Chapter 10 Valvular Heart Disease
Severe valvular heart disease imposes a volume or pressure load on the heart that if untreated can result in ventricular impairment and heart failure. Surgical correction prevents further ventricular dysfunction and improves the rate of survival. However, surgery is associated with appreciable morbidity and mortality rates. Therefore, appropriate timing of surgery is essential; excessive delay can lead to irreversible ventricular dysfunction, but if intervention is too early, the risks involved in surgery may outweigh the benefits.
In this chapter the causes, pathophysiology, treatments, and postoperative problems of valvular heart disease are reviewed. Many of the issues relating to comorbid conditions outlined in Chapter 9 apply equally to patients undergoing valve surgery.
SURGICAL TREATMENT OF VALVULAR HEART DISEASE
Valves and Procedures
Prosthetic valves may be made of mechanical or bioprosthetic material. Mechanical valves are durable but require lifelong anticoagulation with warfarin. Bioprosthetic valves, at least in the aortic position, do not usually require lifelong warfarin, but are less durable and have a primary failure rate of about 30% at 10 to 15 years.1–3 Bioprosthetic valves are less durable in the mitral position than in the aortic position. In general, mechanical valves are preferred in younger patients, and bioprosthetic valves are preferred in older patients and in patients who may become pregnant. Smaller valves tend to be used in the aortic position (commonly 21 to 25 mm) and larger valves tend to be used in the mitral position (commonly 27 to 31 mm). Mechanical prostheses in each of the four heart valve positions on the frontal and lateral chest radiographs are shown in Fig. 6-6.
Mechanical Valves
Most mechanical valves that are currently implanted are of the bileaflet type (e.g., St. Jude Medical, CarboMedics; Fig, 10-1A). Bileaflet valves have a low profile, provide relatively unobstructed flow, and have minimal areas of stagnation on the downstream side of the disks. Bileaflet valves have a characteristic appearance on echocardiography (Fig. 10-2).
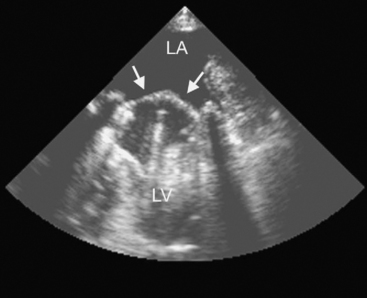
(Reproduced, with permission, from Sidebotham D, Merry A, Legget M: Practical Perioperative Transoesophageal Echocardiography. Fig. 12.2, p. 187. Philadelphia, Butterworth Heinemann, 2003.)
Tilting-disk valves (e.g., Medtronic-Hall; see Fig. 10-1B; Fig. 10-3) have a single pivoting circular disk. Compared to bileaflet valves, tilting-disk valves cause greater impedance to forward flow and a larger area of stagnation on the downstream surface of the disk. The Medtronic-Hall valve has a characteristic central regurgitant jet (see Fig. 10-3).
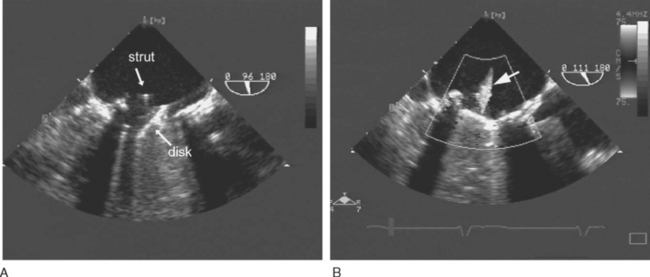
(Reproduced, with permission, from Sidebotham D, Merry A, Legget M: Practical Perioperative Transoesophageal Echocardiography. Fig. 12.4, p. 189. Philadelphia, Butterworth Heinemann, 2003.) TEE, transesophageal echocardiography.
The “ball-in-cage” valve, typified by the Starr-Edwards valve (see Fig. 10-1 C), is the oldest type of mechanical valve. The efficacy of the Starr-Edwards valve is well established; hundreds of thousands of implantations have been performed over 40 years. However, the valve is bulky, results in moderate valvular obstruction, may cause hemolysis, and carries a slightly higher risk for thromboembolism than other valve types. All three types of valves may be used in the mitral or aortic positions.
Bioprosthetic Valves
Bioprosthetic valves may be stented or unstented. Stented valves are made from porcine aortic valves (e.g., Mosaic) or from bovine pericardial tissue (e.g., Carpentier-Edwards Perimount; see Fig. 10-1 D). The stents facilitate implantation and help maintain the three-dimensional structure of the valve. Stented bioprosthetic valves may be used in the aortic or mitral position.
Stentless bioprosthetic valves are made from porcine aortic roots (e.g., Medtronic Freestyle; see Fig. 10-1 E) or cryopreserved human cadaveric aortic roots (homografts). Compared to stented valves, stentless valves provide less obstruction to flow, a reduced risk for endocarditis, and increased durability, but their implantation is more technically demanding, and they can be used only in the aortic and pulmonary positions. Stentless valves may be inserted using a modified subcoronary method as for a standard aortic valve replacement. In addition, some stentless valves, such as the homograft and Freestyle, may be implanted as a freestanding aortic root, in which the aortic valve and sinuses of Valsalva are replaced by the graft, and the coronary arteries are reimplanted as buttons onto fenestrations in the bioprosthesis. An aortic root replacement may be preferred to a subcoronary stentless aortic valve insertion because it can be easier to perform and it provides a larger aortic diameter.
An alternative to a stentless aortic root replacement is the Ross procedure, in which the patient’s own pulmonary valve is transplanted into the aortic position (autograft), and a pulmonary homograft is placed in the pulmonary position. Usually a patient undergoing a Ross procedure (particularly if for aortic incompetence) also requires an aortic root annuloplasty to reduce the annular diameter to between 26 and 28 mm. The pulmonary autograft is durable and may grow with the patient.4 Thus, the Ross procedure is popular for use in younger patients. However, the operation is a long, technically demanding procedure, and it has a mortality rate about twice that of a standard valve replacement. In the long term, autograft dilatation and homograft stenosis can occur.5
SPECIFIC VALVULAR PATHOLOGY
Aortic Stenosis
The causes of aortic valve stenosis are listed in Table 10-1; of these, the most common is calcific degeneration. Calcific degeneration can affect a normal trileaflet valve (Fig. 10-4), a congenitally bicuspid valve, or a bioprosthetic valve. Calcific degeneration of a trileaflet valve typically occurs in patients more than 70 years of age, whereas degeneration of a bileaflet valve typically presents in young adulthood or middle age. Stenosis may also occur at the subvalvular level, either as dynamic left ventricular outflow tract (LVOT) obstruction or secondary to a subaortic membrane.
Causes | Lesion |
---|---|
Calcific degeneration | Predominantly stenosis |
Degeneration of a bicuspid valve | Stenosis, regurgitation, or mixed |
Degeneration of a bioprosthetic valve | Stenosis, regurgitation, or mixed |
Rheumatic aortic valve disease | Stenosis, regurgitation, or mixed |
Thrombus or pannus formation on a mechanical valve | Stenosis |
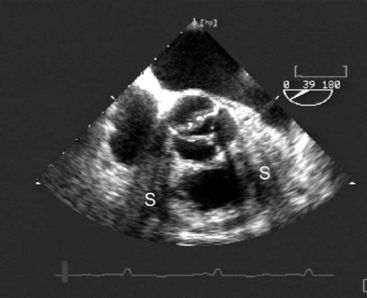
(Reproduced, with permission, from Sidebotham D, Merry A, Legget M: Practical Perioperative Transoesophageal Echocardiography. Fig. 10.3, p. 158. Philadelphia, Butterworth Heinemann, 2003.) TEE, transesophageal echocardiography.
Pathophysiology
Aortic stenosis imposes a pressure load on the left ventricle that results in increased intraventricular pressure and systolic wall tension (Fig. 1-7). Over time, this pressure overload leads to concentric left ventricular hypertrophy, increased end-diastolic pressure and, not infrequently, clinically important diastolic dysfunction. Systolic function is usually preserved until late in the disease process.
Patients with aortic stenosis are at high risk for myocardial ischemia. Myocardial oxygen demands are increased due to the effects of increased pressure work, muscle mass, and wall tension, but coronary blood flow, particularly to the subendocardium, is reduced as a consequence of increased end-diastolic pressure. Also, more than 50% of patients with aortic stenosis have significant coronary artery disease.6 Patients with severe aortic stenosis have a relatively fixed stroke volume and may develop profound hypotension (and myocardial ischemia) in response to modest vasodilation or exercise.
Clinical Features and Investigations
The ECG may demonstrate left ventricular hypertrophy and strain. Nonspecific ST segment changes occur in the majority of patients. Rarely, calcific aortic stenosis is associated with atrioventricular (AV) block. The chest radiograph is frequently normal, although calcification of the valve may be evident. Echocardiography is mandatory to confirm the diagnosis and to assess severity (Table 10-2). Also, it is essential to confirm that the stenosis is due to valvular obstruction and not subvalvular pathology. Significant valvular stenosis is unlikely in the absence of heavy calcification of the valve. Coronary angiography is required in patients over the age of 40 and in those with significant risk factors for coronary artery disease.
Medical Treatment
Symptomatic aortic stenosis is a surgical condition, and medical therapy should not be used to delay surgery. β Blockers provide some relief from angina and may protect against tachyarrhythmias.
Acute Decompensation
Patients with aortic stenosis may present to the intensive care unit with myocardial ischemia, arrhythmias, or congestive cardiac failure. Initial treatment of myocardial ischemia includes oxygen, aspirin, analgesia and, in the absence of heart failure, β blockers. An intraaortic balloon pump (IABP) may be helpful to augment coronary perfusion pressure. In patients with acute coronary syndromes, urgent angiography and combined surgical revascularization and aortic valve replacement should be considered. Pulmonary edema should be treated with diuretics and respiratory support as appropriate. Tachyarrhythmias such as atrial fibrillation are poorly tolerated and must be treated promptly (see Chapter 21). Induction of general anesthesia (e.g., for cardioversion) can cause profound hypotension.
Despite the conventional wisdom that vasodilators are contraindicated in aortic stenosis, low-dose nitroprusside (in slow increments, up to 150 μg/min) has recently been shown to increase cardiac output in normotensive patients with congestive cardiac failure and severe aortic stenosis.7 The authors of this study argue that systemic vasoconstriction may contribute to congestive cardiac failure in patients with severe aortic stenosis. Although this study has received a lot of attention, it must be stressed that potent vasodilators can precipitate cardiovascular collapse in patients with severe aortic stenosis, and that this treatment is not routine.
Surgical Treatment
Sudden death as a result of aortic stenosis is rare in asymptomatic patients. Thus, the presence of symptoms is the primary indication for surgery, irrespective of the aortic valve area or gradient. Recently, levels of B-type natriuretic peptide (BNP; see Chapter 19) have been shown to reflect the onset of symptoms in aortic stenosis. In two studies, patients with symptomatic aortic stenosis had median (± interquartile range) levels of N-BNP (the aminoterminal portion of BNP): 112 pmol/l (70 to 193) and 131 pmol/l (50 to 202) compared to 33 pmol/l (16 to 58) and 31 pmol/l (19 to 56) in asymptomatic patients.8,9 Thus, levels of these hormones may prove to be a useful complement to symptom onset in determining the timing of surgery.
Valve replacement is virtually always required in aortic stenosis because calcified, immobile valve leaflets cannot be repaired. Aortic valve surgery is usually performed through a midline sternotomy. Minimally invasive incisions involving an upper sternotomy and a J incision into the third or fourth intercostal space are also used. The technique of cardiopulmonary bypass (CPB) is similar to that described in Chapter 9. Myocardial protection may be difficult in the setting of severe left ventricular hypertrophy, particularly if there is concomitant aortic regurgitation (see later discussion). Damage to the His bundle may occur during placement of sutures and may cause complete heart block. After CPB, myocardial ischemia may arise as a consequence of (1) a coronary embolus involving aortic or valvular debris; (2) the incorrect seating of a prosthetic valve, causing coronary ostial obstruction; (3) distortion of a coronary artery during reimplantation when performing aortic root replacement. Heavy calcification of the valve or proximal aorta also predisposes to systemic emboli. Percutaneous balloon valvuloplasty of the aortic valve is associated with a very high procedural complication rate; it confers minimal long-term benefit and is rarely performed.10
Postoperative Issues
The operative mortality rate in isolated, first-time aortic valve replacement is 3% to 4%,11,12 but it increases substantially if additional cardiac surgery is required and if left ventricular function is impaired.11 Unlike other valve lesions, the hemodynamic state is immediately improved by valve replacement, even in the presence of preexisting left ventricular dysfunction.
Occasionally, patients with marked left ventricular hypertrophy (particularly involving the basal anterior septum) develop dynamic left ventricular outflow tract (LVOT) obstruction and systolic anterior motion (SAM) of the anterior mitral valve leaflet after aortic valve replacement. Severe SAM can cause hypotension, low cardiac output, and mitral regurgitation. The treatment of this condition is described in Chapter 20.
Aortic Regurgitation
Chronic aortic regurgitation results from aortic root dilatation (Fig. 10-5) or abnormalities of the aortic valve leaflets (Table 10-3). Acute aortic regurgitation results from dissection (see Fig. 11-7), endocarditis and, occasionally, trauma.
Valvular Dysfunction |
Rheumatic aortic disease |
Degeneration of a bicuspid valve |
Degeneration of a bioprosthetic valve |
Endocarditis on a native or prosthetic (bioprosthetic or mechanical) valve |
Leaflet prolapse due to trauma or myxomatous disease |
Aortic Disease |
Aortic root dilation |
Aortic dissection |
Pathophysiology
Because the regurgitant volume is returned to the left ventricle during each diastolic period, aortic regurgitation imposes a volume load on the left ventricle, which results in progressive left ventricular dilatation and eccentric left ventricular hypertrophy. Left ventricular compliance is increased, allowing large ventricular volumes to be accommodated with minimal increase in end-diastolic pressure (see Fig. 1-7). As the left ventricular diameter increases, wall tension and hence afterload are increased. There is compensatory systemic vasodilation. Arterial end-diastolic pressure is low because of diastolic run off into the left ventricle. Thus, the aortic valve opens at a low pressure but peak systolic aortic pressure is increased due to the high stroke volume. Arterial pulse pressure is increased. There may be baroreceptor-mediated tachycardia.
Clinical Features and Investigations
Symptoms occur late in chronic severe aortic regurgitation, by which time important left ventricular dysfunction may have developed. Symptoms, when they do occur, are of congestive cardiac failure: exertional dyspnea and orthopnea. Physical findings include a collapsing pulse, a wide pulse pressure, and diastolic hypotension. The apex beat is displaced laterally and is hyperdynamic. The aortic component of the second heart sound may be soft and there is often a third heart sound. The murmur of aortic regurgitation occurs in early diastole, is high in pitch, and is best heard at the left sternal edge with the patient sitting up and holding his or her breath at expiration.
Surgical Treatment
To prevent irreversible ventricular damage, surgery for chronic severe aortic regurgitation is indicated in patients who are symptomatic or who have evidence of ventricular dysfunction or dilatation (ejection fraction <50% or end-systolic dimension >5.5 cm).13 Severely depressed left ventricular dysfunction (e.g., ejection fraction <20%) imposes a very high perioperative risk and may be considered a contraindication to surgery.
Postoperative Problems
Left ventricular systolic dysfunction is common postoperatively and is treated with optimization of preload, maintenance of a high normal heart rate (e.g., with pacing at 90/min), and inotropic support (see Chapter 21). Echocardiography and a pulmonary artery catheter are helpful to guide therapy. Severe pulmonary hypertension and right ventricular dysfunction are uncommon. The issues of systemic hypertension and damage to the His bundle are the same as those following surgery for aortic stenosis. Following complex aortic root replacement procedures, myocardial stunning, coagulopathy, bleeding, and a marked systemic inflammatory response syndrome are not uncommon.
Mitral Regurgitation
Mitral regurgitation is often thought of as a benign lesion because patients can remain stable for many years. However, longstanding, severe mitral regurgitation can cause irreversible left ventricular dysfunction, atrial fibrillation, pulmonary hypertension, and right ventricular failure.
The causes of mitral regurgitation are listed in Table 10-4. In the developed world, myxomatous degeneration is the most common cause of severe mitral regurgitation. Myxomatous degeneration is a connective tissue disorder characterized by thickening and elongation of the mitral leaflets and chordae and by dilatation of the mitral annulus (Fig. 10-6). Regurgitation usually develops slowly due to leaflet prolapse, but a sudden deterioration may indicate a flail leaflet (see Fig. 7-10) caused by rupture of a chorda.
Cause | Lesion | Mechanism of Regurgitation |
---|---|---|
Myxomatous degeneration | Regurgitation | Leaflet prolapse (chordal elongation) |
Leaflet flail (chordal rupture) | ||
Annular dilation | ||
Ventricular dysfunction | Regurgitation | Leaflet restriction due to ventricular dilation |
Annular dilation | ||
Papillary muscle dysfunction | ||
Papillary muscle rupture | ||
Endocarditis of a native or prosthetic valve | Regurgitation | Leaflet perforation and destruction with transvalvular regurgitation |
Prosthetic valve dehiscence with paravalvular regurgitation | ||
Degeneration of a bioprosthetic valve | Regurgitation, stenosis, or mixed | Transvalvular regurgitation |
Dehiscence of a mitral valve repair | Regurgitation | |
Rheumatic mitral valve disease | Stenosis, mixed, or regurgitation | Leaflet restriction and chordal shortening |
Left ventricular dysfunction, usually secondary to coronary artery disease, is an important cause of mitral regurgitation. Some degree of mitral regurgitation develops in more than 50% of patients after a myocardial infarction.14 In many cases this regurgitation is mild and resolves spontaneously with the resolution of myocardial stunning. However, in some patients, as the ventricle remodels, mitral regurgitation becomes chronic and severe. As the left ventricle becomes dilatated, there is loss of the normal cylindrical shape and development of a spherical conformation. This results in tethering of the papillary muscles and a central coaptation defect of the mitral leaflets. Asymmetric ventricular dilatation, usually involving the posterior wall, causes regurgitation by means of a similar mechanism. Acute severe mitral regurgitation may arise following myocardial infarction due to papillary muscle rupture (see Chapter 9).
Pathophysiology
As with aortic regurgitation, chronic mitral regurgitation results in left ventricular volume overload with ventricular dilatation and eccentric hypertrophy. Unlike aortic regurgitation, left ventricular afterload is reduced with mitral regurgitation due to systolic ejection into the low-impedance left atrium. (Although this is partially offset by left ventricular dilatation, which increases wall stress and therefore afterload). The combination of high preload and low afterload preserves ejection fraction despite potentially important systolic dysfunction. Over time, chronic severe mitral regurgitation causes left atrial dilatation, pulmonary hypertension, and right ventricular dysfunction. Atrial fibrillation is common once the left atrial diameter exceeds 4.5 cm.15 Atrial fibrillation is generally better tolerated in the context of mitral regurgitation than in cases of aortic or mitral stenosis.
Acute mitral regurgitation results in sudden volume overloading of the left ventricle. Left ventricular dilatation has not had time to develop, which causes abrupt rises in left ventricular end-diastolic and left atrial pressures. Pulmonary edema, pulmonary hypertension, acute right ventricular dysfunction, and shock can develop rapidly.
Indications for Surgery
Surgery is indicated for patients with severe mitral regurgitation who are symptomatic or who are asymptomatic and have evidence of mild to moderate left ventricular dysfunction (ejection fraction 30-60%, left ventricular end-systolic dimension >4.0 cm).13 Thus, even mild ventricular dysfunction is an indication for surgery because the low afterload state masks significant ventricular impairment. Surgery may also be offered to symptomatic patients with severe (ejection fraction <30%) left ventricular dysfunction, particularly if valve repair is thought to be feasible.
Surgical Treatment
The mortality rates seen in isolated mitral valve replacement are higher than those in aortic valve replacement; they are about 6%.11 Mortality rates increase to above 10% when multiple valves are replaced or when left ventricular function is severely impaired; it is approximately 15% when mitral valve replacement is combined with coronary revascularization.11 Mitral valve repair is associated with lower mortality rates than is valve replacement,16,17 mainly because of improved ventricular function and reduced risk for thromboembolism. Certain valve pathologies, notably myxomatous degeneration that is limited to the posterior leaflet and ischemic mitral regurgitation, have a high chance of successful repair. Valve replacement is usually required for rheumatic heart disease, endocarditis, or myxomatous degeneration with extensive anterior or bileaflet involvement.
If valve replacement is required, postoperative ventricular function is better maintained if the subvalvular apparatus (chordae and papillary muscles) is preserved at the time of surgery.18 Bioprosthetic valves deteriorate relatively rapidly in the mitral position, and their use tends to be limited to individuals who are unsuitable for warfarin, such as those who wish to become pregnant. Patients with pulmonary hypertension or rheumatic heart disease may have coexisting tricuspid valve disease, necessitating concomitant repair (see later material). Patients with atrial fibrillation may be suitable for a maze type-procedure (see later material).
Postoperative Problems
New or recurrent atrial fibrillation is common after mitral valve surgery. Large V waves suggestive of mitral regurgitation are occasionally seen on the pulmonary artery wedge trace despite a normally functioning prosthesis, particularly when the left atrium is small. Right ventricular dysfunction may occur as a consequence of preexisting pulmonary hypertension and the acute increases in pulmonary vascular resistance associated with CPB. The implications and treatment of pulmonary hypertension are outlined in Chapter 24.
After mitral valve repair, problems involving dehiscence of the repair, mitral stenosis, or SAM (see also Aortic Stenosis, in earlier material) can develop. Dehiscence of the repair causes severe mitral regurgitation. SAM involves prolapse of the anterior mitral leaflet into the LVOT during systole, causing outflow tract obstruction and mitral regurgitation. The echocardiographic appearances are characteristic (see Fig. 7-12). Risk factors for SAM in this situation include a small nondilated left ventricle, the use of an undersized annuloplasty ring, and excessive posterior leaflet tissue causing anterior displacement (i.e., toward the LVOT) of the mitral coaptation line. The treatment of this condition is described in Chapter 20. Mitral stenosis is uncommon after mitral valve repair, but it can occur. The diagnosis is usually confirmed by the intraoperative TEE examination. For the reasons outlined, it is essential that any patient who becomes hemodynamically unstable or develops pulmonary edema following mitral valve surgery should undergo urgent TEE examination.
Mitral Stenosis
The most common cause of native mitral stenosis is rheumatic heart disease.
Rheumatic mitral valve disease results in fibrosis, fusion, and calcification of the valve apparatus. The commissures and leaflet tips are commonly involved, but the body of the leaflets, the annulus, and the chordae can also be involved. The echocardiographic appearances are characteristic (Fig. 10-7). Pure stenosis or mixed stenosis and regurgitation are the typical lesions. Mitral stenosis can also result from calcific degeneration of a bioprosthetic valve. Thrombus or pannus (fibrous overgrowth) formation on a mechanical valve is a rare cause of mitral stenosis.
Pathophysiology
Mitral stenosis causes obstruction of left ventricular filling. The left atrium becomes pressure- and volume-loaded, and the left ventricle is underfilled. Increased left atrial pressure results in pulmonary congestion and left atrial dilatation. Once the left atrial dimension exceeds 4.5 cm, atrial fibrillation is likely. Pulmonary venous hypertension initially results in passive pulmonary arterial hypertension, but over time, changes within the pulmonary arterioles lead to reactive arterial pulmonary hypertension (see Chapter 24). Severe pulmonary arterial hypertension can cause pressure and volume overload of the right ventricle, which results in functional tricuspid regurgitation and, eventually, right ventricular failure. Shunts from the pulmonary vein to the bronchial veins may develop, and they can rupture, causing hemoptysis. The combination of mitral stenosis and atrial fibrillation is a potent stimulus for the formation of left atrial thrombus, which can embolize systemically.
Clinical Features and Investigations
The ECG may show atrial fibrillation or, if the patient is in sinus rhythm, P-mitrale. Right ventricular hypertrophy may be present and is a marker of severity. The chest radiograph may demonstrate characteristic features of left atrial enlargement (see Fig. 6-3). In severe mitral stenosis, there may be evidence of enlargement of the pulmonary artery, the right ventricle, and the right atrium. Radiographic signs of pulmonary venous hypertension and pulmonary edema may be present.
Echocardiography is essential for determining the severity of the mitral stenosis (Table 10-5). Additionally, the presence of other valve pathology (particularly mitral and tricuspid regurgitation), left atrial thrombus, right ventricular dysfunction, and pulmonary hypertension can be evaluated. TEE may be required to rule out left atrial thrombus. In the presence of overt right ventricular failure, a right heart catheter study should be considered to assess pulmonary artery pressures and resistance. Coronary angiography should be performed in patients above 40 years of age and those with risk factors for coronary artery disease.
Table 10-5 Severity of Mitral Stenosis Based on Valve Area and Pressure Gradients
Mean Transvalvular Pressure Gradient | Mitral Valve Area | |
---|---|---|
Normal | 4-6 cm2 | |
Mild | <6 mmHg | >1.5 cm2 |
Moderate | 6-12 mmHg | 1.0-1.5 cm2 |
Severe | >12 mmHg | <1.0 cm2 |
Acute Decompensation
Acute decompensation is usually associated with a precipitating event such as pregnancy, sepsis, or the onset of atrial fibrillation. Patients present with acute pulmonary edema. The initial treatment includes diuretics, respiratory support, and control of atrial fibrillation. Hypotension should be treated with inotropic agents and an IABP. Norepinephrine may increase left atrial pressure and potent β-adrenergic agents may worsen tachycardia. Acute right ventricular failure may respond to inhaled pulmonary vasodilators such as nitric oxide (see Chapter 24). Urgent intervention, with either percutaneous valvuloplasty or surgery, is indicated.
Percutaneous Valvuloplasty and Surgery
Most cases of rheumatic mitral stenosis can be treated successfully with percutaneous mitral balloon valvuloplasty. In this procedure, a balloon catheter is passed via the femoral vein through the interatrial septum and inflated across the mitral valve. Contraindications to the procedure include moderate or severe mitral regurgitation, left atrial thrombus, and heavy valvular calcification. The procedure carries low risk and the outcome is generally good. The requirement for subsequent intervention—usually surgery—is approximately 10% at 3 years and 40% at 7 years.10,19 Percutaneous valvuloplasty is particularly useful in patients who become symptomatic during pregnancy.
Tricuspid Valve Surgery
Tricuspid regurgitation most commonly arises in the context of normal tricuspid valvular morphology (functional tricuspid regurgitation) secondary to right ventricular dilatation. Less commonly, tricuspid regurgitation arises from structural tricuspid valve disease (Table 10-6). Tricuspid stenosis is uncommon and is almost always related to rheumatic or carcinoid disease.
Functional Tricuspid Regurgitation |
Right ventricular volume overload (e.g., atrial septal defect) |
Right ventricular pressure overload (e.g., mitral valve disease) |
Right ventricular systolic dysfunction (e.g., stunning) |
Structural Tricuspid Valve Disease |
Endocarditis (regurgitation) |
Rheumatic (stenosis, regurgitation, or mixed disease) |
Carcinoid disease (stenosis, regurgitation, or mixed disease) |
Ebstein anomaly (regurgitation) |
Trauma (regurgitation) |
Pathophysiology and Clinical Features
Structural tricuspid regurgitation causes right ventricular volume overload. This is initially well tolerated; forward stroke volume is maintained by compensatory right ventricular dilatation. However, over time, progressive right ventricular dilatation exacerbates tricuspid regurgitation and leads to right ventricular systolic failure. Pulmonary arterial hypertension is not a feature of structural tricuspid regurgitation. In contrast, functional tricuspid regurgitation commonly arises as a consequence of pulmonary arterial hypertension. In this situation, patients develop right ventricular volume and pressure overload (see Chapter 20).
Surgical Treatment and Postoperative Care
Patients with severe tricuspid regurgitation due to structural tricuspid valve disease may be considered for isolated tricuspid surgery. Ideally, valve repair is performed, but often valve replacement is the only option. The major postoperative problem is right ventricular failure. Gross dilatation of the right ventricle also causes impaired filling of the left ventricle. Patients develop high systemic venous pressure and low cardiac output. Damage to the atrioventricular node at the time of surgery can cause complete heart block. The treatment of right ventricular failure is described in Chapter 20. The mortality for tricuspid valve replacement is high: about 20%.20–22
Pulmonary Valve Disease
Disease of the pulmonary valve virtually always occurs in the context of congenital cardiac disease. It is discussed in Chapter 15.
Mixed and Multivalve Disease
The presence of mixed or multiple valve lesions complicates hemodynamic management, particularly when regurgitant and stenotic lesions coexist. Therapy should be directed to the hemodynamically significant lesion, which in many circumstances is the downstream lesion. The mortality rates for double-valve surgery are almost twice those of single-valve surgery,23 and postoperative problems involving bleeding, ventricular dysfunction, atrial fibrillation, and pulmonary hypertension are more common.
REOPERATION
Repeat sternotomy carries the risk of damaging a cardiac or vascular structure but unlike reoperative CABG surgery, there is no risk for damaging coronary grafts. A lateral chest radiograph should be obtained preoperatively to assess the size of the anterior mediastinal air space. Additional blood should be cross-matched for transfusion. Reoperative valve surgery may not require dissection and mobilization of the whole heart (unlike reoperative CABG surgery). Postoperative bleeding and ventricular dysfunction are more common than they are after first-time surgery. The mortality rates associated with reoperative single-valve surgery are about 5% to 8%,12,24 which is higher than the rates reported for first-time, single-valve surgery, but only slightly.
SURGERY FOR ATRIAL FIBRILLATION
A number of surgical procedures have been developed for the treatment of atrial fibrillation. The gold standard is the maze III procedure developed by Cox, which has a cure rate of more than 95%.25 In this procedure, multiple surgical incisions are created over the left and right atria to interrupt reentrant circuits and to channel conduction along predetermined pathways. The procedure is complex, requires an additional 45 to 60 minutes of CPB, and has not been widely adopted. Numerous modifications have been made to the maze III procedure; they involve different incisions and the use of alternatives to the traditional cut-and-sew technique for achieving electrical isolation, in particular, the use of cryotherapy and irrigated thermal radiofrequency probes to create transmural scars.
It is now recognized that in most patients, the initiating trigger of atrial fibrillation is rapid focal discharges from cells at the junction of the pulmonary veins and left atrium.26 This has led to the development, also by Cox, of the mini-maze procedure (Fig. 10-8).27 The mini-maze consists of a pulmonary-vein encircling lesion and a lesion between the inferior pulmonary veins and the mitral annulus (the left atrial isthmus lesion). In addition, to prevent atrial flutter, a right atrial isthmus lesion is placed between the coronary sinus and the tricuspid valve. The success rate for the mini-maze procedure is likely to be lower than that of the full maze III, but the operation is considerably simpler and can be performed epicardially and with the atrium closed. Some surgeons now perform a mini-maze-type operation endoscopically, without the use of CPB.
Operative mortality rates after the maze III procedure are 6% to 10%,25,28 although they are less than 1% when the procedure is performed in isolation. Approximately 40% of patients experience postoperative atrial fibrillation, so routine drug prophylaxis is recommended. The success or lack of success of the procedure cannot be judged until 6 months postoperatively. A small percentage of patients are left with persistent sinus node dysfunction and require permanent pacemaker insertion.28
There is some concern regarding the effect of the loss of the atrial appendage that occurs with the maze III procedure (but not routinely with the mini-maze). Excision of the atrial appendage results in reduced secretion of atrial natriuretic peptide,29 which may exacerbate postoperative fluid retention.
ANTICOAGULATION
For patients with prosthetic valves, some centers also recommend anticoagulation with heparin as soon as perioperative bleeding is controlled and until anticoagulation with warfarin becomes therapeutic, but this approach is controversial.13 Cardiac surgery patients are very sensitive to warfarin, particularly if they are receiving amiodarone prophylaxis for atrial fibrillation. Thus, warfarin should be commenced at a low dose (3 to 5 mg/day) on the first postoperative day and carefully titrated to the INR.
Cardiac surgery patients who have been treated with anticoagulants are at high risk for bleeding after invasive procedures (e.g., percutaneous tracheostomy) or as a consequence of gastrointestinal tract stress ulceration. In unstable patients who require prolonged ICU stay (and who therefore may need invasive medical procedures), it is prudent to avoid commencing warfarin in the early postoperative period and to manage anticoagulation with unfractionated heparin. Prior to invasive procedures, heparin may be withheld for 2 to 4 hours or until clotting times have returned to normal. For emergency procedures, or in the case of important bleeding, heparin may be reversed with protamine.
For patients receiving warfarin who require surgery or invasive procedures, the INR should be less than 1.5. To achieve this, warfarin may need to be withheld for 3 to 5 days. In patients at high risk for thrombosis, heparin should be commenced when the INR falls below 2. Strong consideration should also be given to stress ulcer prophylaxis (e.g., omeprazole 40 mg daily) in patients being given anticoagulation medications, especially if they are ventilated for longer than 48 hours.30
ENDOCARDITIS PROPHYLAXIS
The risk for developing endocarditis after an invasive procedure is determined by the nature of the procedure and the underlying cardiac lesion (Table 10-7). In general, invasive procedures carried out in the ICU (e.g., endotracheal intubation, urethral catheterization, insertion of vascular catheters) do not require antibiotic prophylaxis, even in patients who are at high risk.13 Antibiotic prophylaxis is optional (i.e., determined on a case-by-case basis) in high-risk patients undergoing TEE, upper gastrointestinal endoscopy, and flexible bronchoscopy.17 Prophylaxis is recommended for procedures associated with a high likelihood of mucosal injury of the oral cavity (e.g., dental extractions), the respiratory tract (e.g., rigid bronchoscopy), the gastrointestinal tract (e.g., bowel surgery), and the genitourinary tract (e.g., cystoscopy).17 Antibiotic guidelines for specific procedures are provided in Table 10-8.17 (These guidelines are currently under review by the American Heart Association.)13
Table 10-7 Risk for Endocarditis Associated with Various Cardiac Lesions
High Risk |
Prosthetic heart valves |
Previous bacterial endocarditis |
Complex cyanotic congenital heart disease |
Surgically constructed systemic to pulmonary shunts |
Moderate Risk |
Acquired valve dysfunction |
Obstructive hypertrophic cardiomyopathy |
Mitral valve prolapse with mitral regurgitation |
Most other congenital cardiac malformations |
Low or Negligible Risk |
Secundum atrial septal defect |
Surgical repair of an atrial septal defect, a ventricular septal defect, or a patent ductus arteriosus |
Mitral valve prolapse without mitral regurgitation |
Previous coronary artery bypass graft surgery |
Pacemakers or implanted defibrillators |
Physiologic or innocent murmurs |
Table 10-8 Antibiotic Guidelines for Endocarditis Prophylaxis
Standard general prophylaxis | Amoxicillin 2 g orally 1 hr before procedure |
Unable to take oral medication | Ampicillin 2 g intravenously within 30 mins of procedure |
Penicillin allergy | Clindamycin 600 mg orally 1 hr before procedure |
Penicillin allergy and unable to take oral medication | Clindamycin 600 mg intravenously within 30 min of procedure or cefazolin 1 g intravenously within 30 min of procedure |
From Bonow RO, Carabello B, de Leon AC, et al: ACC/AHA guidelines for the management of patients with valvular heart disease. A report of the American College of Cardiology/American Heart Association Task Force on Practice Guidelines (Committee on Management of Patients With Valvular Heart Disease). J Am Coll Cardiol 32:1497, 1998.
1 Yu HY, Ho YL, Chu SH, et al. Long-term evaluation of Carpentier-Edwards porcine bioprosthesis for rheumatic heart disease. J Thorac Cardiovasc Surg. 2003;126:80-89.
2 Hammermeister KE, Sethi GK, Henderson WG, et al. A comparison of outcomes in men 11 years after heart-valve replacement with a mechanical valve or bioprosthesis. Veterans Affairs Cooperative Study on Valvular Heart Disease. N Engl J Med. 1993;328:1289-1296.
3 Rizzoli G, Bottio T, Thiene G, et al. Long-term durability of the Hancock II porcine bioprosthesis. J Thorac Cardiovasc Surg. 2003;126:66-74.
4 Raja SG, Pozzi M. Growth of pulmonary autograft after Ross operation in pediatric patients. Asian Cardiovasc Thorac Ann. 2004;12:285-290.
5 Luciani GB, Casali G, Favaro A, et al. Fate of the aortic root late after Ross operation. Circulation. 2003;108(suppl 1):II61-II67.
6 Ortlepp JR, Schmitz F, Bozoglu T, et al. Cardiovascular risk factors in patients with aortic stenosis predict prevalence of coronary artery disease but not of aortic stenosis: an angiographic pair-matched case-control study. Heart. 2003;89:1019-1022.
7 Khot UN, Novaro GM, Popovic ZB, et al. Nitroprusside in critically ill patients with left ventricular dysfunction and aortic stenosis. N Engl J Med. 2003;348:1756-1763.
8 Gerber IL, Stewart RA, Legget ME, et al. Increased plasma natriuretic peptide levels reflect symptom onset in aortic stenosis. Circulation. 2003;107:1884-1890.
9 Bergler-Klein J, Klaar U, Heger M, et al. Natriuretic peptides predict symptom-free survival and postoperative outcome in severe aortic stenosis. Circulation. 2004;109:2302-2308.
10 Vahanian A, Palacios IF. Percutaneous approaches to valvular disease. Circulation. 2004;109:1572-1579.
11 Jamieson WR, Edwards FH, Schwartz M, et al. Risk stratification for cardiac valve replacement. National Cardiac Surgery Database. Database Committee of The Society of Thoracic Surgeons. Ann Thorac Surg. 1999;67:943-951.
12 Potter DD, Sundt TM3rd, Zehr KJ, et al. Operative risk of reoperative aortic valve replacement. J Thorac Cardiovasc Surg. 2005;129:94-103.
13 Bonow RO, Cavabello B, Chatterjee K, et al. ACC/AHA Guidelines for the management of patients with valvular heart disease. A report of the American College of Cardiology/American Heart Association Task Force on Practice Guidelines (Writing Committee to revise the 1998 guidelines for management of patients with valvular heart disease). J Am Coll Cardiology. 2006;48:1-148.
14 Birnbaum Y, Chamoun AJ, Conti VR, et al. Mitral regurgitation following acute myocardial infarction. Coron Artery Dis. 2002;13:337-344.
15 Henry WL, Morganroth J, Pearlman AS, et al. Relation between echocardiographically determined left atrial size and atrial fibrillation. Circulation. 1976;53:273-279.
16 DeAnda AJr, Kasirajan V, Higgins RS. Mitral valve replacement versus repair in 2003: where do we stand ? Curr Opin Cardiol. 2003;18:102-105.
17 Committee on Management of Patients with Valvular Heart Disease. ACC/AHA guidelines for the management of patients with valvular heart disease. A report of the American College of Cardiology/American Heart Association. Task Force on Practice Guidelines (Committee on Management of Patients with Valvular Heart Disease). J Am Coll Cardiol. 1998;32:1486-1588.
18 Dilip D, Chandra A, Rajashekhar D, et al. Early beneficial effect of preservation of papillo-annular continuity in mitral valve replacement on left ventricular function. J Heart Valve Dis. 2001;10:294-300.
19 Reyes VP, Raju BS, Wynne J, et al. Percutaneous balloon valvuloplasty compared with open surgical commissurotomy for mitral stenosis. N Engl J Med. 1994;331:961-967.
20 Ratnatunga CP, Edwards MB, Dore CJ, et al. Tricuspid valve replacement: UK Heart Valve Registry mid-term results comparing mechanical and biological prostheses. Ann Thorac Surg. 1998;66:1940-1947.
21 Kaplan M, Kut MS, Demirtas MM, et al. Prosthetic replacement of tricuspid valve: bioprosthetic or mechanical. Ann Thorac Surg. 2002;73:467-473.
22 Do QB, Pellerin M, Carrier M, et al. Clinical outcome after isolated tricuspid valve replacement: 20-year experience. Can J Cardiol. 2000;16:489-493.
23 Jamieson SW, Kapelanski DP, Sakakibara N, et al. Pulmonary endarterectomy: experience and lessons learned in 1,500 cases. Ann Thorac Surg. 2003;76:1457-1462.
24 Akins CW, Buckley MJ, Daggett WM, et al. Risk of reoperative valve replacement for failed mitral and aortic bioprostheses. Ann Thorac Surg. 1998;65:1545-1551.
25 Cox JL, Ad N, Palazzo T, et al. Current status of the maze procedure for the treatment of atrial fibrillation. Sem Thorac Cardiovasc Surg. 2000;12:15-19.
26 Haissaguerre M, Jais P, Shah DC, et al. Spontaneous initiation of atrial fibrillation by ectopic beats originating in the pulmonary veins. N Engl J Med. 1998;339:659-666.
27 Cox JL. Atrial fibrillation II: rationale for surgical treatment. J Thorac Cardiovasc Surg. 2003;126:1693-1699.
28 McCarthy PM, Gillinov AM, Castle L, et al. The Cox-maze procedure: the Cleveland Clinic experience. Sem Thorac Cardiovasc Surg. 2000;12:25-29.
29 Stollberger C, Schneider B, Finsterer J. Elimination of the left atrial appendage to prevent stroke or embolism ? Anatomic, physiologic, and pathophysiologic considerations. Chest. 2003;124:2356-2362.
30 Cook DJ, Fuller HD, Guyatt GH, et al. Risk factors for gastrointestinal bleeding in critically ill patients. Canadian Critical Care Trials Group. N Engl J Med. 1994;330:377-381.