increased age. There is a significant decrease in vertebral body strength with aging, resulting from the loss of cancellous bone support.63,64 The compressive strength of any bone is dependent on numerous factors, including the species, site, health status, and quality of bone. The compressive strength of normal human cancellous bone shows great variation but typically resists 5 megapascals (MPa) or 725 pounds of load per square inch (psi).65 Osteoporotic vertebral bone exhibits compressive strength in the lower region of this range (<2 MPa, or 290 psi), whereas younger, healthier, or cancellous bone from other sites may exhibit compressive strength in the upper region of this range. Carter and Hayes showed a strong correlation between bone density and the strength of cancellous bone.66 The compressive strength of trabecular bone increases approximately with the square of its density. Therefore, doubling the density of a given bone results in a fourfold increase in compressive strength. In contrast, if the density of the bone decreases by 50%, the remaining compressive strength is only 25% of the original value.67 Bell also reported a direct correlation between bone loss and strength, noting that a decrease of 25% in the osseous structure resulted in a 50% decrease in vertebral strength.63 Rockoff et al. further characterized the effects of age on loss of bone strength by analyzing the cancellous or trabecular bone’s ability to compensate for applied forces.68 The cancellous vertebral body bone carries approximately 55% of axial loads in the adult spine before the age of 40 years, but this percentage declines to only 35% after the age of 40 years. These changes in bone density are directly responsible for the 4% annual incidence of VCFs in postmenopausal women (primary osteoporosis).69 These fractures can result in functional limitations such as a decrease in gait velocity, an increase in muscle fatigue, and additional falls.
The thoracic and thoracolumbar regions of the spine have a natural kyphotic curvature. This curvature biomechanically places the ventral thoracic spine at an increased risk for developing compression fractures as a consequence of axial loads.70–72 The kyphotic curvature concentrates the applied axial load on the ventral portion of the vertebral body. These axial loads cause all points ventral to the vertebral body (instantaneous axis of rotation; IAR) to come closer together, while simultaneously all points dorsal to the IAR are spread apart.73 If the failure point of the vertebral body is exceeded, a compression fracture occurs. In younger patients, the forces required to produce a fracture are significant and typically result from high-energy trauma. However, as the vertebral body is weakened by osteoporosis, the amount of energy required to initiate a fracture significantly decreases. Once a VCF occurs, the transmission of forces through the vertebral column is altered. A VCF reduces the load-carrying capacity of the anterior column and causes the IAR to migrate dorsally to the region with intact supporting structures.74 The dorsal migration of the IAR causes the previous mechanical advantage of a longer lever arm, from which the dorsal ligaments and muscles acted to maintain sagittal balance, to be shortened. The dorsal displacement of the IAR simultaneously causes the distance from the ventrally located center of gravity to the IAR to be greater, which places additional distraction on the posterior columns and compression on the anterior columns.72,74
Kayanja et al. investigated the distribution of ventral cortical strain at, above, and below an experimentally created index VCF to determine the vertebral body at risk of secondary fracture.62 In this study, 17 human cadaveric thoracic vertebrae were divided into multilevel segments composed of three vertebrae (T1-3, T4-6, T7-9, and T10-12). Measurements of ventral cortical shear strain, applied moments, and applied flexion angle were made in compression and flexion. The results showed that the shear strain distribution was independent of the location of the multilevel segment level in the spine and was highest at the apex of a thoracic index VCF. The vertebra above the index VCF had increased ventral cortical shear strain and was therefore at greatest risk for secondary fracture. These results suggest that restoration of sagittal alignment minimizes strain on the vulnerable vertebral levels above an index VCF, preventing subsequent vertebral fractures. Kayanja et al. also determined the effects of load (compression and flexion) on the adjacent levels (above and below) an augmented VCF.75 Six upper thoracic segments (T1-5) and six lower thoracic segments (T8-12) were biomechanically tested creating VCFs that were subsequently augmented in the intermediate vertebrae T3 and T10. Multilevel segment stiffness and adjacent vertebral strain at superior and inferior levels were measured before and after the vertebral augmentation. The results showed that VCF and augmentation reduced compressive and bending stiffness in the vertebral segment, while the adjacent vertebral strain increased. The augmentation also caused a greater amount of strain on the inferior adjacent level compared to the superior level. These results suggested that cement augmentation reduces stiffness while increasing adjacent level strain primarily on the inferior adjacent vertebra, and this alteration in strain distribution probably spares the superior adjacent vertebra from fracture.
Filler Materials
The filling materials that are used for vertebral augmentation require good biocompatibility, good biomechanical strength and stiffness, and good radiopacity for fluoroscopy-guided procedures.76
PMMA has been the material most commonly used during vertebral augmentation procedures.77 In April 2004, the Food and Drug Administration approved the labeling of certain brands of PMMA for the treatment of pathologic fractures of the vertebral body due to osteoporosis and tumor using a kyphoplasty technique.78 PMMA is reportedly bioinert and shows good biocompatibility over long-term follow-up. Several inherent advantages to PMMA include familiarity for orthopaedic surgeons, ease of handling, good biomechanical strength and stiffness, and cost effectiveness. Some disadvantages include no biologic potential to remodel or integrate into the surrounding bone, no direct bone apposition, excessive inherent stiffness, high polymerization temperature, and potential monomer toxicity. Although good clinical results have been reported in several series of both vertebroplasty and kyphoplasty procedures,12,16,30,48,49,55,58,59,79 it is still unclear whether some component of the pain relief is secondary to the mechanical stabilization, chemical toxicity, or thermal necrosis of surrounding tissues and nerve ends. To date, the concern regarding thermal bone necrosis is still theoretical, and there has been no obvious evidence to support such a concern.80,81
Calcium phosphate cement offers the potential for resorption of the cement over time and replacement with new bone as a biologic method to restore vertebral body mass and avoid any potential thermal effects of PMMA.82–86 This material is also expected to work as an optimum carrier for osteoinductive proteins.87 Preclinical animal studies and human pilot studies have shown that these calcium phosphate cements are highly osteoconductive and undergo gradual remodeling with time.88–93 There are only a few published manuscripts reporting histologic data with calcium phosphate cement in a vertebroplasty model.82,94–96 In general, the cement undergoes resorption and remodeling, which was apparent as fragmentation with vascular invasion and bone ingrowth into the material. The reports also described evidence of osteoclastic resorption of the cement and direct bone apposition in a pattern that suggested remodeling similar to that of normal bone. Turner et al. tested both PMMA and calcium phosphate cement (BoneSource, Stryker Orthopaedics, Mahwah, NJ) in a canine vertebral body defect. In this study, both materials were well integrated histologically, but calcium phosphate underwent resorption and remodeling, and it demonstrated excellent biocompatibility and osteoconductivity.95 Takikawa et al. also reported greater than 80% direct apposition to cancellous bone in postoperative osteopenic sheep vertebrae at 3, 6, 12, and 24 months.96 A number of hydroxyapatite and calcium phosphate cements also have been biomechanically tested in vitro45,97,98; most are able to restore mechanical integrity to the vertebral body.99–101
Calcium sulfate, more commonly known as plaster of Paris, has a long clinical history for use as a bone graft substitute in various skeletal sites. This material is injectable, osteoconductive, and cures with a limited exothermic reaction.102,103 Calcium sulfate paste has also been reported to significantly augment pull-out strength when used for augmentation of pedicle screw fixation.104 However, this material is rapidly resorbed,105–107 and it may not be able to support spinal alignment while it is remodeling; therefore, it would likely be inappropriate for use in a vertebral augmentation procedure.
Composite materials (acrylic cements in conjunction with ceramics) are bioactive, highly radiopaque, and feature excellent mechanical properties.108,109 One such material, terpolymer resin reinforced with combeite glass-ceramic particles (Cortoss, Orthovita, Malvern, PA), is currently undergoing clinical trials for vertebroplasty and kyphoplasty in the United States and has been reported to be a potential alternative to PMMA. Cortoss was approved for vertebroplasty in Europe in January 2003 based on the results of a prospective clinical trial conducted in Europe.110 Its osteoconductivity has been proven in several animal models,109,111 but no human histology has been reported to date.
Biopsy Results
A diagnostic bone biopsy can be easily performed during a kyphoplasty procedure and does not affect the safety of the procedure if done appropriately. We histologically evaluated 178 biopsies obtained from 142 patients during 246 kyphoplasty procedures112 (Figs. 128-8 and 128-9). These showed partially necrotic fragments of bone as well as areas of fibrosis and variable stages of woven bone, suggesting ongoing fracture healing. Specimens obtained from 30 patients (21%) showed markedly increased osteoid in undecalcified sections. These thickened osteoid seams may suggest a possible mineralization defect (osteomalacia). Osteoid can be increased either because of increased bone remodeling activity or a mineralization defect. Tetracycline labeling is the only way to distinguish between these two diagnoses. Careful administration of tetracycline labels may help identify any correlation between vertebral fracture and osteomalacia. Also in this series, four biopsies provided a definite diagnosis of plasma cell dyscrasia in otherwise unsuspected or unknown spinal lesions. These findings suggest that a biopsy is useful for all initial vertebral augmentation cases to rule out any occult lesions.
Histology of Vertebral Augmentation
In spite of reported good clinical results, several aspects of the vertebral augmentation procedure are controversial, including the optimum methods of mixing and depositing the cement, the potential importance of a foreign body reaction at the cement-bone interface, efficacy of bone tamp usage, the use of relatively high concentrations of radiopaque agents and antibiotics in the cement, and the clinical indications for the procedure. We were able to document histologically four vertebral bodies from two cases 1 month and 2 years after cement augmentation, after a surgical corpectomy and at an autopsy80 (Fig. 128-10). In this study, the histology of vertebrae treated using the kyphoplasty technique revealed a dense cancellous shell around the cement mantle. This suggests that the tamping had displaced bone, essentially autografting the space around the cement. Bone immediately around the cement did not show extensive necrosis. However, foreign body giant cells contained material consistent with cement particles and/or barium sulfate. Particles were also identified within vascular spaces (Fig. 128-11).
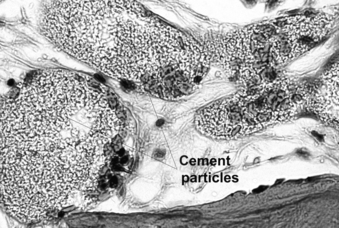
FIGURE 128-11 Cement particles and/or barium sulfate were found within vascular spaces of the vertebral body in an autopsy specimen.
In a baboon vertebral augmentation study, the same histologic features were observed in the vertebrae treated with both vertebroplasty and kyphoplasty.81 There was evidence of a foreign body reaction to PMMA and a few necrotic segments of bone present in both the vertebroplasty and kyphoplasty vertebrae. However, it was not clear that the necrosis was caused by the PMMA polymerization process or bone tamping procedures. Further histologic evaluation may help clarify the safety and efficacy of vertebral augmentation.
Adjacent and Remote Fractures
One theoretical issue continuously raised by spine practitioners concerns the incidence of remote and adjacent-level vertebral compression fractures after an index VCF has been augmented by either vertebroplasty or kyphoplasty. Keller et al. investigated the biomechanics of age-related spine deformity using a sagittal plane biomechanical model and showed that postural forces were responsible for initiation and propagation of osteoporotic spine deformity in the elderly.113 Kayanja et al. reported results of biomechanical tests for cadaveric thoracic wedge compression fractures and showed that ventral cortical strain was maximum at the apex of a thoracic kyphotic curve and that the vertebral body immediately above it had the next highest strain, with an increased risk of secondary fracture.62 Moreover, in a 10-year Swedish population–based cohort study of 598 individuals, Hasserius et al. suggested that a prevalent vertebral deformity could predict both increased mortality and increased fracture incidence during the following decade in both men and women.114 Left untreated, the incidence of subsequent vertebral fracture after an index fracture is reported in other studies as approximately 20%.69,115
There was no consistent rate of subsequent fracture after vertebroplasty. From the vertebroplasty literature, one study reported a 52% rate of remote or adjacent-level fractures after vertebroplasty.33 A second study reported a 12% rate of remote fractures (one third) and adjacent-level fractures (two thirds).116
From the kyphoplasty literature, Harrop et al. reported that the incidence of postkyphoplasty VCF in primary osteoporotic patients was 11.25% (9 fractures/80 patients), whereas the incidence in steroid-induced osteoporotic patients was 48.6% (17 fractures/35 patients).117 These results imply that the intervention, kyphoplasty, in primary osteoporotic patients does not increase the rate of remote or adjacent-level fractures compared with the published natural history reports. These results also imply that compared with primary osteoporotic patients, secondary osteoporotic patients are in fact at increased risk for subsequent VCFs, although there is no natural history benchmark to which we can compare this rate. Fribourg et al. also reported the results of retrospective review of charts and radiographs of patients who underwent kyphoplasty.118 In 38 patients with 47 treated levels, 10 patients sustained 17 subsequent fractures in this study (9 above adjacent-level, 4 below adjacent-level, 4 at remote levels). Although this report is informative, 15 patients in this series had known oncologic disease, and 7 patients had steroid use, with known increased risk of subsequent fractures.
Osteolytic Vertebral Compression Fractures
Osteolytic destruction of the vertebral bodies secondary to metastatic disease or multiple myeloma affects up to 70% of cancer patients.119,120 Pain is the initial complaint in up to 95% of patients despite the fact that more than 50% of spinal metastases are asymptomatic.119,120 Only 10% of patients with spinal metastases present with neurologic signs or symptoms on initial presentation.12 With modern advances in oncologic treatment, patient survival has improved to the point where disease that was once life-threatening can now be considered chronic. Unfortunately, the oncologic treatment itself also contributes to the already present osteolytic bone loss. With the increased survival and ongoing bone loss, osteolytic VCFs are becoming much more of a clinical and functional problem for affected patients. The indications for surgical intervention are reported as being progressive collapse, neurologic deterioration, and/or intractable pain. However, surgical intervention in this patient group is typically considered to be very difficult by virtue of comorbid conditions and poor bone quality. Similar to the scenario with osteoporotic compression fractures, possible complications associated with these osteolytic fractures include cord compression, urinary retention, ileus, intractable pain, and pulmonary compromise (9% loss in predicted forced vital capacity with each vertebral fracture).121,122 Other chronic sequelae include deconditioning, deformity, insomnia, and depression, resulting in substantial physical, functional, and psychosocial impairment. Recently, to alleviate these issues, percutaneous minimally invasive vertebral augmentation techniques have evolved and show promising preliminary results for these debilitated patients.
Multiple Myeloma
Multiple myeloma is a monoclonal proliferation of malignant plasma cells that usually affects the bone marrow. Excessive bone resorption due to an increase of proinflammatory cytokines is a characteristic feature of the disease.123 This tumor is grossly a very soft vascular tumor, as evidenced by the backflow of blood from the working cannulae during kyphoplasty. The near-fluid consistency of the tumor and the lytic nature of the bone make it easy for the IBT to displace tissue in the act of reducing the fracture and creating the cavity. This then results in impressive cement filling of the vertebra. Dudeney et al. reported satisfying results in the treatment of osteolytic VCFs due to multiple myeloma.48 Fifty-five consecutive kyphoplasty procedures were performed over 27 sessions in 18 patients. The mean age of patients was 63.5 (48–79) years, the mean duration of symptoms was 11 months, and the mean follow-up was 7.4 months. There were no major complications related directly to use of this technique. On average, 34% of height loss at the time of fracture was restored. After stratifying for patients whose height was not restored, the remaining vertebral bodies showed an average of 56% height restoration. Asymptomatic cement leakage occurred at 2/55 levels (4%). Significant improvement in SF-36 scores occurred for bodily pain (23.2 to 55.4, P = .0008), physical function (21.3 to 50.6, P = .0010), vitality (31.3 to 47.5, P = .010), and social functioning (40.6 to 64.8, P = .014). The authors concluded that the kyphoplasty technique (1) was efficacious in the treatment of osteolytic VCFs due to multiple myeloma and (2) was associated with early clinical improvement of pain and function as well as some restoration of vertebral body height in these patients. The effects of potential tumor dissemination, in what is already widespread disease, are unknown. Any significant systemic effects are not suspected and have not been noted in the initial study group.48 Lane et al. also prospectively evaluated 19 patients with multiple myeloma treated with kyphoplasty.124 They reported significant improvement in functional status measured by the Oswestry Disability Index (ODI) score, with significant vertebral height restoration. In their comparison, there was no significant difference between the myeloma patient group and the osteoporotic compression fracture group in terms of either ODI score or vertebral height restoration.
Other Tumors
In an ongoing evaluation of nonmyelomatous osteolytic collapse, the results have remained very favorable. From April 1999 to April 2004, 21 patients with nonmyelomatous osteolytic vertebral collapse (9 breast, 4 leukemia/lymphoma, 3 lung, 1 pharyngeal basal cell, 4 unknown origin) underwent 42 kyphoplasty procedures over 24 sessions.125 The procedure was successfully performed in all patients. The perioperative and clinical follow-up revealed that the procedure was well tolerated, with improvement in pain and early mobilization. The patients reported statistically significant improvements in their objective outcome scores (SF-36). Barr et al. also reported that the results with osteolytic metastatic vertebral collapse in eight patients revealed that only four patients experienced any pain relief. In this group of patients, there was a 6% complication rate.19
Conclusions
Although these two minimally invasive techniques are shown to be quite beneficial for the VCF patients, controversy continues regarding the optimum timing of these surgeries and their true effectiveness and cost effectiveness over the long term, especially since the two randomized controlled studies were published in the New England Journal of Medicine41,42 Several ongoing randomized clinical trials would provide further information in the future.
Buchbinder R., Osborne R.H., Ebeling P.R., et al. A randomized trial of vertebroplasty for painful osteoporotic vertebral fractures. N Engl J Med. 2009;361:557-568.
Garfin S.R., Buckley R.A., Ledlie J. Balloon kyphoplasty for symptomatic vertebral body compression fractures results in rapid, significant, and sustained improvements in back pain, function, and quality of life for elderly patients. Spine (Phila Pa 1976). 2006;31:2213-2220.
Kallmes D.F., Comstock B.A., Heagerty P.J., et al. A randomized trial of vertebroplasty for osteoporotic spinal fractures. N Engl J Med. 2009;361:569-579.
Lieberman I.H., Dudeney S., Reinhardt M.K., et al. Initial outcome and efficacy of “kyphoplasty” in the treatment of painful osteoporotic vertebral compression fractures. Spine (Phila Pa 1976). 2001;26:1631-1638.
Lindsay R., Silverman S.L., Cooper C., et al. Risk of new vertebral fracture in the year following a fracture. JAMA. 2001;285:320-323.
Wardlaw D., Cummings S.R., Van Meirhaeghe J., et al. Efficacy and safety of balloon kyphoplasty compared with non-surgical care for vertebral compression fracture (FREE): a randomised controlled trial. Lancet. 2009;373:1016-1024.
1. Johnell O., Kanis J.A. An estimate of the worldwide prevalence and disability associated with osteoporotic fractures. Osteoporos Int. 2006;17:1726-1733.
2. Incidence of vertebral fracture in Europe. results from the European Prospective Osteoporosis Study (EPOS). J Bone Miner Res. 2002;17:716-724.
3. Carmona R.H. Office of the Surgeon General: Bone health and osteoporosis: a report of the Surgeon General. Rockville, MD: Department of Health and Human Services; 2004.
4. Lyritis G.P., Mayasis B., Tsakalakos N., et al. The natural history of the osteoporotic vertebral fracture. Clin Rheumatol. 1989;8(Suppl 2):66-69.
5. Hasserius R., Karlsson M.K., Jonsson B., et al. Long-term morbidity and mortality after a clinically diagnosed vertebral fracture in the elderly—a 12- and 22-year follow-up of 257 patients. Calcif Tissue Int. 2005;76:235-242.
6. Cranney A., Wells G., Willan A., et al. Meta-analyses of therapies for postmenopausal osteoporosis. II. Meta-analysis of alendronate for the treatment of postmenopausal women. Endocr Rev. 2002;23:508-516.
7. Cranney A., Tugwell P., Adachi J., et al. Meta-analyses of therapies for postmenopausal osteoporosis. III. Meta-analysis of risedronate for the treatment of postmenopausal osteoporosis. Endocr Rev. 2002;23:517-523.
8. Patel U., Skingle S., Campbell G.A., et al. Clinical profile of acute vertebral compression fractures in osteoporosis. Br J Rheumatol. 1991;30:418-421.
9. Bostrom M.P., Lane J.M. Future directions. Augmentation of osteoporotic vertebral bodies. Spine (Phila Pa 1976). 1997;22:S38-S42.
10. Deramond H., Depriester C., Galibert P., Le Gars D. Percutaneous vertebroplasty with polymethylmethacrylate. Technique, indications, and results. Radiol Clin North Am. 1998;36:533-546.
11. Chiras J., Depriester C., Weill A., et al. Percutaneous vertebral surgery. Technics and indications. J Neuroradiol. 1997;24:45-59.
12. Jensen M.E., Evans A.J., Mathis J.M., et al. Percutaneous polymethylmethacrylate vertebroplasty in the treatment of osteoporotic vertebral body compression fractures: technical aspects. AJNR Am J Neuroradiol. 1997;18:1897-1904.
13. Cotten A., Boutry N., Cortet B., et al. Percutaneous vertebroplasty: state of the art. Radiographics. 1998;18:311-320. discussion 320–323
14. Einhorn T.A. Vertebroplasty: an opportunity to do something really good for patients. Spine (Phila Pa 1976). 2000;25:1051-1052.
15. Deramond H., Wright N.T., Belkoff S.M. Temperature elevation caused by bone cement polymerization during vertebroplasty. Bone. 1999;25:S17-S21.
16. Lieberman I.H., Dudeney S., Reinhardt M.K., Bell G. Initial outcome and efficacy of “kyphoplasty” in the treatment of painful osteoporotic vertebral compression fractures. Spine (Phila Pa 1976). 2001;26:1631-1638.
17. Galibert P., Deramond H., Rosat P., Le Gars D. Preliminary note on the treatment of vertebral angioma by percutaneous acrylic vertebroplasty. Neurochirurgie. 1987;33:166-168.
18. Bai B., Jazrawi L.M., Kummer F.J., Spivak J.M. The use of an injectable, biodegradable calcium phosphate bone substitute for the prophylactic augmentation of osteoporotic vertebrae and the management of vertebral compression fractures. Spine (Phila Pa 1976). 1999;24:1521-1526.
19. Barr J.D., Barr M.S., Lemley T.J., McCann R.M. Percutaneous vertebroplasty for pain relief and spinal stabilization. Spine (Phila Pa 1976). 2000;25:923-928.
20. Mathis J.M., Petri M., Naff N. Percutaneous vertebroplasty treatment of steroid-induced osteoporotic compression fractures. Arthritis Rheum. 1998;41:171-175.
21. Cotten A., Dewatre F., Cortet B., et al. Percutaneous vertebroplasty for osteolytic metastases and myeloma: effects of the percentage of lesion filling and the leakage of methyl methacrylate at clinical follow-up. Radiology. 1996;200:525-530.
22. Dean J.R., Ison K.T., Gishen P. The strengthening effect of percutaneous vertebroplasty. Clin Radiol. 2000;55:471-476.
23. Heini P.F., Walchli B., Berlemann U. Percutaneous transpedicular vertebroplasty with PMMA: operative technique and early results. A prospective study for the treatment of osteoporotic compression fractures. Eur Spine J. 2000;9:445-450.
24. Cotten A., Duquesnoy B. Vertebroplasty: current data and future potential. Rev Rhum Engl Ed. 1997;64:645-649.
25. Cortet B., Cotten A., Boutry N., et al. Percutaneous vertebroplasty in patients with osteolytic metastases or multiple myeloma. Rev Rhum Engl Ed. 1997;64:177-183.
26. Weill A., Chiras J., Simon J.M., et al. Spinal metastases: indications for and results of percutaneous injection of acrylic surgical cement. Radiology. 1996;199:241-247.
27. Martin J.B., Jean B., Sugiu K., et al. Vertebroplasty: clinical experience and follow-up results. Bone. 1999;25:S11-S15.
28. Evans A.J., Jensen M.E., Kip K.E., et al. Vertebral compression fractures: pain reduction and improvement in functional mobility after percutaneous polymethylmethacrylate vertebroplasty: retrospective report of 245 cases. Radiology. 2003;226:366-372.
29. Cohen J.E., Lylyk P., Ceratto R., et al. Percutaneous vertebroplasty: technique and results in 192 procedures. Neurol Res. 2004;26:41-49.
30. Cortet B., Cotten A., Boutry N., et al. Percutaneous vertebroplasty in the treatment of osteoporotic vertebral compression fractures: an open prospective study. J Rheumatol. 1999;26:2222-2228.
31. Cyteval C., Sarrabere M.P., Roux J.O., et al. Acute osteoporotic vertebral collapse: open study on percutaneous injection of acrylic surgical cement in 20 patients. AJR Am J Roentgenol. 1999;173:1685-1690.
32. Maynard A.S., Jensen M.E., Schweickert P.A., et al. Value of bone scan imaging in predicting pain relief from percutaneous vertebroplasty in osteoporotic vertebral fractures. AJNR Am J Neuroradiol. 2000;21:1807-1812.
33. Grados F., Depriester C., Cayrolle G., et al. Long-term observations of vertebral osteoporotic fractures treated by percutaneous vertebroplasty. Rheumatology (Oxford). 2000;39:1410-1414.
34. Kim A.K., Jensen M.E., Dion J.E., et al. Unilateral transpedicular percutaneous vertebroplasty: initial experience. Radiology. 2002;222:737-741.
35. Kaufmann T.J., Jensen M.E., Schweickert P.A., et al. Age of fracture and clinical outcomes of percutaneous vertebroplasty. AJNR Am J Neuroradiol. 2001;22:1860-1863.
36. Ryu K.S., Park C.K., Kim M.C., Kang J.K. Dose-dependent epidural leakage of polymethylmethacrylate after percutaneous vertebroplasty in patients with osteoporotic vertebral compression fractures. J Neurosurg. 2002;96:56-61.
37. McKiernan F., Faciszewski T., Jensen R. Quality of life following vertebroplasty. J Bone Joint Surg [Am]. 2004;86:2600-2606.
38. Alvarez L., Alcaraz M., Perez-Higueras A., et al. Percutaneous vertebroplasty: functional improvement in patients with osteoporotic compression fractures. Spine (Phila Pa 1976). 2006;31:1113-1118.
39. Diamond T.H., Bryant C., Browne L., Clark W.A. Clinical outcomes after acute osteoporotic vertebral fractures: a 2-year non-randomised trial comparing percutaneous vertebroplasty with conservative therapy. Med J Aust. 2006;184:113-117.
40. Voormolen M.H., Mali W.P., Lohle P.N., et al. Percutaneous vertebroplasty compared with optimal pain medication treatment: short-term clinical outcome of patients with subacute or chronic painful osteoporotic vertebral compression fractures. The VERTOS study. AJNR Am J Neuroradiol. 2007;28:555-560.
41. Buchbinder R., Osborne R.H., Ebeling P.R., et al. A randomized trial of vertebroplasty for painful osteoporotic vertebral fractures. N Engl J Med. 2009;361:557-568.
42. Kallmes D.F., Comstock B.A., Heagerty P.J., et al. A randomized trial of vertebroplasty for osteoporotic spinal fractures. N Engl J Med. 2009;361:569-579.
43. Kuivaniemi H., Tromp G., Prockop D.J. Mutations in collagen genes: causes of rare and some common diseases in humans. Faseb J. 1991;5:2052-2060.
44. Padovani B., Kasriel O., Brunner P., Peretti-Viton P. Pulmonary embolism caused by acrylic cement: a rare complication of percutaneous vertebroplasty. AJNR Am J Neuroradiol. 1999;20:375-377.
45. Cunin G., Boissonnet H., Petite H., et al. Experimental vertebroplasty using osteoconductive granular material. Spine (Phila Pa 1976). 2000;25:1070-1076.
46. Leeson M.C., Lippitt S.B. Thermal aspects of the use of polymethylmethacrylate in large metaphyseal defects in bone. A clinical review and laboratory study. Clin Orthop Relat Res. 1993;295:239-245.
47. Phillips H., Cole P.V., Lettin A.W. Cardiovascular effects of implanted acrylic bone cement. Br Med J. 1971;3:460-461.
48. Dudeney S., Lieberman I.H., Reinhardt M.K., Hussein M. Kyphoplasty in the treatment of osteolytic vertebral compression fractures as a result of multiple myeloma. J Clin Oncol. 2002;20:2382-2387.
49. Coumans J.V., Reinhardt M.K., Lieberman I.H. Kyphoplasty for vertebral compression fractures: 1-year clinical outcomes from a prospective study. J Neurosurg. 2003;99:44-50.
50. Khanna A.J., Reinhardt M.K., Togawa D., et al. Functional outcomes of kyphoplasty for the treatment of osteoporotic and osteolytic vertebral compression fractures. Osteoporos Int. 2006;17:817-826.
51. Lieberman I., Reinhardt M.K. Vertebroplasty and kyphoplasty for osteolytic vertebral collapse. Clin Orthop Relat Res. 2003;415(suppl):S176-S186.
52. Ledlie J.T., Renfro M.B. Kyphoplasty treatment of vertebral fractures: 2-year outcomes show sustained benefits. Spine (Phila Pa 1976). 2006;31:57-64.
53. Garfin S.R., Buckley R.A., Ledlie J. Balloon kyphoplasty for symptomatic vertebral body compression fractures results in rapid, significant, and sustained improvements in back pain, function, and quality of life for elderly patients. Spine (Phila Pa 1976). 2006;31:2213-2220.
54. Wardlaw D., Cummings S.R., Van Meirhaeghe J., et al. Efficacy and safety of balloon kyphoplasty compared with non-surgical care for vertebral compression fracture (FREE): a randomised controlled trial. Lancet. 2009;373:1016-1024.
55. Ledlie J.T., Renfro M. Balloon kyphoplasty: one-year outcomes in vertebral body height restoration, chronic pain, and activity levels. J Neurosurg. 2003;98:36-42.
56. Taylor R.S., Fritzell P., Taylor R.J. Balloon kyphoplasty in the management of vertebral compression fractures: an updated systematic review and meta-analysis. Eur Spine J. 2007;16:1085-1100.
57. Majd M.E., Farley S., Holt R.T. Preliminary outcomes and efficacy of the first 360 consecutive kyphoplasties for the treatment of painful osteoporotic vertebral compression fractures. Spine J. 2005;5:244-255.
58. Garfin S.R., Yuan H.A., Reiley M.A. New technologies in spine: kyphoplasty and vertebroplasty for the treatment of painful osteoporotic compression fractures. Spine (Phila Pa 1976). 2001;26:1511-1515.
59. Phillips F.M., Ho E., Campbell-Hupp M., et al. Early radiographic and clinical results of balloon kyphoplasty for the treatment of osteoporotic vertebral compression fractures. Spine (Phila Pa 1976). 2003;28:2260-2265.
60. Gaughen J.R.Jr., Jensen M.E., Schweickert P.A., et al. The therapeutic benefit of repeat percutaneous vertebroplasty at previously treated vertebral levels. AJNR Am J Neuroradiol. 2002;23:1657-1661.
61. Frey M.E. Redo kyphoplasty with vertebroplasty technique: a case report and review of the literature. Pain Physician. 2009;12:645-649.
62. Kayanja M.M., Ferrara L.A., Lieberman I.H. Distribution of anterior cortical shear strain after a thoracic wedge compression fracture. Spine J. 2004;4:76-87.
63. Bell G.H., Dunbar O., Beck J.S., et al. Variations in strength of vertebrae with age and their relation to osteoporosis. Calcif Tissue Res. 1967;1:75-86.
64. Perry O. Fracture of the vertebral end-plate in the lumbar spine. Acta Orthop Scand. 1957;25:34-39.
65. Park J.B. Structure-property relationships of biological materials. In: Park J.B., editor. Biomaterials science and engineering. New York: Plenum, 1984. chapter 6
66. Carter D.R., Hayes W.C. The compressive behavior of bone as a two-phase porous structure. J Bone Joint Surg [Am]. 1977;59:954-962.
67. Tencer A.F., Johnson K.D. Factors affecting the strength of bone. In: Tencer A.F., Johnson K.D., editors. Biomechanics in orthopedic trauma: bone fracture and fixation. Philadelphia: JB Lippincott, 1994. chapter 2
68. Rockoff S.D., Sweet E., Bleustein J. The relative contribution of trabecular and cortical bone to the strength of human lumbar vertebrae. Calcif Tissue Res. 1969;3:163-175.
69. Lindsay R., Silverman S.L., Cooper C., et al. Risk of new vertebral fracture in the year following a fracture. JAMA. 2001;285:320-323.
70. Haher T.R., Felmy W., Baruch H., et al. The contribution of the three columns of the spine to rotational stability. A biomechanical model. Spine (Phila Pa 1976). 1989;14:663-669.
71. Haher T.R., Tozzi J.M., Lospinuso M.F., et al. The contribution of the three columns of the spine to spinal stability: a biomechanical model. Paraplegia. 1989;27:432-439.
72. Haher T.R., Bergman M., O’Brien M., et al. The effect of the three columns of the spine on the instantaneous axis of rotation in flexion and extension. Spine (Phila Pa 1976). 1991;16:S312-S318.
73. Benzel E.C. Biomechanics of spine stabilization. Rolling Meadows, Illinois: American Association of Neurological Surgeons; 2001. pp 1–305
74. Haher T.R., O’Brien M., Felmly W.T., et al. Instantaneous axis of rotation as a function of the three columns of the spine. Spine (Phila Pa 1976). 1992;17:S149-S154.
75. Kayanja M.M., Togawa D., Lieberman I.H. Biomechanical changes following the augmentation of experimental osteoporotic vertebral compression fracture in the cadaveric thoracic spine. Spine J. 2005;5:55-63.
76. Lieberman I.H., Togawa D., Kayanja M.M. Vertebroplasty and kyphoplasty—filler materials. Spine J. 2005;5:S305-S316.
77. Jasper L.E., Deramond H., Mathis J.M., et al. Material properties of various cements for use with vertebroplasty. J Mater Sci Mater Med. 2002;13:1-5.
78. FDA Public Health Web Notification. Complications related to the use of bone cement and bone void fillers in treating compression fractures of the spine. http://www.fda.gov/cdrh/safety/bonecement.pdf.
79. Gangi A., Kastler B.A., Dietemann J.L. Percutaneous vertebroplasty guided by a combination of CT and fluoroscopy. AJNR Am J Neuroradiol. 1994;15:83-86.
80. Togawa D., Bauer T.W., Lieberman I.H., Takikawa S. Histologic evaluation of human vertebral bodies after vertebral augmentation with polymethyl methacrylate. Spine (Phila Pa 1976). 2003;28:1521-1527.
81. Togawa D., Kovacic J.J., Bauer T.W., et al. Radiographic and histologic findings of vertebral augmentation using polymethylmethacrylate in the primate spine: percutaneous vertebroplasty versus kyphoplasty. Spine (Phila Pa 1976). 2006;31:E4-E10.
82. Verlaan J.J., Oner F.C., Slootweg P.J., et al. Histologic changes after vertebroplasty. J Bone Joint Surg [Am]. 2004;86:1230-1238.
83. Fulmer M.T., Ison I.C., Hankermayer C.R., et al. Measurements of the solubilities and dissolution rates of several hydroxyapatites. Biomaterials. 2002;23:751-755.
84. Friedman C.D., Costantino P.D., Takagi S., Chow L.C. BoneSource hydroxyapatite cement: a novel biomaterial for craniofacial skeletal tissue engineering and reconstruction. J Biomed Mater Res. 1998;43:428-432.
85. Chow L.C., Hirayama S., Takagi S., Parry E. Diametral tensile strength and compressive strength of a calcium phosphate cement: effect of applied pressure. J Biomed Mater Res. 2000;53:511-517.
86. Lee D.D., Tofighi A., Aiolova M., Chakravarthy P., et al. Alpha-BSM: a biomimetic bone substitute and drug delivery vehicle. Clin Orthop Relat Res. 1999;367(suppl)::S396-S405.
87. Seeherman H.J., Bouxsein M., Kim H., et al. Recombinant human bone morphogenetic protein-2 delivered in an injectable calcium phosphate paste accelerates osteotomy-site healing in a nonhuman primate model. J Bone Joint Surg [Am]. 2004;86:1961-1972.
88. Constantz B.R., Ison I.C., Fulmer M.T., et al. Skeletal repair by in situ formation of the mineral phase of bone. Science. 1995;267:1796-1799.
89. Larsson S., Bauer T.W. Use of injectable calcium phosphate cement for fracture fixation: a review. Clin Orthop Relat Res. 2002;395:23-32.
90. Frankenburg E.P., Goldstein S.A., Bauer T.W., et al. Biomechanical and histological evaluation of a calcium phosphate cement. J Bone Joint Surg [Am]. 1998;80:1112-1124.
91. Goodman S.B., Bauer T.W., Carter D., et al. Norian SRS cement augmentation in hip fracture treatment. Laboratory and initial clinical results. Clin Orthop Relat Res. 1998;348:42-50.
92. Larsson S., Mattsson P., Bauer T.W. Resorbable bone cement for augmentation of internally fixed hip fractures. Ann Chir Gynaecol. 1999;88:205-213.
93. Schildhauer T.A., Bauer T.W., Josten C., Muhr G. Open reduction and augmentation of internal fixation with an injectable skeletal cement for the treatment of complex calcaneal fractures. J Orthop Trauma. 2000;14:309-317.
94. Verlaan J.J., van Helden W.H., Oner F.C., et al. Balloon vertebroplasty with calcium phosphate cement augmentation for direct restoration of traumatic thoracolumbar vertebral fractures. Spine (Phila Pa 1976). 2002;27:543-548.
95. Turner T.M., Urban R.M., Lim T.H., et al. Vertebroplasty using injectable calcium phosphate cement compared to polymethylmethacrylate in a unique canine vertebral body large defect model. New Orleans, LA: 49th Annual Meeting of Orthopaedic Research Society; 2003. Paper 267
96. Takikawa S., Bauer T.W., Turner A.S., et al. Comparison of injectable calcium phosphate cement and polymethylmethacrylate for use in vertebroplasty: in-vivo evaluation using an osteopenic sheep model. Tampa, FL: 28th Annual Meeting of the Society of Biomaterials; 2002. 23 [abstract]
97. Liebschner M.A., Rosenberg W.S., Keaveny T.M. Effects of bone cement volume and distribution on vertebral stiffness after vertebroplasty. Spine (Phila Pa 1976). 2001;26:1547-1554.
98. Lim T.H., Brebach G.T., Renner S.M., et al. Biomechanical evaluation of an injectable calcium phosphate cement for vertebroplasty. Spine (Phila Pa 1976). 2002;27:1297-1302.
99. Belkoff S.M., Mathis J.M., Erbe E.M., Fenton D.C. Biomechanical evaluation of a new bone cement for use in vertebroplasty. Spine (Phila Pa 1976). 2000;25:1061-1064.
100. Heini P.F., Berlemann U., Kaufmann M., et al. Augmentation of mechanical properties in osteoporotic vertebral bones—a biomechanical investigation of vertebroplasty efficacy with different bone cements. Eur Spine J. 2001;10:164-171.
101. Belkoff S.M., Mathis J.M., Deramond H., et al. An ex vivo biomechanical evaluation of a hydroxyapatite cement for use with kyphoplasty. AJNR Am J Neuroradiol. 2001;22:1212-1216.
102. Turner T.M., Urban R.M., Gitelis S., et al. Resorption evaluation of a large bolus of calcium sulfate in a canine medullary defect. Orthopedics. 2003;26(Suppl 5):577-579.
103. Togawa D., Reid J., Bauer T.W., et al. Histological evaluation of calcium sulfate HA/TCP composites using a canine metaphyseal defect model. San Francisco, CA: 50th Annual Meeting of Orthopaedic Research Society; 2004. Poster 1040
104. Rohmiller M.T., Schwalm D., Glattes R.C., et al. Evaluation of calcium sulfate paste for augmentation of lumbar pedicle screw pullout strength. Spine J. 2002;2:255-260.
105. Glazer P.A., Spencer U.M., Alkalay R.N., Schwardt J. In vivo evaluation of calcium sulfate as a bone graft substitute for lumbar spinal fusion. Spine J. 2001;1:395-401.
106. Stubbs D., Deakin M., Chapman-Sheath P., et al. In vivo evaluation of resorbable bone graft substitutes in a rabbit tibial defect model. Biomaterials. 2004;25:5037-5044.
107. Walsh W.R., Morberg P., Yu Y., et al. Response of a calcium sulfate bone graft substitute in a confined cancellous defect. Clin Orthop Relat Res. 2003;406:228-236.
108. DiCicco M., Compton R., Jansen-Varnum S.A. In vitro evaluation of orthopedic composite cytotoxicity: assessing the potential for postsurgical production of hydroxyl radicals. J Biomed Mater Res. 2004;72:146-155.
109. Erbe E.M., Clineff T.D., Gualtieri G. Comparison of a new bisphenol-a-glycidyl dimethacrylate-based cortical bone void filler with polymethyl methacrylate. Eur Spine J. 2001;10(Suppl 2):S147-S152.
110. Deramond H., Palussiere J. Percutaneous vertebroplasty with a new biocompatible composite. Helsinki, Finland: Annual Meeting, Proceedings of European Federation of National Associations of Orthopaedics and Traumatology (EFORT); 2003.
111. Clineff T., Erbe E., Gualtieri G., Carroll B. Quantitative evaluation of bone apposition to Cortoss at 1 year. San Francisco, CA: 47th Annual Meeting of the Orthopaedic Research Society; 2001.
112. Togawa D., Lieberman I.H., Bauer T.W., et al. Histological evaluation of biopsies obtained from vertebral compression fractures: unsuspected myeloma and osteomalacia. Spine (Phila Pa 1976). 2005;30:781-786.
113. Keller T.S., Harrison D.E., Colloca C.J., et al. Prediction of osteoporotic spinal deformity. Spine (Phila Pa 1976). 2003;28:455-462.
114. Hasserius R., Karlsson M.K., Nilsson B.E., et al. Prevalent vertebral deformities predict increased mortality and increased fracture rate in both men and women: a 10-year population-based study of 598 individuals from the Swedish cohort in the European Vertebral Osteoporosis Study. Osteoporos Int. 2003;14:61-68.
115. Silverman S.L. The clinical consequences of vertebral compression fracture. Bone. 1992;13(Suppl 2):S27-S31.
116. Uppin A.A., Hirsch J.A., Centenera L.V., et al. Occurrence of new vertebral body fracture after percutaneous vertebroplasty in patients with osteoporosis. Radiology. 2003;226:119-124.
117. Harrop J.S., Prpa B., Reinhardt M.K., Lieberman I. Primary and secondary osteoporosis’ incidence of subsequent vertebral compression fractures after kyphoplasty. Spine (Phila Pa 1976). 2004;29:2120-2125.
118. Fribourg D., Tang C., Sra P., et al. Incidence of subsequent vertebral fracture after kyphoplasty. Spine (Phila Pa 1976). 2004;29:2270-2276. discussion 2277
119. Sandaresan N., Krol G., Digiacinto G., Hughes J. Metastatic tumors of the spine. In: Sundaresan N., Schmidek H.H., Schiller A.L., Rosenthal D., editors. Tumors of the spine: diagnosis and clinical management. Philadelphia: WB Saunders; 1990:279-304.
120. McLain R.F., Weinstein J.N. Tumors of the spine. In Herkowitz H.N., Garfin S.R., Balderston R.A., et al, editors: The spine, ed 4, Philadelphia: WB Saunders, 1999. Chapter 38, pp 1171–1206
121. Leech J.A., Dulberg C., Kellie S., et al. Relationship of lung function to severity of osteoporosis in women. Am Rev Respir Dis. 1990;141:68-71.
122. Kado D.M., Browner W.S., Palermo L., et alfor the Study of Osteoporotic Fractures Research Group. Vertebral fractures and mortality in older women: a prospective study. Arch Intern Med. 1999;159:1215-1220.
123. Lecouvet F.E., Vande Berg B.C., Maldague B.E., et al. Vertebral compression fractures in multiple myeloma. Part I. Distribution and appearance at MR imaging. Radiology. 1997;204:195-199.
124. Lane J.M., Hong R., Koob J., et al. Kyphoplasty enhances function and structural alignment in multiple myeloma. Clin Orthop Relat Res. 2004;426:49-53.
125. Khanna A.J., Neubauer P., Togawa D., et al. Kyphoplasty and vertebroplasty for the treatment of spinal metastases. Support Cancer Ther. 2005;3:21-25.