Chapter 11 Tumors in Eloquent Areas of Brain
Rationale for Aggressive Resection
Surgery for diffuse tumor masses such as oligodendrogliomas and astrocytomas involves consideration of another dimension, that being resection of tumor infiltrating functional brain. It is well recognized that these diffuse tumors extend into otherwise grossly normal-appearing or slightly gliotic and potentially eloquent areas.1–3 The identification of this functional brain within the tumor margin is important for ensuring continued neurologic function. The topic concerning the benefits of extent of resection with respect to high- and low-grade gliomas is controversial. Unfortunately, there are no prospective, randomized controlled trials to specifically address the benefits of radical resection. We are thus restricted to considering nonrandomized, retrospective data in an effort to guide clinical therapy. It cannot be overemphasized that decision making must be individualized and is best undertaken with a multidisciplinary approach to each patient considering the risks and benefits of each specific treatment option. Any benefit of surgery will come only by way of minimizing operative and neurologic morbidity related to the treatment.
The literature, overall, supports a positive effect of surgery on the natural history of low-grade gliomas. In a number of studies, gross or near-gross total resection of low-grade astrocytomas was correlated with lower recurrence rates and longer times to progression as compared with subtotal resection.4–10 Fewer studies have correlated extent of resection with a survival advantage.11 The rationale for aggressive resection is based on the assumption that small tumors will with time progress to larger tumors and become potentially either more difficult to resect or nonresectable. There is also good evidence to suggest that the potential for malignant degeneration is related to the tumor size and length of time the mass has been present.4,12 Malignant degeneration has been variously reported, and probably occurs in about 50% of patients harboring these lesions.13,14 An exhaustive review of this literature is beyond the scope of this chapter, and the reader is referred to a recent review by Keles and colleagues.15 Surgery for high-grade gliomas is less controversial. Several studies not only indicate a benefit for time to progression and improved neurologic performance, but also improved survival.16–26 Newer evidence, however, emphasizes that acquired motor or speech deficits may negatively impact overall survival.27
Definitions of Eloquent Cortex
The concept of cortical localization with respect to language dates back to Broca’s seminal report of two patients with nonfluent aphasia after having suffered autopsy-proven left inferior frontal strokes.28 Wernicke subsequently added the description of another form of aphasia, variously termed fluent aphasia, in 1874 under similar circumstances.29 These experiments of nature have opened the door to extensive research efforts at anatomically localizing various aspects of language and motor function to specific regions of the cerebral cortex. Numerous types of language deficit can result from injury both to cortical and subcortical regions. The focus here will be directed toward those regions essential for language and motor function as it applies to neurosurgical procedures and practice.
Sir Victor Horsley30 performed the first stimulation mappings of the cerebral cortex in a series of patients when he stimulated the motor and somatosensory cortex producing contralateral limb movement and paresthesias. About 50 years later, Penfield and Boldrey detailed their results in stimulation of the precentral and postcentral gyri and noted that primary motor and sensory function is reliably located in these regions, although they may be displaced from their usual anatomic location as a result of mass effect from adjacent tissue.31 They found these areas to be indispensable for movement and emphasized that during surgery every effort to preserve their structural integrity should be made. An exception to this general rule may be the face region, which is represented bilaterally, and resection of lesions in the nondominant motor cortex have been described.32 This is not advocated in the dominant hemisphere because of the close proximity of language areas and the greater role the face motor region may play as association cortex with the language areas.
The most extensive work done on mapping primary essential language areas up to recent years was done by Penfield and colleagues as well.33,34 Penfield tested naming, counting, spelling, and reading in patients during awake craniotomy and documented these areas on maps of the lateral and superior cortical surface. Three main areas were identified as being essential for language function. These were the inferior frontal opercular area (corresponding to Broca’s area), the posterior temporal area (corresponding to Wernicke’s area), and a third area located on the medial and superior surfaces of the superior frontal gyrus (the supplementary motor area). They also noted that these sites had relative importance in subserving language function, with the posterior temporal and inferior frontal regions being unresectable if language function was to be preserved. They also noted that the mesial frontal region was associated with severe expressive deficits postoperatively but that these deficits gradually disappeared in the weeks following surgery. Numerous others have confirmed this observation, indicating that resection of the supplementary motor area does not generally lead to permanent deficits as long as the more posterior primary motor cortex is spared.35,36
The standard technique for intraoperative stimulation mapping was also developed by Penfield to a large extent and later refined by Ojemann et al.37 Of note in Penfield’s stimulation maps is that errors in naming and object recognition were indeed clustered in the classical Broca’s and Wernicke’s areas, but a significant number of sites occurred outside of these traditional boundaries. In classic mapping studies done by Ojemann and colleagues on 117 patients, 67% had more than one distinct essential language area and 24% had three or more distinct areas subserving language function in the dominant hemisphere peri-sylvian region. There was also greater variability in the temporal language area as compared with the frontal region. Additionally, essential language sites were found to be confined to an area of 2.5 cm2 or less in about 50% of people, with only 16% of patients having an area equal to or larger than 6 cm237 Seldom are the entire extents of classical language areas essential for language function thus allowing potentially aggressive resection of lesions in eloquent areas.
Testing of Cerebral Dominance
The evaluation of cerebral dominance has interested scientists since the time of Broca. It is well established that the vast majority of right-handed individuals are left brain dominant for speech and language function, while fewer left-handed individuals are left brain dominant, but still a majority. Efforts at finding out which patients may have atypical language localization (i.e., bilateral or right sided) is of great importance to the neurosurgeon particularly when operating in the peri-sylvian region of the right hemisphere. Strauss and Wada evaluated patients for various lateralized preferences, including hand, foot, eye, and ear, and found that taken together, these had a higher correlation with cerebral dominance than any one did alone. Perhaps more revealing in this study was that only 3% of patients with all left-sided preferences were left hemisphere dominant, indicating a high-risk group for undergoing right-sided surgical procedures.38 Noninvasive means of testing for dominance offer clues about the lateralization of cerebral dominance, but application to a broad population base is problematic. These evaluations alone cannot be used to predict cerebral dominance reliably in individual patients, especially those with any left-sided preference. The intracarotid amobarbital procedure is the method of choice for the determination of dominance in this setting.
The intracarotid amobarbital procedure was initially developed by Wada in 194939 and later applied to a larger number of patients in reports by Wada and Rasmussen40 and Branch and colleagues41 for determining cerebral dominance with respect to language function. Milner and colleagues applied the technique to study the dominance of memory in patients undergoing resection of mesial temporal lobe structures for epilepsy.42 There have been numerous other applications of this technique, but the focus of this chapter will be on testing for language and memory dominance as it applies to lesions in eloquent cortex.
Anatomic Localization of Eloquent Areas
Several methods have been described for localizing the central sulcus based on external (skull) landmarks. This gives the surgeon a general idea of where the precentral and postcentral gyri are located preoperatively and can help in planning the craniotomy in the absence of neuronavigational aids. These techniques are based on Taylor-Haughton lines.43 The motor strip is typically located 4 to 5 cm posterior to the coronal suture in the midsagittal plane.44
The central sulcus is often readily identified on preoperative imaging. Berger and colleagues correlated intraoperative stimulation mapping of motor cortex with preoperative MRI scans and found that the central sulcus is reliably identified on high-vertex axial T2-weighted imaging as transverse sulci with the motor cortex located immediately anterior (Fig. 11-1). Additionally, it was found less reliably on slightly parasagittal images using the termination of the cingulate sulcus in the marginal sulcus with the sensorimotor cortex anterior to this.45 Efforts at localizing language areas have been less certain, although for Broca’s area, which is more constant in location, Quiñones-Hinojosa and colleagues have demonstrated 87% to 89% accuracy when the frontal opercular area is categorized in specific anatomic subtypes when compared to intraoperative stimulation mapping.46 This may be readily identified on sagittal imaging as an “M-shaped” gyrus representing the pars orbitalis, triangularis, and opercularis (Fig. 11-2).
Intraoperative Stimulation Mapping
Localization of eloquent areas of the brain during surgery is of paramount importance both in preserving function and in ensuring the most radical resection possible when lesions are in close proximity to them. Furthermore, tumors may abut eloquent cortex, displacing it and making landmarks more difficult to identify, or they may invade critical structures. Intraoperative cortical stimulation is widely used and has been validated in numerous studies. It is currently the “gold standard” in the identification and preservation of eloquent areas to which all other modalities such as functional imaging should be compared.
Localization of Primary Motor and Sensory Cortex
The method of using somatosensory evoked potentials to identify the sensorimotor cortex was introduced by Goldring in treating pediatric epilepsy patients.47 It has since broadened to include use in patients having tumors in the rolandic region.48,49 Somatosensory evoked potential mapping is quick and reliable in identifying the somatosensory region. Typically, an eight-contact strip electrode array is placed over the region of interest in a transverse orientation, and stimulation of the median or tibial nerve, depending on the lesion location, is done with recording of the contralateral cortical surface, either epidurally or subdurally. The somatosensory cortex is located at the point of phase reversal between two adjacent contacts. The array of electrodes may then be repositioned to confirm the location of the central sulcus superiorly or inferiorly.
Motor-evoked potentials have more recently been used to identify motor cortex specifically and allow direct stimulation monitoring of motor cortex and subcortical pathways with a high-frequency stimulator in patients under general anesthetic by observing limb movements or with electromyography. 48,50,51
Cortical stimulation mapping can be used to map the rolandic cortex with great precision (Figs. 11-3 to 11-5). Mapping of the motor cortex can be done with the patient either awake or under general anesthesia. Somatosensory stimulation can only be done with the patient awake. Stimulation may involve the cortex or subcortical white matter, which may be especially advantageous for tumors that extend deeply into the hemisphere or in the region of the internal capsule in the case of insular masses. Notably, children may have relatively inexcitable cortex as compared to adults, making stimulation mapping more difficult.52
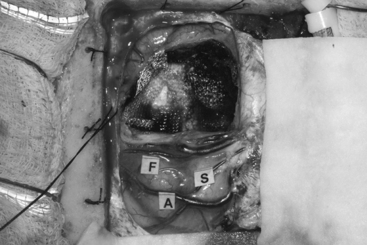
FIGURE 11-4 Intraoperative photograph of the patient in Figure 11-3 showing stimulation mapping of the right upper arm and leg region done before tumor removal. The resection was done with stereotactic guidance and ongoing neurologic examinations, both motor and speech, to protect the radiating white matter tracts during the resection of the deeper components of the tumor. A, arm; F, face; S, shoulder.
Stimulation Mapping Technique
Patient positioning is of great importance to balance the requirements of a multidisciplinary team during the surgical procedure. The patient must be comfortable enough with respect to head position and padding of pressure points to allow cooperation for often extended periods of time. He or she must have an unobstructed view of the examiner so as to participate fully in testing during the procedure. The anesthesiologist must also have ready access to the airway for emergency intubation as well as monitoring during pin placement and craniotomy, when the patient is under more sedation. In our practice, frameless stereotaxis is often used and, therefore, patient positioning must also consider line-of-sight issues of the infrared camera and stereotactic equipment.53 With these issues in mind, the surgeon must often determine if there will be adequate access to the lesion. Often, optimal positioning from the surgical standpoint is somewhat compromised, and judgment must be exercised about the feasibility of doing an awake procedure as opposed to using alternative methods such as extraoperative mapping. Usually, however, a satisfactory solution can be found, facilitating safe tumor resection.
The stereotactic navigation system can be used to outline the tumor and identify possible motor and sensory cortex to minimize the amount of time spent mapping as well as serving as a useful guide about the extent of tumor resection. Intraoperatively, the main concern with stereotaxis and the use of preoperative imaging is brain shift. As the operation progresses, this distortion increases as cerebrospinal fluid is lost and the tumor is resected. This distortion is accentuated when the tumor is large or when the ventricle is entered. Intraoperative ultrasound is an alternative method to ensure maximal resection after mapping has been done to localize the tumor boundaries as well as potentially allowing correction due to brain shift.54 Small tickets or catheters may be used to outline the depth of the tumor around the periphery in a “picket fence” arrangement, with resection proceeding up the edge of each catheter. This minimizes the chance that brain shift will have an adverse effect on tumor resection. Ultrasound has shown good correlation to T2 signal abnormality on MRI.55
Standard cortical mapping is then done with the Ojemann stimulator, as has been described previously.52 An established anesthesia protocol should be in place in anticipation of the rare instance that stimulation induces a seizure, especially if the patient’s head is fixed in a pinion. To minimize induction of intraoperative seizure activity, a surface electrocorticography strip is placed outside the resection field on the cortical surface to monitor for afterdischarges. Stimulation current is selected to be less than that which results in 1 or 2 afterdischarges. If there is evidence of cortical irritability following stimulation, the brain’s surface is irrigated with ice-cooled saline. A low setting (2–5 mA) constant current, 60-Hz biphasic square wave stimulus with a 1-msec duration is used to stimulate various regions of interest. Motor stimulation may have to be higher than sensory stimulation. If no response is elicited at 16 mA, then no functional cortex is located in the stimulated area. A quick test of the temporalis muscle reflected from the craniotomy site can confirm that the equipment is functional in sleeping patients. Electromyography can be used when performing mapping of the motor cortex to provide greater sensitivity and lessen the chances of stimulation induced seizure activity.56 Contact of the bipolar stimulator, parallel to the adjacent sulci, should last 1 to 2 seconds, and no two stimulation trials should be attempted in succession in one area. Current may need to be increased to identify certain areas such as the face motor region and depends on the anesthesia used if the patient is asleep. Pediatric patients also may require higher stimulation current to elicit a response, as noted earlier. The patient is assessed for motor or sensory findings with each stimulus when mapping the perirolandic areas. The cortex is stimulated in stepwise fashion at 1.5-cm intervals, with two to three positive stimulations defining functional cortex.53 A numbered tag is then placed on the brain surface at these sites. Subcortical tracts may also be stimulated similarly during tumor resection with the same or slightly higher current parameters.57–60 This may be particularly useful for insular gliomas adjacent to the internal capsule or medial temporal tumors growing over the tentorial edge to identify the cerebral peduncle at the medial extent of the resection.
Language mapping is done similarly, with a speech pathologist examining the patient with confrontational naming, spelling, counting, reading, or other site-specific test. The patient is shown objects or assessed every 4 seconds with any errors, anomia, dysnomia, hesitation, or speech arrest being noted. After each stimulation trial, the patient is allowed to name an object without stimulation to ensure recovery of function. Afterdischarges are allowed to dissipate prior to the next stimulation. It is important to note when stimulating the posterior inferior frontal lobe that speech arrest is not caused by oropharyngeal motor arrest, as occurs when stimulating the precentral gyrus, by observing the patient as well as listening. Stimulation of the postcentral gyrus may aid in this distinction, because the patient is able to note oropharyngeal sensory stimuli. Cortical sites essential for language function have been found to be located on the crests of gyri and not generally in sulci unless continuous with an adjacent gyrus, according to Ojemann and co-workers.37
A limitation of intraoperative cortical mapping is that high-intensity stimulation may inhibit or activate functional areas whereas low-intensity stimulation may not identify intended target areas.61 Other pitfalls to be aware of are that more than two language areas can exist, and thus the inability to identify any eloquent cortex should raise caution that the stimulation is not working rather than lead to the conclusion that none exists in a given area. Also, preservation of cortex with disruption of subcortical tracts by undercutting gyri may lead to permanent morbidity.
It is often straightforward to identify by MRI the central sulcus or to map eloquent motor and language cortex as just described. However, it is more difficult to map the subcortical white matter tracts. One technique to minimize the risk of injury to radiating white matter tracts is to conduct repetitive neurologic and speech examinations during the ongoing tumor resection. This obviously requires a coordinated team approach, which includes having both a neurologist and speech pathologist, possibly with interpreters for mapping multiple language regions available in the operating room. With ongoing examinations, the surgeon is able to be more aggressive and proceeds with resection until the onset of neurologic deficits. In this circumstance, a maximum neurologically permissible resection of infiltrative tumors can be performed with a risk of significant neurologic injury that approximates 15%.53
There is evidence that epilepsy associated with slow-growing low-grade neoplasms resides in adjacent tumor-free cortex.62 Epilepsy associated with AVMs may be similar.63 With respect to lesions in eloquent cortex, this epileptogenic zone may also reside in functional cortex, hence the need to perform either extraoperative mapping as described later, or intraoperative electrocorticography to define this area. Multiple studies have shown that resection of adjacent electrocorticography-active foci results in improved seizure control as compared with resection of the tumor mass alone.62 Children may fare better in this regard than adults.64 Combining an epilepsy operation with an oncologic operation may provide the best chance at tumor and seizure control. It is also important to monitor for epileptic discharges after resection to ensure that additional seizure foci are not left behind.
Intraoperative cortical mapping combined with appropriate functional imaging and stereotactic neuronavigation should theoretically protect the patient from iatrogenic neurologic morbidity. This, however, is not always the case, and there are several possible reasons. First, patients with lesions in eloquent areas already have some degree of neurologic impairment, and manipulation or close resection to critical areas may worsen the neurologic condition. Additionally, maximizing resection by removing tumor until a deficit becomes apparent is a strategy sometimes used.8,52 Second, regional ischemia and peritumoral edema may become manifest after an apparently uneventful resection.65 Third, a lack of specificity of the testing paradigm to the area of resection may miss potentially eloquent areas. This is minimized by using naming as a part of language evaluation, as the majority of aphasias have anomia as a component of the syndrome. More specific tests, however, can be done when there is concern about important association cortex or functions such as calculation.66 Complex language functions may in time be better identified with more specific testing paradigms used after assessment with functional MRI (fMRI), evaluating the specific functional modalities at risk during lesion resection.67
Resection of gliomas to within 2 cm of eloquent tissue used in naming carries a risk of persistent postoperative deficit, as noted by Ojemann and Dodrill.68 Haglund and colleagues later reported on a series of patients undergoing temporal glioma resection that a margin of greater than 8 mm was associated with no postoperative deficits lasting more than 30 days.69 In general, it is best to keep a margin of about 1 cm between resection and eloquent cortex. Subcortical pathways from sensorimotor and language areas are thought to descend perpendicular to gyri; therefore, undercutting identified eloquent cortex should be avoided.
Functional Imaging
There has been an explosion in recent years in the research and application of functional imaging to neurosurgery. These techniques are based on identifying regions of the brain that are “active” relative to other regions of the brain during a specific testing algorithm. This technology is extremely helpful in that it offers the possibility of localizing eloquent areas of cortex with respect to a mass lesion preoperatively, determining the best surgical approach, and potentially guiding the decision to use intraoperative mapping in a given patient.
Functional MRI (fMRI) and positron emission tomography (PET) scanning are being used more frequently in the preoperative assessment of eloquent areas of the brain. Functional MR signal changes are believed to be a result of local blood oxygenation differences between activated and, therefore, more metabolically active, brain and relatively silent areas. This has been termed the blood oxygen level-dependent (BOLD) contrast method and requires no contrast agent70 (Fig. 11-6). Various paradigms exist for testing certain areas of the brain. All are dependent on a comparison with the performance of a task and a resting state or alternate task. fMRI studies and their interpretation, therefore, are extremely dependent on the tasks and comparisons used. While fMRI measures the changes in deoxyhemoglobin levels reflecting oxygen consumption, PET measures regional differences in cerebral blood flow through use of an injected radioisotope, most commonly [15O]H2O or 2-[18F]-2-desoxy-D-glucose (FDG). Despite the inherent differences in the physiologic basis for the imaging modalities, concordance between them when compared to intraoperative stimulation mapping has been good.71,72 In the future, fMRI may have more efficacy in comparison with PET because of its noninvasive nature and more widespread availability.
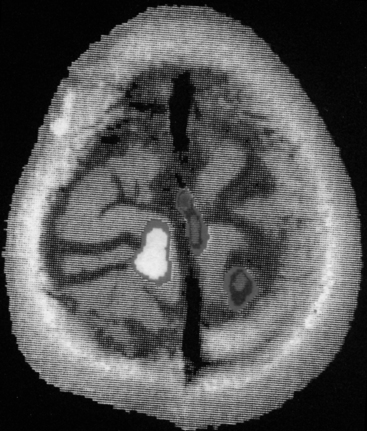
FIGURE 11-6 Functional magnetic resonance image using the BOLD technique for identifying motor cortex preoperatively.
Maps of eloquent areas identified on fMRI or PET can be coregistered or “fused” to standard MRI scans, slice by slice manually or with fusion software, to give better anatomic detail in most neuronavigational systems.73,74 This coregistration can in turn be applied in planning the surgical procedure, as well as during the formal operation. The potential benefit to using fMRI data is that it preoperatively determines the general location of eloquent cortex with respect to the lesion in question, allowing for preprocedural planning of the craniotomy and the approach to the tumor, as well as aiding in the decision whether to apply intraoperative stimulation. It may further reduce the size of the craniotomy, thus minimizing both surgical morbidity and the amount of time spent performing intraoperative mapping.75
More recently there has been interest in combining fMRI with diffusion-weighted MR images (diffusion tensor imaging [DTI]) to identify the motor cortex and pyramidal tracts with respect to space-occupying lesions.76,77 This may also provide additional insight into the preoperative planning and again reduce the time needed for intraoperative stimulation of subcortical tracts, which can be tedious and time consuming. It may also shed light on specific areas where the tumor mass may invade deep white matter tracts that must be spared during resective surgery. This information may be coregistered with MRI or fMRI imaging sets using neuronavigation at the time of surgery to maximize safety.
Several potential pitfalls should be taken into account when relying on fMRI, DTI, or PET data. There can be significant technical issues in integrating functional scans with a neuronavigational system with respect to echo-planar image distortion and complementary slice integration. This may lead to functional mislocalization of eloquent tissue.78 The areas identified by fMRI or PET utilized for a specific task are often much larger than those identified at surgery with electrical mapping. This poses a problem in deciphering on fMRI the areas that are essential for a given task and the areas that simply participate in a task but are nonessential. This has been more problematic in mapping language areas than in mapping sensorimotor regions.79,80 Additionally, local vasoreactivity in peritumoral brain may distort results that rely on vasoreactivity and oxygen consumption for producing data maps.81 A recent review by Giussani and colleagues nicely illustrates these complex issues.82
Bello L., Gallucci M., Fava M., et al. Intraoperative subcortical language tract mapping guides surgical removal of gliomas involving speech areas. Neurosurgery. 2007;60:67-80.
Berger M.S., Cohen W.A., Ojemann G.A. Correlation of motor cortex brain mapping data with magnetic resonance imaging. J Neurosurg. 1990;72:383-387.
Berger M.S., Kincaid J., Ojemann G.A., et al. Brain mapping techniques to maximize resection, safety, and seizure control in children with brain tumors. Neurosurgery. 1989;25:786-792.
Bittar R.G., Olivier A., Sadikot A.F., et al. Presurgical motor and somatosensory cortex mapping with functional magnetic resonance imaging and positron emission tomography. J Neurosurg. 1999;91:915-921.
Boling W., Olivier A., Fabinyi G. Historical contributions to the modern understanding of function in the central area. Neurosurgery. 2002;50:1296-1310.
Coenen V.A., Krings T., Weidemann J., et al. Sequential visualization of brain and fiber tract deformation during intracranial surgery with three-dimensional ultrasound: an approach to evaluate the effect of brain shift. Neurosurgery. 2005;56(Suppl 1):133-141.
Duffau H., Capelle L., Denvil D., et al. Usefulness of intraoperative electrical subcortical mapping during surgery for low-grade gliomas located within eloquent brain regions: functional results in a consecutive series of 103 patients. J Neurosurg. 2003;98:764-778.
Duffau H., Lopes M., Denvil D., et al. Delayed onset of the SMA syndrome after surgical resection of the mesial frontal lobe: a time course study using intraoperative mapping in an awake patient. Stereotact Funct Neurosurg. 2001;76:74-82.
Giussani C., Roux F.E., Ojemann J., et al. Is preoperative functional magnetic resonance imaging reliable for language areas mapping in brain tumor surgery? Review of language functional magnetic resonance imaging and direct cortical stimulation correlation studies. Neurosurgery. 2010;66:113-120.
Keles G.E., Lamborn K.R., Berger M.S. Low-grade hemispheric gliomas in adults: a critical review of extent of resection as a factor influencing outcome. J Neurosurg. 2001;95:735-745.
Lacroix M., Abi-Said D., Fourney D.R., et al. A multivariate analysis of 416 patients with glioblastoma multiforme: prognosis, extent of resection, and survival. J Neurosurg. 2001;95:190-198.
Laws E.R., Parney I.F., Huang W., et al. Survival following surgery and prognostic factors for recently diagnosed malignant glioma: data from the glioma outcomes project. J Neurosurg. 2003;99:467-473.
LeRoux P., Berger M.S., Wang K., et al. Low grade gliomas: comparison of intraoperative ultrasound characteristics with preoperative imaging studies. J Neurosurg. 1992;13:189-198.
McGirt M.J., Chaichana K.L., Attenello F.J., et al. Extent of surgical resection is independently associated with survivial in patients with hemispheric infiltrating low-grade gliomas. Neurosurgery. 2008;63:700-708.
McGirt M.J., Mukherjee D., Chaichana K.L., et al. Association of surgically acquired motor and language deficits on overall survival after resection of glioblastoma multiforme. Neurosurgery. 2009;65:463-469.
Meyer F.B., Bates L.M., Goerss S.J., et al. Awake craniotomy for aggressive resection of primary gliomas located in eloquent brain. Mayo Clin Proc. 2001;76:677-687.
Nimsky C., Ganslandt O., Hastreiter P., et al. Preoperative and intraoperative diffusion tensor imaging-based fiber tracking in glioma surgery. Neurosurgery. 2005;56:130-137.
Ojemann G., Ojemann J., Lettich E., et al. Cortical language localization in left, dominant hemisphere. J Neurosurg. 1989;71:316-326.
Proescholdt M.A., Macher C., Woertgen C., et al. A level of evidence in the literature concerning brain tumor resection. Clin Neurol Neurosurg. 2005;107:95-98.
Quiñones-Hinojosa A., Ojemann S.G., Sanai N., et al. Preoperative correlation of intraoperative cortical mapping with magnetic resonance imaging landmarks to predict localization of the Broca area. J Neurosurg. 2003;99:311-318.
Romstock J., Fahlbusch R., Ganslandt, et al. Localization of the sensorimotor cortex during surgery for brain tumours: feasibility and waveform patterns of somatosensory evoked potentials. J Neurol Neurosurg Psychiatry. 2002;72:221-229.
Roux F.E., Boulanouar K., Lotterie J.A., et al. Language functional magnetic resonance imaging in preoperative assessment of language areas: correlation with direct cortical stimulation. Neurosurgery. 2003;52:1335-1347.
Sawaya R. Extent of resection in malignant gliomas: a critical summary. J Neurooncol. 1999;42:303-305.
Schmidt M.H., Berger M.S., Lamborn K.R., et al. Repeated operations for infiltrative low-grade gliomas without intervening therapy. J Neurosurg. 2003;98:1165-1169.
Skirboll S.S., Ojemann G.A., Berger M.S., et al. Functional cortex and subcortical white matter located within gliomas. Neurosurgery. 1996;38:678-685.
1. Ojemann J.G., Miller J.W., Silbergeld D.L. Preserved function in brain invaded by tumor. Neurosurgery. 1996;39:253-259.
2. Skirboll S.S., Ojemann G.A., Berger M.S., et al. Functional cortex and subcortical white matter located within gliomas. Neurosurgery. 1996;38:678-685.
3. Kelly P.J., Daumas-Duport C., Kispert D.B., et al. Imaging-based stereotaxic serial biopsies in untreated intracranial glial neoplasms. J Neurosurg. 1987;66:865-874.
4. Berger M.S., Deliganis A.V., Dobbins J., et al. The effect of extent of resection on recurrence in patients with low grade cerebral hemisphere gliomas. Cancer. 1994;74:1784-1791.
5. Hirsch J.F., Rose C.S., Pierre-Kahn A., et al. Benign astrocytic and oligodendrocytic tumors of the cerebral hemispheres in children. J Neurosurg. 1989;70:568-572.
6. Berger M.S., Rostomily R.C. Low grade gliomas: functional mapping resection strategies, extent of resection, and outcome. J Neurooncol. 1997;34:85-101.
7. Nakamura M., Konishi N., Tsundoda S., et al. Analysis of prognostic and survival factors related to treatment of low-grade astrocytoma in adult. Oncology. 2000;58:108-116.
8. Peraud A., Meschede M., Eisner W., et al. Surgical resection of grade II astrocytomas in the superior frontal gyrus. Neurosurgery. 2002;50:966-977.
9. Piepmeier J., Christopher S., Spencer D., et al. Variations in the natural history and survival of patients with supratentorial low-grade astrocytomas. Neurosurgery. 1996;38:872-878.
10. Vives K.P., Piepmeier J.M. Complications and expected outcome of glioma surgery. J Neurooncol. 1999;42:289-302.
11. Soffietti R., Chio A., Giordana M.T., et al. Prognostic factors in well-differentiated cerebral astrocytomas in the adult. Neurosurgery. 1989;24:686-692.
12. Shafqat S., Hedley-White E.T., Henson J.W. Age-dependent rate of anaplastic transformation in low-grade astrocytoma. Neurology. 1999;52:867-869.
13. Laws E.R.Jr., Taylor W.F., Clifton M.B., et al. Neurosurgical management of low-grade astrocytoma of the cerebral hemispheres. J Neurosurg. 1984;61:665-673.
14. Schmidt M.H., Berger M.S., Lamborn K.R., et al. Repeated operations for infiltrative low-grade gliomas without intervening therapy. J Neurosurg. 2003;98:1165-1169.
15. Keles G.E., Lamborn K.R., Berger M.S. Low-grade hemispheric gliomas in adults: a critical review of extent of resection as a factor influencing outcome. J Neurosurg. 2001;95:735-745.
16. Ammirati M., Vick N., Liao Y., et al. Effect of the extent of surgical resection on survival and quality of life in patients with supratentorial glioblastomas and anaplastic astrocytomas. Neurosurgery. 1987;21:201-205.
17. Ciric I., Ammirati M., Vick N., et al. Supratentorial gliomas: surgical considerations and immediate postoperative results. Neurosurgery. 1987;21:21-26.
18. DeVaux B.C., O’Fallon J.R., Kelly P.J. Resection, biopsy and survival in malignant glial neoplasma: a retrospective study of clinical parameters, therapy, and outcome. J Neurosurg. 1993;78:767-775.
19. Lacroix M., Abi-Said D., Fourney D.R., et al. A multivariate analysis of 416 patients with glioblastoma multiforme: prognosis, extent of resection, and survival. J Neurosurg. 2001;95:190-198.
20. Nitta T., Sato K. Prognostic implications of the extent of surgical resection in patients with intracranial malignant gliomas. Cancer. 1995;75:2727-2731.
21. Sawaya R. Extent of resection in malignant gliomas: a critical summary. J Neurooncol. 1999;42:303-305.
22. Laws E.R., Parney I.F., Huang W., et al. Survival following surgery and prognostic factors for recently diagnosed malignant glioma: data from the glioma outcomes project. J Neurosurg. 2003;99:467-473.
23. Jeremic B., Grujicic D., Antunovic V., et al. Influence of extent surgery and tumor location on treatment outcome of patients with glioblastoma multiforme treated with combined modality approach. J Neurooncol. 1994;21:177-185.
24. Hess K.R. Extent of resection as a prognostic variable in the treatment of gliomas. J Neurooncol. 1999;42:227-231.
25. Proescholdt M.A., Macher C., Woertgen C., et al. A level of evidence in the literature concerning brain tumor resection. Clin Neurol Neurosurg. 2005;107:95-98.
26. McGirt M.J., Chaichana K.L., Attenello F.J., et al. Extent of surgical resection is independently associated with survivial in patients with hemispheric infiltrating low-grade gliomas. Neurosurgery. 2008;63:700-708.
27. McGirt M.J., Mukherjee D., Chaichana K.L., et al. Association of surgically acquired motor and language deficits on overall survival after resection of glioblastoma multiforme. Neurosurgery. 2009;65:463-469.
28. Broca P. Remarques sur le siège de la faculté du language articulé, suivies d’une observation d’aphémie (perte de la parole). Bull Soc Anat. 1861;36:330-357.
29. Wernicke C. Der Aphasische Symptomen Komplex. Breslau: cohn & Weigart. 1874.
30. Boling W., Olivier A., Fabinyi G. Historical contributions to the modern understanding of function in the central area. Neurosurgery. 2002;50:1296-1310.
31. Penfield W., Boldrey E. Somatic motor and sensory representation in the cerebral cortex of man as studied by electrical stimulation. Brain. 1937;60:389-443.
32. LeRoux P.D., Berger M.S., Haglund M.M., et al. Resection of intrinsic tumors from nondominant face motor cortex using stimulation mapping: report of two cases. Surg Neurol. 1991;36:44-48.
33. Penfield W., Jasper H. Epilepsy and the Functional Anatomy of the Human Brain. Boston: little, Brown & Co.; 1954.
34. Penfield W., Roberts L. Speech and Brian Mechanisms. Princeton, NJ: princeton University Press; 1959.
35. Rostomily R., Berger M., Ojemann G., et al. Postoperative deficits and functional recovery following removal of tumors involving the dominant hemisphere supplementing motor area. J Neurosurg. 1991;75:62-68.
36. Zentner J., Hufnagel A., Pechstein U., et al. Functional results after resective procedures involving the supplementary motor area. J Neurosurg. 1996;85:542-549.
37. Ojemann G., Ojemann J., Lettich E., et al. Cortical language localization in left, dominant hemisphere. J Neurosurg. 1989;71:316-326.
38. Strauss E., Wada J. Lateral preferences and cerebral speech dominance. Cortex. 1983;19:165-177.
39. Wada J. A new method for the determination of the side of cerebral speech dominance: a preliminary report of the intracarotid injection of sodium amytal in man. Igaku Seibutsugaki (Medicine and Biology). 1949;14:221-222.
40. Wada J., Rasmussen T. Intracranial injection of amytal for the lateralization of cerebral speech dominance. J Neurosurg. 1960;17:266-282.
41. Branch C., Milner B., Rasmussen T. Intracarotid sodium amytal for the lateralization of cerebral speech dominance: observations in 123 patients. J Neurosurg. 1964;21:399-405.
42. Milner B., Branch C., Rasmussen T. Study of short-term memory after intracarotid injection of sodium amytal. Trans Am Neurol Assoc. 1962;87:224-226.
43. Taylor A.J., Haughton V.M., Syvertsen A., et al. Taylor-Haughton line revisited. AJNR Am J Neuroradiol. 1980;1:55-56.
44. Kido D.K., LeMay M., Levinson A.W., et al. Computed tomographic localization of the precentral gyrus. Radiology. 1980;135:373-377.
45. Berger M.S., Cohen W.A., Ojemann G.A. Correlation of motor cortex brain mapping data with magnetic resonance imaging. J Neurosurg. 1990;72:383-387.
46. Quiñones-Hinojosa A., Ojemann S.G., Sanai N., et al. Preoperative correlation of intraoperative cortical mapping with magnetic resonance imaging landmarks to predict localization of the Broca area. J Neurosurg. 2003;99:311-318.
47. Goldring S. A method for surgical management of focal epilepsy, especially as it relates to children. J Neurosurg. 1978;49:344-356.
48. Cedzich C., Taniguchi M., Schafer S., et al. Somatosensory evoked potential phase reversal and direct motor cortex stimulation during surgery in and around the central region. Neurosurgery. 1996;38:962-970.
49. Romstock J., Fahlbusch R., Ganslandt, et al. Localization of the sensorimotor cortex during surgery for brain tumours: feasibility and waveform patterns of somatosensory evoked potentials. J Neurol Neurosurg Psychiatry. 2002;72:221-229.
50. Kombos T., Suess O., Funk T., et al. Intra-operative mapping of the motor cortex during surgery in and around the motor cortex. Acta Neurochir (Wien). 2000;142:263-268.
51. Cedzich C., Pechstein U., Schramm J., et al. Electrophysiological considerations regarding electrical stimulation of motor cortex and brain stem in humans. Neurosurgery. 1998;42:527-532.
52. Berger M.S., Kincaid J., Ojemann G.A., et al. Brain mapping techniques to maximize resection, safety, and seizure control in children with brain tumors. Neurosurgery. 1989;25:786-792.
53. Meyer F.B., Bates L.M., Goerss S.J., et al. Awake craniotomy for aggressive resection of primary gliomas located in eloquent brain. Mayo Clin Proc. 2001;76:677-687.
54. Coenen V.A., Krings T., Weidemann J., et al. Sequential visualization of brain and fiber tract deformation during intracranial surgery with three-dimensional ultrasound: an approach to evaluate the effect of brain shift. Neurosurgery. 2005;56(Suppl 1):133-141.
55. LeRoux P., Berger M.S., Wang K., et al. Low grade gliomas: comparison of intraoperative ultrasound characteristics with preoperative imaging studies. J Neurosurg. 1992;13:189-198.
56. Yingling C.D., Ojemann S., Dodson B., et al. Identification of motor pathways during tumor surgery facilitated by multichannel electromyographic recording. J Neurosurg. 1999;91:922-927.
57. Berger M.S., Ojemann G.A. Intraoperative brain mapping techniques in neuro-oncology. Stereotact Funct Neurosurg. 1992;58:153-161.
58. Berger M.S., Ojemann G.A., Lettich E. Neurophysiological monitoring during astrocytoma surgery. Neurosurg Clin North Am. 1990;1:65-79.
59. Duffau H., Capelle L., Denvil D., et al. Usefulness of intraoperative electrical subcortical mapping during surgery for low-grade gliomas located within eloquent brain regions: functional results in a consecutive series of 103 patients. J Neurosurg. 2003;98:764-778.
60. Bello L., Gallucci M., Fava M., et al. Intraoperative subcortical language tract mapping guides surgical removal of gliomas involving speech areas. Neurosurgery. 2007;60:67-80.
61. Carpentier A., Pugh K.R., Westerveld M., et al. Functional MRI of language processing: dependence on input modality and temporal lobe epilepsy. Epilepsia. 2001;42:1241-1254.
62. Berger M.S., Ghatan S., Geyer J.R., et al. Seizure outcome in children with hemispheric tumors and associated intractable epilepsy: the role of tumor removal combined with seizure foci resection. Pediatr Neurosurg. 1991;17:185-191.
63. Yeh H.S., Kashiwagi S., Tew J.M.Jr., et al. Surgical management of epilepsy associated with cerebral arteriovenous malformations. J Neurosurg. 1990;72:216-223.
64. Berger M.S., Ghatan S., Haglund M.M., et al. Low-grade gliomas associated with intractable epilepsy: seizure outcome utilizing electrocorticography during tumor resection. J Neurosurg. 1993;79:62-69.
65. Duffau H., Lopes M., Denvil D., et al. Delayed onset of the SMA syndrome after surgical resection of the mesial frontal lobe: a time course study using intraoperative mapping in an awake patient. Stereotact Funct Neurosurg. 2001;76:74-82.
66. Duffau H., Denvil D., Lopes M., et al. Intraoperative mapping of the cortical areas involved in multiplication and subtraction: an electrostimulation study in a patient with a left parietal glioma. J Neurol Neurosurg Psychiatry. 2002;73:733-820.
67. Whittle I.R., Borthwick S., Haq N. Brain dysfunction following “awake” craniotomy, brain mapping and resection of glioma. Br J Neurosurg. 2003;17:130-137.
68. Ojemann G.A., Dodrill C.G. Intraoperative techniques for reducing language and memory deficits with left temporal lobe lobectomy. In: wolf P., Dam M., Janz D., Dreifuss F. Advances in epileptology. New York: raven Press; 1987:327-330.
69. Haglund M.M., Berger M.S., Shamseldin M., et al. Cortical localization of temporal lobe language sites in patients with gliomas. Neurosurgery. 1994;34:567-576.
70. Ogawa S., Lee T.M., Kay A.R., et al. Brain magnetic resonance imaging with contrast dependent on blood oxygenation. Proc Natl Acad Sci U S A. 1990;87:9868-9872.
71. Bittar R.G., Olivier A., Sadikot A.F., et al. Presurgical motor and somatosensory cortex mapping with functional magnetic resonance imaging and positron emission tomography. J Neurosurg. 1999;91:915-921.
72. Ramsey N.F., Kirkby B.S., Van Gelderen P., et al. Functional mapping of human sensorimotor cortex with 3D BOLD fMRI correlates highly with H2[15]O PET rCBF. J Cereb Blood Flow Metab. 1996;16:755-764.
73. McDonald J.D., Chong B.W., Lewine J.D., et al. Integration of preoperative and intraoperative functional brain mapping in a frameless stereotactic environment for lesions near eloquent cortex. J Neurosurg. 1999;90:591-598.
74. Schulder M., Maldjian J.A., Liu W.C., et al. Functional image-guided surgery of intracranial tumors located in or near the sensorimotor cortex. J Neurosurg. 1998;89:412-418.
75. Rutten G.J., Ramsey N.F., van Rijen P.C., et al. Development of a functional magnetic resonance imaging protocol for intraoperative localization of critical temporoparietal language areas. Ann Neurol. 2002;51:350-360.
76. Krings T., Reinges M.H., Thiex R., et al. Functional and diffusion-weighted magnetic resonance images of space-occupying lesions affecting the motor system: imaging the motor cortex and pyramidal tracts. J Neurosurg. 2001;95:816-824.
77. Nimsky C., Ganslandt O., Hastreiter P., et al. Preoperative and intraoperative diffusion tensor imaging-based fiber tracking in glioma surgery. Neurosurgery. 2005;56:130-137.
78. Roux F.E., Ibarrola D., Tremoulet M., et al. Methodological and technical issues for integrating functional magnetic resonance imaging data in a neuronavigational system. Neurosurgery. 2001;49:1145-1156.
79. Roux F.E., Boulanouar K., Lotterie J.A., et al. Language functional magnetic resonance imaging in preoperative assessment of language areas: correlation with direct cortical stimulation. Neurosurgery. 2003;52:1335-1347.
80. Lehericy S., Duffau H., Cornu P., et al. Correspondence between functional magnetic resonance imaging somatotopy and individual brain anatomy of the central region: comparison with intraoperative stimulation in patients with brain tumors. J Neurosurg. 2000;92:589-598.
81. Roux F.E., Boulanouar K., Ranjeva J.P., et al. Usefulness of motor functional MRI correlated to cortical mapping in rolandic low-grade astrocytoma. Acta Neurochir (Wien). 1999;141:71-79.
82. Giussani C., Roux F.E., Ojemann J., et al. Is preoperative functional magnetic resonance imaging reliable for language areas mapping in brain tumor surgery? Review of language functional magnetic resonance imaging and direct cortical stimulation correlation studies. Neurosurgery. 2010;66:113-120.