31 Transfusion Medicine and Coagulation Disorders
Coagulation and bleeding assume particular importance when surgery is performed on the heart and great vessels using extracorporeal circulation. This chapter provides an understanding of the depth and breadth of hemostasis relating to cardiac procedures, beginning with coagulation pathophysiology. The pharmacology of heparin and protamine follows. This background is then applied to treatment of the bleeding patient. Coagulation monitoring is covered in Chapter 17, and fluid and blood management is further discussed in Chapter 30.
Overview of hemostasis
Proper hemostasis requires the participation of innumerable biologic elements (Box 31-1). This section groups them into four topics to facilitate understanding: coagulation factors, platelet function, the endothelium, and fibrinolysis. The reader must realize this is for simplicity of learning, and that in biology, the activation creates many reactions and control mechanisms, all interacting simultaneously. The interaction of the platelets, endothelial cells, and proteins to either activate or deactivate coagulation is a highly buffered and controlled process. It is perhaps easiest to think of coagulation as a wave of biologic activity occurring at the site of tissue injury (Figure 31-1).1 Although there are subcomponents to coagulation itself, the injury/control leading to hemostasis is a four-part event: initiation, acceleration, control, and lysis (recanalization/fibrinolysis). The initiation phase begins with tissue damage, which really is begun with endothelial cell destruction or dysfunction. This initiation phase leads to binding of platelets, as well as protein activations; both happen nearly simultaneously, and each has feedbacks into the other. Platelets adhere, creating an activation or acceleration phase that gathers many cells to the site of injury. From that adhesion a large number of events of cellular/protein messaging cascade. As the activation phase ramps up into an explosive set of reactions, counter-reactions are spun off, leading to control proteins damping the reactions. It is easiest, conceptually, to think of these control mechanisms as analogous to a nuclear reactor. The activation phase would continue to grow and overcome the whole organism unless control rods were inserted (e.g., thrombomodulin, proteins C and S, and tissue plasminogen activator [t-PA]) to stop the spread of the reaction. The surrounding normal endothelium acts quite differently from the disturbed (ischemic) endothelium. Eventually, the control reactions overpower the acceleration reactions and lysis comes into play. The diagram in Figure 31-1 shows lysis as relatively quick, but it can take 24 hours to days to have its full effects. A key concept is that hemostasis is part of a larger body system: inflammation. Most, if not all, of the protein reactions of coagulation control have importance in signaling inflammation leading to other healing mechanisms. Entire books have been written merely to examine these fascinating interactions. It is no wonder that cardiopulmonary bypass (CPB) has such profound inflammatory effects when it is considered that each of the activated coagulation proteins and cell lines then feeds into upregulation of inflammation.
During cardiac surgery, the endothelium (locally and systemically) is disturbed.2 The coronary arteries are made either partially or fully ischemic for periods with cardioplegia (perfused CPB being relatively rare today). Little known is that high concentrations of potassium are particularly insulting to endothelial cells. Ischemia/reperfusion injury is, therefore, the norm for every cardiac surgical case using CPB.3 Systemic ischemia and reperfusion occur throughout every capillary bed because microair, thrombus, and fat emboli are by-products of CPB4 (see Chapters 28 to 30 and 36). It is quite possible that transfusion of red blood cell (RBC) products, through blocking of the microcirculation, actually may contribute to ischemia/reperfusion injury. Transfusion has been shown to evoke endothelial cell hyper-reactivity mediated through cell membrane microparticles.4
Protein Coagulation Activations
Coagulation Pathways
The coagulation factors participate in a series of activating and feedback inhibition reactions, ending with the formation of an insoluble clot.5 A clot is the sum total of platelet-to-platelet interactions, leading to the formation of a platelet plug (initial stoppage of bleeding). The cross-linking of platelets to each other by way of the final insoluble fibrin leads to a stable clot. Clot is not simply the activation of proteins leading to more protein deposition. Clinicians have been shaped in their thinking about coagulation by the historic way that coagulation proteins were discovered and the resulting coagulation tests. It is that teaching of the coagulation cascade, with resultant monitoring technology, that has led to some transfusion behaviors. The way coagulation has been classically taught (protein cascades) is not the way coagulation proceeds biologically.
With few exceptions, the coagulation factors are glycoproteins (GPs) synthesized in the liver, which circulate as inactive molecules termed zymogens. Factor activation proceeds sequentially, each factor serving as substrate in an enzymatic reaction catalyzed by the previous factor in the sequence. Hence this classically has been termed a cascade or waterfall sequence. Cleavage of a polypeptide fragment changes an inactive zymogen to an active enzyme. The active form is termed a serine protease because the active site for its protein-splitting activity is a serine amino acid residue. Many reactions require the presence of calcium ion (Ca2+) and a phospholipid surface (platelet phosphatidylserine). The phospholipids occur most often either on the surface of an activated platelet or endothelial cell and occasionally on the surface of white cells. So anchored, their proximity to one another permits reaction rates profoundly accelerated (up to 300,000-fold) from those measured when the enzymes remain in solution. The factors form four interrelated arbitrary groups (Figure 31-2): the contact activation, intrinsic, extrinsic, and common pathways. They were so labeled historically by the human need for order. In biology, they are all highly interactive, occur simultaneously on the surface of cells, and have feedback loops with cross-reactions.
Contact Activation
Factor XII, high-molecular-weight kininogen (HMWK), prekallikrein (PK), and factor XI form the contact or surface activation group. The in vivo events that activate factor XII remain unconfirmed. Clinicians do know that ex vivo contact with ionically charged surfaces will activate factor XII. Because factor XII autoactivates by undergoing a shape change in the presence of a negative charge, in vitro coagulation tests use glass, silica, kaolin, and other compounds with negative surface charge (see Chapter 17). The glycocalyx of endothelial cells has a repelling charge for coagulation proteins. One potential in vivo mechanism for factor XII activation is disruption of the endothelial cell layer, which exposes the underlying negatively charged collagen matrix. Activated platelets also provide negative charges on their membrane surfaces. HMWK anchors the other surface activation molecules, PK and factor XI, to damaged endothelium or activated platelets. Factor XIIa cleaves both factor XI, to form factor XIa, and PK, to form kallikrein. Figure 31-3 depicts the events of surface activation.
Intrinsic System
Intrinsic activation forms factor XIa from the products of surface activation. Factor XIa splits factor IX to form factor IXa, with Ca2+ required for this process. Then factor IXa activates factor X with help from Ca2+, a phospholipid surface (platelet-phosphatidylserine), and a GP cofactor, factor VIIIa. Figure 31-4 displays a stylized version of factor X activation. The phospholipids and GP cofactors are on the surface of platelets.
Extrinsic System
Activation of factor X can proceed independently of factor XII by substances classically thought to be extrinsic to the vasculature. This is of historic interest because today it is known that the expression of tissue factor is actually a highly regulated event in endothelial cells. Any number of endothelial cell insults can lead to the production of tissue factor by the endothelial cell.3–10 At rest, the endothelial cell is quite antithrombotic. However, with ischemia, reperfusion, sepsis, or cytokines (particularly interleukin-6), the endothelial cell will stimulate its production of intracellular nuclear factor-κB and send messages for the production of messenger RNA for tissue factor production.6 This can happen quickly and the resting endothelial cell can turn out large amounts of tissue factor. It is widely held today that the activation of tissue factor is what drives many of the abnormalities of coagulation after cardiac surgery, rather than contact activation.7,8 In some tissues, cells outside the vasculature contain large amounts of tissue factor. They are released when cells are damaged/ruptured. Thromboplastin, also known as tissue factor, released from tissues into the vasculature, acts as a cofactor for initial activation of factor X by factor VII. Factors VII and X then activate one another with the help of platelet phospholipid and Ca2+, thus rapidly generating factor Xa. (Factor VIIa also activates factor IX, thus linking the extrinsic and intrinsic paths.)
Thromboplastin straddles the extravascular cell membrane, with its extracellular portion available to bind factor VIIa. Cytokines (particularly tumor necrosis factor-α and interleukin-6) and endotoxins can stimulate its expression on endothelium.9,10 It anchors factor VIIa to the cell surface, thus facilitating activation of factor X. The amount of available factor Va also seems to be quite important for the adequate functioning of the normal coagulation cascades.
Common Pathway
Figure 31-5 depicts the steps involved in formation of thrombin from its precursor, prothrombin. The by-product, fragment F1.2, serves as a plasma marker of prothrombin activation. An alternative scheme generates a different species, meizothrombin, involved more specifically in activation of coagulation inhibitors.11
Thrombin cleaves the fibrinogen molecule to form soluble fibrin monomer and polypeptide fragments termed fibrinopeptides A and B. Fibrin monomers associate to form a soluble fibrin matrix. Factor XIII, activated by thrombin, cross-links these fibrin strands to form an insoluble clot. Patients with lower levels of factor XIII have been found to have more bleeding after cardiac surgery.11,12
Modulators of the Coagulation Pathway
Thrombin, the most important coagulation modulator, exerts a pervasive influence throughout the coagulation factor pathways. It activates factors V, VIII, and XIII; cleaves fibrinogen to fibrin; stimulates platelet recruitment, chemotaxis of leukocytes and monocytes; releases t-PA, prostacyclin, and nitric oxide from endothelial cells; releases interleukin-1 from macrophages; and with thrombomodulin, activates protein C, a substance that then inactivates factors Va and VIIIa.12 Note the negative feedback aspect of this last action (Figure 31-6). Coagulation function truly centers around the effects of thrombin. The platelets, tissue factor, and contact activation all are interactive and are activated by a rent in the surface of the endothelium or through the loss of endothelial coagulation control. Platelets adhere to a site of injury and, in turn, are activated, leading to sequestration of other platelets. It is the interaction of all of those factors together that eventually creates a critical mass. Once enough platelets are interacting together, with their attached surface concomitant serine protease reactions, then a thrombin burst is created. Only when enough thrombin activation has been encountered in a critical time point is a threshold exceeded, and the reactions become massive and much larger than the sum of the whole. It is thought that the concentration and ability of platelets to react fully affect the ability to have a critical thrombin burst. CPB may affect the ability to get that full thrombin burst because of its effects on platelet number, platelet-to-platelet interactions, and the decreased amounts of protein substrates.
The many serine proteases that compose the coagulation pathways are balanced by serine protease inhibitors, termed serpins.13 This biologic yin and yang leads to an excellent buffering capacity. It is only when the platelet-driven thrombin burst so overwhelms the body’s localized anticoagulation or inhibitors that clot proceeds forward. Serpins include α1-antitrypsin, α2-macroglobulin, heparin cofactor II, α2-antiplasmin, antithrombin (AT; also termed antithrombin III [AT III]), and others.
An important note is that activated AT III is active only against free thrombin (fibrin-bound thrombin cannot be seen by AT III).14 Prothrombin circulates in the plasma but is not affected by heparin-AT III complexes; it is only thrombin, and thrombin does not circulate freely. Most thrombin in its active form is either bound to GP binding sites of platelets or in fibrin matrices. When blood is put into a test tube and clot begins to form (such as in an activated coagulation time [ACT]), 96% of thrombin production is yet to come. Most thrombin generation is on the surface of platelets and on clot-held fibrinogen. Platelets, through their GP binding sites and phospholipid folds, protect activated thrombin from attack by AT III. Therefore, the biologic role of AT III is to create an anticoagulant surface on endothelial cells. It is not present biologically to sit and wait for a dose of heparin before CPB.
CPB dilutes AT III substantially, and the further consumption of AT III during CPB (thrombin generation) leads, in some patients, to profoundly low levels of this important inhibitor.15 Research work adding AT III back to the CPB circuit has shown promise in that by doing so there is better preservation of serine protease proteins and platelets. Unfortunately, no pharmaceutical company has decided to pursue this line of research to the point that it would be either commonplace or economically feasible to add large amounts of AT III to CPB and, therefore, avoid consumptive coagulopathies. Congenital AT III deficiency can lead to in utero fetal destruction if the fetus is homozygous for the abnormal AT III. However, patients who are heterozygous for AT III abnormalities have about 40% to 60% of normal AT III activity. They have a particularly high risk for deep vein thrombosis. Low AT III levels have been described during extracorporeal membrane oxygenation, and the addition of AT III to the extracorporeal membrane oxygenation circuit has been effective in improving outcome and decreasing bleeding in some circumstances.16 It is not known how useful it would be in all patients on CPB because only small trials have been performed, but these have been encouraging.17,18 Both human AT III concentrate (harvested from multiple plasma donors and pasteurized) and a pharmaceutically engineered, goat milk–produced AT III (slightly different structure than human AT III) are commercially available.
Heparin cofactor II also inhibits thrombin, once it is activated. Although large doses of heparin activate heparin cofactor II, dermatans on endothelial cell surfaces activate it far more effectively, suggesting dermatans as alternative drugs to heparin.19 Dermatan sulfates are not available for use today in the United States.
Another serpin, protein C, degrades factors Va and VIIIa. Like other vitamin K–dependent factors, it requires Ca2+ to bind to phospholipid. Its cofactor, termed protein S, also exhibits vitamin K dependence. Genetic variants of protein C are less active and lead to increased risk for deep vein thrombosis and pulmonary embolism. When endothelial cells release thrombomodulin, thrombin then accelerates by 20,000-fold its activation of protein C20 (see Figure 31-6). Activated protein C also promotes fibrinolysis through a feedback loop to the endothelial cells to release t-PA.21
Regulation of the extrinsic limb of the coagulation pathway occurs via tissue factor pathway inhibitor (TFPI), a glycosylated protein that associates with lipoproteins in plasma.22 TFPI is not a serpin. It impairs the catalytic properties of the factor VIIa-tissue factor complex on factor X activation. Both vascular endothelium and platelets appear to produce TFPI.23,24 Heparin releases TFPI from endothelium, increasing TFPI plasma concentrations by as much as sixfold, which should be viewed as a biologic indicator of how poor heparin is as an anticoagulant. TFPI is not tested for in routine coagulation testing. It may be that some individuals with certain types of TFPI or who have very large amounts of circulating TFPI could be at risk for severe adverse bleeding after cardiac surgery.25–30 This area is just beginning to be examined today both in terms of whether TFPI is responsible for abnormal bleeding and whether its genetic variants have abnormal bleeding or thrombosis.
von Willebrand factor (vWF), a massive molecule composed of disulfide-linked glycosylated peptides, associates with factor VIII in plasma, protecting it from proteolytic enzymes. It circulates in the plasma in its coiled inactive form.31 Disruption of the endothelium either allows for binding of vWF from the plasma or allows for expression of vWF from tissue and from endothelial cells. Once bound, vWF uncoils to its full length and exposes a hitherto cryptic domain in the molecule. This A-1 domain has a very high affinity for platelet GPs. Initially, vWF attaches to the glycoprotein Iα (GPIα) platelet receptor, which slows the platelet forward movements against the shear forces of blood flow. Shear forces are activators of platelets. As the platelet’s forward movement along the endothelial brush border is slowed (because of vWF attachment), shear forces actually increase; thus, the binding of vWF to GP1 acts to provide a feedback loop for individual platelets, further activating them. The activation of vWF and its attachment to the platelet are not enough to bind the platelet to the endothelium, but it creates a membrane signal that allows for early shape change and expression of other GPs, GPIb and GPIIb/IIIa. Then, secondary GPIb binding connects to other vWF nearby, binding the platelet and beginning the activation sequence. It bridges normal platelets to damaged subendothelium by attaching to the GPIb platelet receptor. An ensuing platelet shape change then releases thromboxane, β-thromboglobulin, and serotonin, and exposes GPIIb/IIIa, which binds fibrinogen.
Deficiency States
Decreased amounts of coagulation proteins may be inherited or acquired. Deficiencies of each part of the coagulation pathway are considered in turn. Table 31-1 summarizes the coagulation factors, their activation sequences, and vehicles for factor replacement when deficient.
TABLE 31-1 The Coagulation Pathway Proteins, Minimal Amounts Needed for Surgery, and Replacement Sources
Contact Activation
Although decreased amounts of factor XII, PK, and HMWK can occur, these defects do not have clinical sequelae. The autosomal dominant deficiency of factor XI is very rare. However, its incidence among Ashkenazi Jews is as high as 0.1% to 0.3%.32 Most of these patients require factor replacement with fresh-frozen plasma (FFP) for surgery. Spontaneous bleeding does not occur, but increased bleeding after a surgical event or trauma is possible. Factor XI concentrations do not directly correlate with bleeding after trauma or surgery, suggesting that factor XI deficiency can be easily overcome by activation of platelets, factor IX, and other signaling mechanisms. An FFP dose of 10 mL/kg will yield target concentrations of 20% activity, and it is often given for this rare deficiency.
Intrinsic
Hemophilia occurs worldwide, with a prevalence of 1 in 10,000. Hemophilia A, which constitutes about 80% to 85% of cases, originates from decreased activity of factor VIII. Because platelet function remains normal, minor cuts and abrasions do not bleed excessively. Joint and muscle hemorrhages ensue from minor trauma or, seemingly, spontaneously. Airway issues include epistaxis and obstruction from bleeding into the tongue. The bleeding time and prothrombin time (PT) remain normal, whereas the activated partial thromboplastin time (aPTT) is prolonged.33 Desmopressin, a synthetic analog of vasopressin, will increase factor VIII activity by releasing vWF from endothelial cells, except in patients with severe hemophilia A who have too little functional factor VIII available for vWF.34 Major surgery requires replenishment of factor VIII functional activity to greater than 80% of normal with FFP, cryoprecipitate, or factor VIII concentrate.35 Factor VIII concentrate is the preferred method today. After surgery, factor VIII concentrations should be maintained greater than 30% for 2 weeks with repeat doses. Current plasma-derived concentrates are solvent detergent and heat-treated to remove lipid-coated viruses (human immunodeficiency virus [HIV], hepatitis B, human T-lymphotropic virus [HTLV]. However, this was not historically the case, and in Europe during the early parts of the HIV/acquired immune deficiency syndrome (AIDS) crisis, most individuals with hemophilia contracted HIV/AIDS from contaminated products. A recombinant product also is available but costs about three times that of the plasma-derived one.
Factor IX deficiency manifests as hemophilia B, constituting 15% to 20% of all hemophilia cases. Patients present with symptoms identical to those with hemophilia A. No study has demonstrated a salutary effect of desmopressin here. Prothrombin complex (factor IX) concentrates will replenish levels, but consumptive coagulopathy remains a possible complication, stemming from the presence of activated coagulation factors, principally factor VIIa, in the preparation.36 Purified factor IX concentrate, a plasma-derived, solvent detergent and heat-treated product, currently constitutes the replacement vehicle of choice for patients with hemophilia B.37 Recombinant pure factor IX concentrate will be available, but at considerable expense (factor VIIa is now available as well). Consultation with an experienced hematologist aids in the care of patients with hemophilia undergoing surgery.
Common Pathway
Deficiency of either factor V or factor X, both extremely rare autosomal recessive disorders, increases both the PT and PTT. The bleeding time is normal in factor X deficiency but prolonged in one third of patients with factor V deficiency. The bleeding time prolongation arises from the role of factor V in platelet function.38 Prothrombin complex concentrate or FFP supplies prothrombin, factor V, and factor X. Numerous inherited abnormalities (polymorphisms) of prothrombin and fibrinogen occur, with varying characteristics. Cryoprecipitate, which contains 250 mg fibrinogen and 100 units factor VIII per 10-mL bag, as well as vWF and factor XIII, treats inherited or acquired disorders of fibrinogen. Many of these polymorphisms are associated with hypercoagulability and, perhaps, accelerated atherosclerosis rather than bleeding.
Warfarin
Administration of this vitamin K antagonist affects plasma levels of factors II, VII, IX, and X, as well as proteins C and S.39 Protein C has the shortest half-life, followed by factors VII (6 hours), IX (24 hours), X (2 days), and II (3 days).36 Substantial PT prolongation and some PTT prolongation accompany warfarin therapy. For immediate restoration of clotting function, FFP is given. Otherwise, parenteral vitamin K or cessation of warfarin (Coumadin) suffices. Clinicians should be extremely careful in administering these compounds if a patient is suspected of having heparin-induced thrombocytopenia (HIT). Treatment with commercially available factor VIIa restores PT to normal and appears to stop bleeding when warfarin therapy has not had time to be reversed. The use of factor VIIa for this intervention before surgery is both effective and, perhaps, worthwhile as it avoids the use of FFP. The time scale of factor VIIa effectiveness may not be as long as if one normalized circulating levels through FFP administration. It makes sense, therefore, that if the PT is prolonged again approximately 8 to 12 hours after a dose of factor VIIa, then redosage of the drug be given.
Inherited Thrombotic Disorders
A number of genetic abnormalities lead to thrombosis. The most prevalent (2% to 5%) in European-derived populations is factor V Leiden, in which a point mutation at residue 1691 on factor V renders it resistant to inactivation by activated protein C.40 Venous thromboembolism risk increases 7-fold in heterozygotes and 80-fold in homozygotes, but episodes are less severe than in other thrombotic disorders. Pregnancy and oral contraceptives greatly exacerbate the thrombotic tendency.41
Protein C or S deficiencies, if homozygous, present at birth as neonatal purpura fulminans. Protein C deficiency heterozygotes demonstrate 40% to 60% protein C activity and present with venous thrombosis beginning in adolescence. The role of reduced concentrations of protein S in causing thrombosis has come into question.41 Together, deficiencies of AT, protein C, and protein S account for 10% to 15% of inherited thrombosis.42
Homocysteinemia is the mild heterozygous state of cystathione β-synthetase deficiency, known as homocystinuria in its more serious homozygous form. Increased plasma concentrations of homocysteine induce endothelial cell tissue factor activity, stimulate factor V activation, and impair protein C activation, all of which contribute to thrombosis. Folic acid and vitamins B6 and B12 reduce homocysteine plasma concentrations.43

Platelet Function
Most clinicians think first of the coagulation proteins when considering hemostasis. Although no one element of the many that participate in hemostasis assumes dominance, platelets may be the most complex.44 Without platelets, there is no coagulation and no hemostasis, so it could be argued that they are most important. Without the proteins, there is hemostasis, but it lasts only about 10 to 15 minutes as the platelet plug is inherently unstable and breaks apart under the shear stress of the vasculature. Platelets provide phospholipid for coagulation factor reactions; contain their own microskeletal system and release coagulation factors; secrete active substances affecting themselves, other platelets, the endothelium, and other coagulation factors; and alter shape (through active actin-myosin contraction) to expose membrane GPs essential to hemostasis. Their cell signaling is highly regulated, is present in other cell lines (RBCs, leukocytes, and endothelial cells), and has been intensively studied. Platelets have perhaps as many as 30 to 50 different types of cell receptors, with many ways of these being activated and inhibited.
The initial response to vascular injury is formation of a platelet plug. Good hemostatic response depends on proper functioning of platelet adhesion, activation, and aggregation (Figure 31-7). This section first discusses these aspects and then follows with the effects of platelet disorders and platelet-inhibiting pharmaceuticals. Clinicians talk about platelet dysfunction, which is largely overarching and grossly too general a term. The complexity that is platelet function really needs careful study.
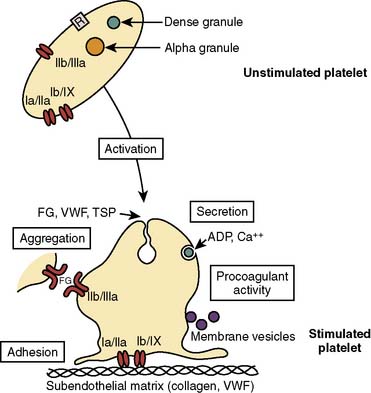
Figure 31-7 Platelet function in hemostasis.
(From George J, Shattil SJ: The clinical importance of acquired abnormalities of platelet function. N Engl J Med 324:27, 1991.)
Platelet Adhesion
Capillary blood exhibits laminar flow, which maximizes the likelihood of interaction of platelets with the vessel wall. Red cells and white cells stream near the center of the vessels and marginate platelets. However, turbulence causes reactions in endothelium that lead to the secretion of vWF, adhesive molecules, and tissue factor. Shear stress is high as fast-moving platelets interact with the endothelium. When the vascular endothelium becomes denuded or injured, the platelet has the opportunity to contact vWF, which is bound to the exposed collagen of the subendothelium. A platelet membrane component, GPIb, attaches to vWF, thus anchoring the platelet to the vessel wall. Independently, platelet membrane GPIa and GPIIa and IX may attach directly to exposed collagen, furthering the adhesion stage.44–46
After activation (see later), additional adhesive mechanisms come into play. Release of selectin GPs from α-granules allows their membrane expression, thus promoting platelet-leukocyte adhesion. This interaction ultimately may allow expression of tissue factor on monocytes, thus amplifying coagulation.47
The integrin GPs form diverse types of membrane receptors from combinations of 20 α and 8 β subunits.48 One such combination is GPIIb/IIIa, a platelet membrane component that initially participates in platelet adhesion. Platelet activation causes a conformational change in GPIIb/IIIa, which results in its aggregator activity.
Platelet adhesion begins rapidly—within 1 minute of endothelial injury—and completely covers exposed subendothelium within 20 minutes.45 It begins with decreased platelet velocity when GPIb/IX and vWF mediate adhesion, followed by platelet activation, GPIIb/IIIa conformational change, then vWF binding and platelet arrest on the endothelium at these vWF ligand sites.44,46
Platelet Activation and Aggregation
Agonists induce a graded platelet shape change (the amount based on the relative amount of stimulation), increase platelet intracellular Ca2+ concentration, and stimulate platelet G protein. In addition, serotonin and thromboxane A2 are potent vasoconstrictors (particularly in the pulmonary vasculature).49 The presence of sufficient agonist material results in platelet aggregation. Aggregation occurs when the integrin proteins (mostly fibrinogen) released from α granules form molecular bridges between the GPIIb/IIIa receptors of adjacent platelets (the final common platelet pathway).
Platelet Disorders
Dysfunctional vWF produces von Willebrand disease (vWD), an autosomal dominant disorder of variable expressivity.34 With an incidence of 1.4 to 5 cases per 1000 population, vWD is the most common inherited coagulopathy. Patients present with mucocutaneous hemorrhages rather than hemarthroses. Common symptoms include epistaxis, ecchymoses, and excessive bleeding after trauma, with surgery, or during menses. Because vWF concentrations vary greatly with time, symptoms have variable expressivity. Desmopressin reverses the prolonged bleeding time in patients with mild vWD.50 As with hemophilia A, severe cases of vWD do not benefit from desmopressin therapy. In one rare class of vWD (type IIB, 3% to 5% of vWD), desmopressin aggregates platelets, inducing thrombocytopenia and worsening rather than helping hemostasis. Table 31-2 summarizes features of the more common types of vWD. When blood products are needed, cryoprecipitate constitutes the replacement vehicle of choice in vWD, although recent factor VIII concentrates retain vWF activity and have been used successfully during cardiac surgery.51
The addition of agonist (ADP or collagen) to platelets allows measurement of platelet aggregation in vitro. In Glanzmann thrombasthenia, the GPIIb/IIIa receptor is absent, preventing aggregation. However, ristocetin, a cationic antibiotic similar to vancomycin, can agglutinate platelets directly via GPIb receptors and vWF. Absence of the GPIb receptor, Bernard–Soulier syndrome, prevents adhesion and agglutination with ristocetin, but aggregation to ADP is normal, because the GPIIb/IIIa receptor is intact. Patients with vWD also exhibit impaired platelet adhesion and normal aggregation. Decreased amounts of vWF antigen distinguish it from the Bernard–Soulier syndrome. In platelet storage pool deficiency, impairment of dense granule secretion yields no ADP on adhesion. In vitro addition of collagen will not aggregate platelets because of absence of ADP release, whereas added ADP will initiate some aggregation. Table 31-3 summarizes these diagnostic findings. Uremia impairs the secretory and aggregating functions of platelets, resulting in an increased bleeding time. However, the most common effect of renal dysfunction is hypercoagulability. It is only with severe uremia that the platelets are poisoned. It is, therefore, a common misconception in the operating room that a patient with mild-to-moderate renal failure will be at increased risk for bleeding. The utilization of thromboelastography (TEG) can help in deciding whether the extent of renal failure is causing hypocoagulability. The cause and clinical significance remain poorly defined (see Chapter 17).