Chapter 11 Tumors in Eloquent Areas of Brain
Rationale for Aggressive Resection
Surgery for diffuse tumor masses such as oligodendrogliomas and astrocytomas involves consideration of another dimension, that being resection of tumor infiltrating functional brain. It is well recognized that these diffuse tumors extend into otherwise grossly normal-appearing or slightly gliotic and potentially eloquent areas.1–3 The identification of this functional brain within the tumor margin is important for ensuring continued neurologic function. The topic concerning the benefits of extent of resection with respect to high- and low-grade gliomas is controversial. Unfortunately, there are no prospective, randomized controlled trials to specifically address the benefits of radical resection. We are thus restricted to considering nonrandomized, retrospective data in an effort to guide clinical therapy. It cannot be overemphasized that decision making must be individualized and is best undertaken with a multidisciplinary approach to each patient considering the risks and benefits of each specific treatment option. Any benefit of surgery will come only by way of minimizing operative and neurologic morbidity related to the treatment.
The literature, overall, supports a positive effect of surgery on the natural history of low-grade gliomas. In a number of studies, gross or near-gross total resection of low-grade astrocytomas was correlated with lower recurrence rates and longer times to progression as compared with subtotal resection.4–10 Fewer studies have correlated extent of resection with a survival advantage.11 The rationale for aggressive resection is based on the assumption that small tumors will with time progress to larger tumors and become potentially either more difficult to resect or nonresectable. There is also good evidence to suggest that the potential for malignant degeneration is related to the tumor size and length of time the mass has been present.4,12 Malignant degeneration has been variously reported, and probably occurs in about 50% of patients harboring these lesions.13,14 An exhaustive review of this literature is beyond the scope of this chapter, and the reader is referred to a recent review by Keles and colleagues.15 Surgery for high-grade gliomas is less controversial. Several studies not only indicate a benefit for time to progression and improved neurologic performance, but also improved survival.16–26 Newer evidence, however, emphasizes that acquired motor or speech deficits may negatively impact overall survival.27
Definitions of Eloquent Cortex
The concept of cortical localization with respect to language dates back to Broca’s seminal report of two patients with nonfluent aphasia after having suffered autopsy-proven left inferior frontal strokes.28 Wernicke subsequently added the description of another form of aphasia, variously termed fluent aphasia, in 1874 under similar circumstances.29 These experiments of nature have opened the door to extensive research efforts at anatomically localizing various aspects of language and motor function to specific regions of the cerebral cortex. Numerous types of language deficit can result from injury both to cortical and subcortical regions. The focus here will be directed toward those regions essential for language and motor function as it applies to neurosurgical procedures and practice.
Sir Victor Horsley30 performed the first stimulation mappings of the cerebral cortex in a series of patients when he stimulated the motor and somatosensory cortex producing contralateral limb movement and paresthesias. About 50 years later, Penfield and Boldrey detailed their results in stimulation of the precentral and postcentral gyri and noted that primary motor and sensory function is reliably located in these regions, although they may be displaced from their usual anatomic location as a result of mass effect from adjacent tissue.31 They found these areas to be indispensable for movement and emphasized that during surgery every effort to preserve their structural integrity should be made. An exception to this general rule may be the face region, which is represented bilaterally, and resection of lesions in the nondominant motor cortex have been described.32 This is not advocated in the dominant hemisphere because of the close proximity of language areas and the greater role the face motor region may play as association cortex with the language areas.
The most extensive work done on mapping primary essential language areas up to recent years was done by Penfield and colleagues as well.33,34 Penfield tested naming, counting, spelling, and reading in patients during awake craniotomy and documented these areas on maps of the lateral and superior cortical surface. Three main areas were identified as being essential for language function. These were the inferior frontal opercular area (corresponding to Broca’s area), the posterior temporal area (corresponding to Wernicke’s area), and a third area located on the medial and superior surfaces of the superior frontal gyrus (the supplementary motor area). They also noted that these sites had relative importance in subserving language function, with the posterior temporal and inferior frontal regions being unresectable if language function was to be preserved. They also noted that the mesial frontal region was associated with severe expressive deficits postoperatively but that these deficits gradually disappeared in the weeks following surgery. Numerous others have confirmed this observation, indicating that resection of the supplementary motor area does not generally lead to permanent deficits as long as the more posterior primary motor cortex is spared.35,36
The standard technique for intraoperative stimulation mapping was also developed by Penfield to a large extent and later refined by Ojemann et al.37 Of note in Penfield’s stimulation maps is that errors in naming and object recognition were indeed clustered in the classical Broca’s and Wernicke’s areas, but a significant number of sites occurred outside of these traditional boundaries. In classic mapping studies done by Ojemann and colleagues on 117 patients, 67% had more than one distinct essential language area and 24% had three or more distinct areas subserving language function in the dominant hemisphere peri-sylvian region. There was also greater variability in the temporal language area as compared with the frontal region. Additionally, essential language sites were found to be confined to an area of 2.5 cm2 or less in about 50% of people, with only 16% of patients having an area equal to or larger than 6 cm237 Seldom are the entire extents of classical language areas essential for language function thus allowing potentially aggressive resection of lesions in eloquent areas.
Testing of Cerebral Dominance
The evaluation of cerebral dominance has interested scientists since the time of Broca. It is well established that the vast majority of right-handed individuals are left brain dominant for speech and language function, while fewer left-handed individuals are left brain dominant, but still a majority. Efforts at finding out which patients may have atypical language localization (i.e., bilateral or right sided) is of great importance to the neurosurgeon particularly when operating in the peri-sylvian region of the right hemisphere. Strauss and Wada evaluated patients for various lateralized preferences, including hand, foot, eye, and ear, and found that taken together, these had a higher correlation with cerebral dominance than any one did alone. Perhaps more revealing in this study was that only 3% of patients with all left-sided preferences were left hemisphere dominant, indicating a high-risk group for undergoing right-sided surgical procedures.38 Noninvasive means of testing for dominance offer clues about the lateralization of cerebral dominance, but application to a broad population base is problematic. These evaluations alone cannot be used to predict cerebral dominance reliably in individual patients, especially those with any left-sided preference. The intracarotid amobarbital procedure is the method of choice for the determination of dominance in this setting.
The intracarotid amobarbital procedure was initially developed by Wada in 194939 and later applied to a larger number of patients in reports by Wada and Rasmussen40 and Branch and colleagues41 for determining cerebral dominance with respect to language function. Milner and colleagues applied the technique to study the dominance of memory in patients undergoing resection of mesial temporal lobe structures for epilepsy.42 There have been numerous other applications of this technique, but the focus of this chapter will be on testing for language and memory dominance as it applies to lesions in eloquent cortex.
Anatomic Localization of Eloquent Areas
Several methods have been described for localizing the central sulcus based on external (skull) landmarks. This gives the surgeon a general idea of where the precentral and postcentral gyri are located preoperatively and can help in planning the craniotomy in the absence of neuronavigational aids. These techniques are based on Taylor-Haughton lines.43 The motor strip is typically located 4 to 5 cm posterior to the coronal suture in the midsagittal plane.44
The central sulcus is often readily identified on preoperative imaging. Berger and colleagues correlated intraoperative stimulation mapping of motor cortex with preoperative MRI scans and found that the central sulcus is reliably identified on high-vertex axial T2-weighted imaging as transverse sulci with the motor cortex located immediately anterior (Fig. 11-1). Additionally, it was found less reliably on slightly parasagittal images using the termination of the cingulate sulcus in the marginal sulcus with the sensorimotor cortex anterior to this.45 Efforts at localizing language areas have been less certain, although for Broca’s area, which is more constant in location, Quiñones-Hinojosa and colleagues have demonstrated 87% to 89% accuracy when the frontal opercular area is categorized in specific anatomic subtypes when compared to intraoperative stimulation mapping.46 This may be readily identified on sagittal imaging as an “M-shaped” gyrus representing the pars orbitalis, triangularis, and opercularis (Fig. 11-2).
Intraoperative Stimulation Mapping
Localization of eloquent areas of the brain during surgery is of paramount importance both in preserving function and in ensuring the most radical resection possible when lesions are in close proximity to them. Furthermore, tumors may abut eloquent cortex, displacing it and making landmarks more difficult to identify, or they may invade critical structures. Intraoperative cortical stimulation is widely used and has been validated in numerous studies. It is currently the “gold standard” in the identification and preservation of eloquent areas to which all other modalities such as functional imaging should be compared.
Localization of Primary Motor and Sensory Cortex
The method of using somatosensory evoked potentials to identify the sensorimotor cortex was introduced by Goldring in treating pediatric epilepsy patients.47 It has since broadened to include use in patients having tumors in the rolandic region.48,49 Somatosensory evoked potential mapping is quick and reliable in identifying the somatosensory region. Typically, an eight-contact strip electrode array is placed over the region of interest in a transverse orientation, and stimulation of the median or tibial nerve, depending on the lesion location, is done with recording of the contralateral cortical surface, either epidurally or subdurally. The somatosensory cortex is located at the point of phase reversal between two adjacent contacts. The array of electrodes may then be repositioned to confirm the location of the central sulcus superiorly or inferiorly.
Motor-evoked potentials have more recently been used to identify motor cortex specifically and allow direct stimulation monitoring of motor cortex and subcortical pathways with a high-frequency stimulator in patients under general anesthetic by observing limb movements or with electromyography. 48,50,51
Cortical stimulation mapping can be used to map the rolandic cortex with great precision (Figs. 11-3 to 11-5). Mapping of the motor cortex can be done with the patient either awake or under general anesthesia. Somatosensory stimulation can only be done with the patient awake. Stimulation may involve the cortex or subcortical white matter, which may be especially advantageous for tumors that extend deeply into the hemisphere or in the region of the internal capsule in the case of insular masses. Notably, children may have relatively inexcitable cortex as compared to adults, making stimulation mapping more difficult.52
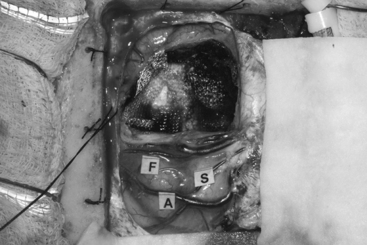
FIGURE 11-4 Intraoperative photograph of the patient in Figure 11-3 showing stimulation mapping of the right upper arm and leg region done before tumor removal. The resection was done with stereotactic guidance and ongoing neurologic examinations, both motor and speech, to protect the radiating white matter tracts during the resection of the deeper components of the tumor. A, arm; F, face; S, shoulder.