CHAPTER 319 Thoracolumbar Trauma
The incidence of thoracolumbar spine injuries after blunt trauma is approximately 2% to 7.5%.1–4 Nineteen percent to 50% of these injuries have associated neurological injury.5–7 Even in the absence of neurological deficits, injuries to the thoracolumbar spine can be associated with long-term pain and disability.8,9
Thoracolumbar fractures are the result of high-velocity deceleration mechanisms and occur most commonly in association with falls or motor vehicle accidents.1,10,11 The thoracolumbar spine is particularly vulnerable to injury in falls from a height greater than 3 m. Spinal and lower extremity fractures are the most common injuries after a fall from a height.12
Anatomy
The anatomy and biomechanical properties of the thoracolumbar spine dictate the patterns of injury that occur commonly. The transition between the more rigid thoracic spine and the mobile lumbar spine concentrates bending and axial loads at the thoracolumbar junction and thus explains the higher prevalence of injury at the T11-L1 level than at more proximal aspects of the thoracic spine or distal lumbar levels.13 Unfortunately, in adults the part of the spinal cord that ends near the mechanically vulnerable thoracolumbar junction contains the primary efferents for all the lumbosacral roots, and hence canal encroachment can have significant neurological consequences.
The anatomy of the rib cage significantly influences the biomechanical properties of the thoracolumbar spine. Above T10, ribs 3 through 8 articulate anteriorly with the sternum and posteriorly with their associated vertebral body and transverse process, as well as with the vertebral body above via an inferior demifacet. This configuration stabilizes the thoracic spine and increases its rigidity twofold to threefold.14 Below this level, the rigidity of the thoracic spine is reduced as the anatomy evolves into the configuration of the more mobile lumbar spine.
The recently proposed Thoracolumbar Injury Classification and Severity Score (TLICS) has emphasized the integrity of the posterior ligamentous complex (PLC) in determining stability and dictating treatment of thoracolumbar injuries.15 The PLC is composed of several individually named ligamentous structures, including (anterior to posterior) the facet joint capsules, ligamentum flavum (LF), interspinous ligament (ISL), and supraspinous ligament (SSL).
The LF in the thoracolumbar region has two layers, superficial and deep.16 The deep layer runs vertical and resists kyphotic deformation of the spine. The superficial layer contains a “zone of separation” between the right and left fibers, whereas the deep layer contains a prominent midline “cleavage separation.” Elastic fibers make up 80% of the LF and give it its characteristic yellow color. In the neutral position, the LF is actually in a stretched state. With aging, the elastic component is replaced with collagen and chondrocytes and at times may become ossified. The degenerative changes in the LF result in a decreased ability to resist flexion. The increased mass of the thickened LF can also contribute to neural compression in trauma.
The ISL becomes more developed as one moves caudally down the thoracolumbar spine. The ISL can be divided into anterior, middle, and posterior portions. The anterior portion is separated into a pair of ligaments with a thin sheet of adipose tissue between them. Each side is continuous with the corresponding LF anteriorly. The posterior portion is fused into a single ligament and blends with the SSL posteriorly. The middle portion makes up the majority of the ligament. The fibers actually run from anteroinferior to posterosuperior. This orientation does not limit flexion, but complex interactions between collagen fibers increase the ligament’s stiffness in flexion.17 In a cadaveric model, the ISL and SSL are the first to sprain during hyperflexion.18
The SSL, which runs along the entire length of the spine, becomes strongest in the lumbar spine. Some controversy exists whether it is a true ligament or whether it represents the strong tendinous fibers of the deep erector spinae and crossing fibers of the thoracolumbar fascia.19 Regardless, because of its orientation and distance from the axis of rotation, it is particularly effective in resisting flexion movements.
Thoracolumbar spinal fractures place the conus medullaris and cauda equina at risk, and injury to these neurological structures has profound functional consequences. The conus medullaris and cauda equina mark the transition between the central and peripheral nervous systems. Injury results in a constellation of upper and lower motoneuron symptoms, depending on the exact site of the lesion. The location of the conus medullaris varies by developmental stage. At birth, the cord fills the vertebral canal and terminates at the lumbosacral junction.20 The distal tip of the spinal cord then ascends with infant development, probably because of the differential growth between the spinal column and spinal cord. In adults, the tip usually terminates at the midaspect of the first lumbar vertebra. However, its position varies between the lower 11th thoracic and upper 3rd lumbar vertebrae.21 Traditionally, it has been taught that the termination also varies with the position of the spine, although a recent study by Bauer and colleagues found no change in the position of the conus with flexion.22 However, they did demonstrate that the cauda equina displaces medially over the conus with flexion.
The origin of the artery is normally a direct or indirect branch of the left lower intercostal or upper lumbar arteries, between the 10th and 12th thoracic vertebrae (Fig. 319-1). Lu and coauthors reported in a cadaver study of 15 individuals that the artery was present on the left side as the primary radiculomedullary supply to the spinal cord in 80% of specimens.23 In the remaining 20% they found supplemental lumbar feeders lower down at L1 or L2 that anastomosed with the descending division. The vessel originated off the intercostal artery near the costotransverse joint before entering the intervertebral foramen on its intradural course. It is at risk for iatrogenic injury during approaches requiring disarticulation of the rib or sacrifice of the nerve root (e.g., in posterolateral approaches to the anterior thoracic spine).
Initial Assessment and Management
Clinical Evaluation
The secondary survey includes a complete neurological examination. This is best completed in the standardized format developed by the American Spinal Injury Association (ASIA).24 Certain myotomes are not tested with the standard ASIA form and should be noted specifically in patients with thoracolumbar trauma. The S2 myotome is documented by assessing the ability to flex the toes. Superficial cutaneous reflexes (abdominal cutaneous reflex and the cremasteric reflex) should also be documented.
As indicated earlier, at the thoracolumbar junction the spinal cord is ending and the cauda equina is emerging. Hence, injuries at this level can cause a constellation of neurological signs and symptoms as a result of varying degrees of spinal cord or peripheral nerve damage (Fig. 319-E1). Distinguishing the two in an individual with a T12 or L1 burst fracture can be extremely difficult, although conceptually, constituents of the peripheral nervous system have a greater chance of recovery after injury than do constituents of the central nervous system. Injuries isolated to the very tip of the conus medullaris where the sacral nerve roots emerge may cause profound deficits in bowel/bladder and sexual function with almost paradoxically normal lower extremity function. More typical, however, are mixed patterns of injury, given that the anatomy of the region makes it difficult to damage the distal aspect of the spinal cord without also damaging some of the roots of the cauda equina that are emerging at the same level. Fractures distal to L1 will affect the roots of the cauda equina more predominantly, depending obviously on how low the spinal cord extends in a given individual.
As in the cervical spine, the greatest danger in the assessment and early management of thoracolumbar injuries is missing the diagnosis. Delayed diagnosis occurs in almost 5% of thoracolumbar injuries, and such delays have been shown to increase the incidence of neurological deficits.8 Early recognition and prompt appropriate treatment are imperative to improve patient outcomes.
In a more recent study designed to develop imaging guidelines for the thoracolumbar spine in blunt trauma patients, Hsu and coworkers retrospectively applied a diagnostic pathway protocol based on a review of the literature.10 The majority of fractures in the study occurred at the thoracolumbar junction (62%) and were caused either by falls (49%) or by motor vehicle accidents (26%). The most common clinical sign of injury was the presence of back pain or midline tenderness (88%). Seven percent of patients had no detectable clinical signs. The clinical findings of back pain and midline tenderness are often difficult to ascertain in patients who are intoxicated, in those with a lowered Glasgow Coma Scale score, or in patients who have major distracting injuries.
Finally, a comment should be made regarding spinal immobilization and the use of spine boards. Spinal immobilization is used early during transportation to prevent neurological injury as a result of displacement of unstable injuries. Spine boards, in contrast, should act only as a transportation device and not as a means of providing ongoing immobilization once the patient has been evaluated. This distinction is sometimes lost on health care staff, who often view the spine board as a therapeutic measure for patients with thoracolumbar injuries, thereby resulting in prolonged periods of immobilization on a hard, flat surface. This has resulted in reported rates of pressure ulcers in trauma patients as high as 31%.25
Immobilization and spine boards are a known risk factor for pressure necrosis.26 In a study by Lerner and Moscati, the average time that a trauma patient spent on a spine board was approximately 1 hour.27 If patients required radiologic investigation before removal of the spine board, the total time reached 3 hours. Replacing the spine board with a supportive mattress is a simple maneuver that can prevent significant morbidity and mortality. It is important to remember that patients should be removed from the spine board as soon as possible after a qualified physician has assessed them.
Imaging
Imaging studies are ultimately required to make the diagnosis of thoracolumbar injuries and to guide management. To date, no standardized imaging algorithm for assessment of spine trauma has been established, although many institutions have developed their own protocols in an attempt to minimize the incidence of “missed injuries.” The most common cause of a missed thoracolumbar injury is inadequate imaging, which occurs not infrequently in the setting of a patient with urgent, life-threatening injuries.1,11
Despite variations in approach, some basic principles do exist for imaging patients with a thoracolumbar spine injury. Traditionally, imaging usually commenced with screening radiographs of the entire spine. More recently, however, the use of multidetector computed tomography (CT) in many emergency departments has begun to supplant conventional radiographic imaging. Plain radiographs continue to be useful, however, for identifying the level of injury, characterizing bony injuries (e.g., loss of height of the anterior vertebral body), assessing the relationship of one vertebral body to another, and evaluating the overall alignment of the spine.28
Review of the radiographic imaging requires a systematic approach to avoid overlooking injuries. Alignment should be assessed in the lateral and frontal planes by checking the anterior and posterior vertebral body lines, the spinolaminar line, the articular pillar and facet joints, the interpedicular and interspinous distances, and the position of the transverse processes. Translation of one vertebral body on the next should be evident in this assessment and probably reflects significant instability.29 Angulation across an injury site can be measured with the Cobb method, which involves measuring from the superior end plate one level above the fracture to the inferior end plate one level below the fracture (Fig. 319-2).30 On anteroposterior (AP) radiographs, widening of the interpedicular distance relative to other levels suggests failure of the posterior vertebral body (middle column), as seen in burst fractures. The interspinous distances can sometimes also be appreciated, and widening is suggestive of a flexion-distraction injury.
Radiographic signs that may indicate major ligamentous (including PLC) disruption and instability include displacement of bone, widening of the interlaminar space, widening of the apophyseal joints, widening of the vertebral canal, and disruption of the posterior vertebral body.31 Daffner and colleagues retrospectively reviewed 138 spinal injuries in 125 patients and noted an association of five radiographic findings with specific anatomic injuries (skeletal, ligamentous, and articular).32 They concluded that “the presence of only one is sufficient to establish a diagnosis of instability.” In an earlier cadaveric study, Nagel and associates were able to demonstrate, in the absence of a vertebral fracture, that kyphosis of 20 degrees or lateral angulation of 10 degrees or greater requires that the PLC and posterior anulus must be disrupted.33
Unfortunately, radiographs of the spine are often inadequate, especially in an obese patient. With advances in CT, plain radiography is taking on a more limited role. Multidetector CT allows excellent bone detail, and multiplanar reformations permit visualization in any plane. Three-dimensional constructions add a spatial appreciation of the fracture pattern. CT also adds the benefit of visceral imaging. Abdominal and pelvic injury is common in patients with thoracolumbar injuries.34
Once a spine injury is identified, it is prudent to obtain a CT scan or further radiographs of the entire spine to rule out other noncontiguous fractures. Approximately 4.2% to 23.8% of patients have noncontiguous fractures on plain radiographic studies.35 These most commonly occur at the cervicothoracic and lumbosacral junctions. In a prospective study of all spine trauma patients admitted over a 3-year period, Green and Saifuddin documented a 34% incidence of noncontiguous injuries with magnetic resonance imaging (MRI) (21% for noncontiguous wedge compression fractures and 13% for noncontiguous burst fractures)36 (Fig. 319-3).
MRI in patients with thoracolumbar trauma is complementary to CT scanning. The obvious benefit is the ability to visualize the diskoligamentous soft tissues and neurological structures. The anatomy of the neural elements (spinal cord, conus medullaris, cauda equina, and nerve roots) is important to visualize in patients with neurological deficits. Epidural hematomas and their relationship to the spinal cord can be appreciated. The great medullary artery can be detected in the majority of patients with contrast-enhanced magnetic resonance angiography.37
Because the diskoligamentous structures (and particularly the PLC, which consists of the SSL, ISL, LF, and facet joint capsules) play such an important role in determining the stability of the injury, assessment of these soft tissue structures with MRI can be extremely helpful in guiding management decisions. In fact, there is a general consensus among leading spine surgeons that disruption of the PLC makes a thoracolumbar spinal injury much more unstable and warrants strong consideration for operative intervention.29
Although plain radiography and CT rely on relative displacement or angulation of adjacent vertebral segments as indirect signs of PLC disruption, MRI may be able to directly detect disruption of the PLC.35,38,39 The sensitivity and specificity of MRI in predicting a torn PLC are 90% and 100%, respectively.39 What defines PCL disruption and which imaging sequence is used to do so vary between studies. Discontinuity of the “black stripe” on T2-weighted MRI, which represents the LF, posterior longitudinal ligament, or SSL, is often used. Tears of the facet capsule and the ISL result in accumulation of fluid and is represented by high-intensity signal on T2-weighted imaging. The high-intensity changes related to acute edema can be difficult to distinguish from fat, which has signal intensity similar to that of edema on standard T2-weighted imaging. T2-weighted fat suppression protocols remove the surrounding “noise” caused by fat and improve the ability to discern acute injuries (Fig. 319-4). In an important study, Lee and associates documented the clinical, radiographic, and MRI findings in patients with thoracolumbar injuries to assess their reliability in accurately predicting injury to the PLC, which was subsequently evaluated intraoperatively40 (Table 319-E1). The accuracy of palpation, plain radiography, and MRI for correctly predicting disruption of the PLC (SSL/ISL/LF) was 53.6%, 66.7%, and 90.9%/97%/87.9%, respectively. It should be noted that palpation and plain radiography had excellent positive predictive values (92.9% and 95.2%, respectively), which suggests that these tests are actually quite good in identifying injuries but that MRI may have a stronger role when clinical examination or plain radiology is negative or indeterminate. Unfortunately, standardized criteria for defining PLC integrity do not currently exist, and accordingly, even experienced surgeons demonstrate only fair agreement when assessing MRI studies to determine the integrity of the PLC.15,29 Further refinements in MRI protocols and criteria for establishing the integrity of the PLC are needed.
Despite the increased use of MRI in patients with thoracolumbar trauma, many spine surgeons believe that plain AP and lateral radiographs are the most important factors in defining PLC integrity.41 This is consistent with Daffner and colleagues’ work on radiographic signs.32 Members of the Spine Trauma Study Group (STSG) were surveyed to identify imaging parameters that they thought were most suggestive of PLC disruption in the thoracolumbar spine. “Vertebral body translation” on plain radiographs was ranked the most important indicator of PLC disruption by 50% of respondents, with “interspinous spacing greater than the level above or below on AP plain X-ray” and “diastasis of the facet joints on CT” being ranked second and third, respectively. Surprisingly, MRI indicators of PLC disruption ranked lower. In essence, this simply reflects the fact that when gross malalignment of the spine exists, there is little doubt about the integrity of the PLC.
Classification
Fracture classification systems attempt to produce a universal nomenclature to facilitate communication between health care members, guide patient management, and allow consistent stratification for ongoing research. Classification categories should be clinically relevant entities that surgeons use for diagnosis with sufficient confidence to limit incorrect categorization and associated treatment errors. No classification system of thoracolumbar injuries has been universally accepted to date. Historically, thoracolumbar spine classification systems have been scrutinized for their reliability, reproducibility, or clinical validity.42–44 As a result, classification systems for thoracolumbar trauma continue to evolve.
The first generation of thoracolumbar fracture classification systems focused on describing common fracture patterns. Bohler in 1930 published the first thoracolumbar classification system.45 He used fracture pattern and mechanism of injury to distinguish five fracture subtypes: compression, flexion-distraction, extension, shear, and rotational injuries. He did not attempt to classify injuries as stable or unstable based on fracture pattern. In 1943, Watson-Jones published a book in which he described the concept of instability in spinal fractures.15a Nicholl expanded on this work by identifying the instrumental role of the PLC.16
The second generation of thoracolumbar fracture classification systems used architectonic abstractions, most notably the concept of spinal “columns,” in an attempt to predict the relationship of different fracture patterns to immediate and long-term mechanical and neurological instability. Holdsworth developed the two-column concept in which the vertebral body acted as the anterior weight-bearing column, with the stabilizing ligamentous complex being located posteriorly.46 Disruption of the PLC defined instability. Biomechanical studies, however, demonstrated that disruption of the PLC in conjunction with the vertebral body (middle and anterior columns) was required to produce instability.16,47 Holdsworth’s two-column concept did not allow for this.
The third generation of thoracolumbar fracture classification systems is marked by the advent of CT, which dramatically increased the ability to describe fracture morphology in detail. Based on this, Denis48 and McAfee and colleagues49 simultaneously introduced the three-column concept of the spine in 1983. Denis’ retrospective review of 412 thoracolumbar injuries added the middle column to Holdsworth’s two-column model. The anterior column included the anterior longitudinal ligament and the anterior half of the disk and vertebral body. The middle column included the posterior longitudinal ligament and the posterior half of the disk and body. The posterior column included the remaining elements (Fig. 319-5). According to Denis, failure of the middle column was key in defining “instability.” Four fracture patterns were defined on the basis of relative involvement of each column: compression, burst, seat belttype, and fracture-dislocation. These four types were then divided into groups and subgroups, which resulted in 16 total fracture subtypes (Figs. 319-6 to 319-8 and Fig. 319-E2). Burst fractures, based on disruption of the middle column, were classified as being mechanically unstable, but this concept has subsequently generated substantial confusion given that we now recognize that such injuries are usually stable if the PLC is intact.
The Arbeitsgemeinschaft für Osteosynthesefragen (AO) classification was introduced by Magerl and colleagues in 1994.50 It is the most widely used system in European centers. The AO classification is based on mechanistic and morphologic criteria. Within each mechanistic class, further subclassification reflects a graduated scale of progressive injury and instability (Table 319-E2). The classification system is built on a 3-3-3 scheme. The fundamental injury pattern is determined by the mechanism of injury: A, compression; B, distraction; and C, axial torque. Each mechanistic type is further subdivided into three groups based on morphologic severity and then into three subgroups. As result of its complexity, the reliability of the system is compromised.44,51 Wood and associates evaluated the reliability of the AO and Denis classification systems. Thirty-one acute thoracolumbar spine fractures were classified with each system on two occasions 3 months apart by 19 trained spine surgeons. The mean interrater reliability was only moderate for both systems (AO classification, κ = 0.48; Denis classification, κ = 0.54). Intrarater reliability was substantial for the basic level of classification (A, B, and C in the AO classification; compression, burst, seat belt, and fracture-dislocation in the Denis classification). With further subclassification, the reliability was significantly diminished (no κ value given). The authors pointed out the concern over the “tendency for well trained spine surgeons to classify the same fracture differently.”
Type A: Vertebral Body Compression | ||
A1. Impaction fractures | A1.1. End-plate fractures | |
A1.2. Wedge impaction fractures |
From Magerl F, Aebi M, Gertzbein S, et al. A comprehensive classification of thoracic and lumbar injuries. Eur Spine J. 1994;3:184-201.
Moving Forward from Purely Morphologic Classifications: Thoracolumbar Injury Severity Score and Thoracolumbar Injury Classification and Severity Score
The first attempt at establishing such a system was the Thoracolumbar Injury Severity Score (TLISS).52 This is a “point accrual” system in which the higher the points, the more likely the patient is to require surgical intervention. The severity score is based on three major categories: the mechanism of injury as defined by imaging, the integrity of the PLC, and the neurological status of the patient (Table 319-1). A point value (1 to 4) is assigned for each category, which implies a progressive degree of injury and potential instability. Once a total score is assigned based on the major variables, the need for operative intervention can be determined. Patients with scores lower than 4 are considered to have injuries that can be managed nonoperatively. Patients with scores higher than 4 are considered to have injuries that most likely should be treated surgically. Patients with a score of 4 have injuries that may be treated operatively or nonoperatively based on the preference of the surgeon. The system also allowed qualifiers (severe head injury, multisystem polytrauma, excessive kyphosis, and others) to be used to permit the physician to treat on the basis of the unique features of each patient.
DESCRIPTION | QUALIFIER | POINTS |
---|---|---|
1. Injury Mechanism: Worst Level Is Usedand the Injury Is Additive | ||
a. Compression | Simple compression | 1 |
Lateral angulation >15 degrees | 1 | |
Burst | 1 | |
b. Translational/rotational | 3 | |
c. Distraction | 4 | |
2. Posterior Ligamentous Complex Disruptedin Tension, Rotation, or Translation | ||
a. Intact | 0 | |
b. Suspected/indeterminate | 2 | |
c. Injured | 3 | |
3. Neurological Status | ||
a. Nerve root | 2 | |
b. Cord, conus | Incomplete | 3 |
Complete | 2 | |
c. Cauda equina syndrome | 3 |
Total score less than 4, nonoperative management; score of 4, operative or nonoperative management; and score higher than 4, operative management suggested.
Adapted from Vaccaro A, Baron E, Sanfilippo J, et al. Reliability of a novel classification system for thoracolumbar injuries: the Thoracolumbar Injury Severity Score. Spine. 2006;31(11 suppl):S62-S69; discussion S104.
The TLISS classification system was assessed for its reliability and validity in two subsequent studies. In a study by Vaccaro and coworkers, five attending spine surgeons applied the TLISS to 71 acute thoracolumbar spine injuries on two separate occasions.53 Using the score defined by TLISS, surgeons agreed on the appropriateness of surgical or nonsurgical treatment in 96.4% of cases. Interrater reliability was best for neurological status (κ = 0.91). However, κ scores fell dramatically for injury mechanism (κ = 0.35, fair agreement), PLC integrity (κ = 0.35, fair agreement), and total TLISS score (κ = 0.52, moderate agreement). A larger scale study by Harrop and associates had similar findings.15 Again, the agreement on overall treatment recommendation was maintained, but the interrater reliability of individual scores (mechanism of injury and PLC integrity) was marginal. Based on these findings, the STSG modified the classification system. Within the discussion of the paper by Harrop and colleagues, the TLICS was proposed to improve interrater reliability.
The TLICS is calculated by adding the injury morphology, integrity of the PLC, and neurological status scores (Table 319-2). Based on this assigned score and patient factors, treatment decisions can be made. Scores lower than 4 suggest that nonoperative management should be considered. Patients with scores higher than 4 should be considered for operative management. Again, as in the TLISS system, scores of 4 are indeterminate and rely on the treating surgeon’s preference.
TABLE 319-2 Thoracolumbar Injury Classificationand Severity Score
INJURY MORPHOLOGY | QUALIFIER | POINTS |
---|---|---|
a. Compression | Compression fracture | 1 |
Burst fracture | 2 | |
b. Translational/rotational | 3 | |
c. Distraction | 4 | |
Neurological Involvement | ||
a. Intact | 0 | |
b. Nerve root | 2 | |
c. Complete | 2 | |
d. Incomplete | 3 | |
e. Cauda equina syndrome | 3 | |
Posterior Ligamentous Complex Integrity | ||
a. Intact | 0 | |
b. Suspected/indeterminate | 2 | |
c. Definite injury | 3 |
Total score less than 4, nonoperative management; score of 4, operative or nonoperative management; and score higher than 4, operative management suggested.
Adapted from Harrop J, Vaccaro A, Hurlbert R, et al. Intrarater and interrater reliability and validity in the assessment of the mechanism of injury and integrity of the posterior ligamentous complex: a novel injury severity scoring system for thoracolumbar injuries. Invited submission from the Joint Section Meeting on Disorders of the Spine and Peripheral Nerves, March 2005. J Neurosurg Spine. 2006;4:118-122.
Studies evaluating the TLISS have demonstrated marginal reliability for injury mechanism and posterior ligamentous integrity but nearly perfect agreement on neurological status.15,51 Therefore, the injury mechanism category was replaced by injury morphology. This was thought to be more reliable than inferring the mechanism from imaging. In the TLISS system, multiple mechanisms could be summated to give a greater severity score for the injury mechanism category, but this was believed to be a source of confusion for individuals attempting to use the score. In the TLICS system, the morphology of only the most severely injured level is scored. In addition, the subcategory in which 1 point could be assigned for coronal angulation (>15 degrees of angulation) was removed. This eliminates the problem with summating scores at multiple levels and resulting in an inflated score.
In the TLISS system, an intact PLC was assigned points based on the degree of injury defined by the surgeon: intact, 0; indeterminate disruption, 2; and definitive disruption, 3. Studies evaluating the reliability of classifying the integrity of the PLC had only fair interrater reliability (κ = 0.336) and moderate intrarater reliability (κ = 0.4777).15,54 Given that intrarater reliability was better than interrater reliability, Harrop and coworkers concluded that this reflects individuals’ varying definitions of PLC integrity. The TLICS system allows the treating surgeon to evaluate the posterior ligamentous structures according to clinical and imaging findings (radiographs, CT reconstructions, and MRI). The authors of the TLICS system make a strong case for using MRI to define the integrity of the PLC, although it is recognized that MRI is not universally available and hence may not always be used in this assessment.
Management Considerations
Decision Making for Nonsurgicalversus Surgical Treatment
Historically, management of unstable fractures involved 6 to 8 weeks of recumbency with strict attention to maintenance of lumbar lordosis.54 As expected, such recumbency was associated with significant patient mortality and morbidity. Postural reduction, casting, and mobilization developed to avoid complications associated with prolonged bed rest.55 The popularity of nonoperative management has waned with the development of improved surgical techniques and spinal instrumentation systems that allow segmental fixation of the spine. With the widespread use of spinal instrumentation (in particular, pedicle screw-rod constructs), achieving immediate stability and allowing rapid mobilization have become commonplace in the management of thoracolumbar injuries. Nonetheless, the most difficult decisions to make are often related to whether a given injury is “unstable enough” to warrant the immediate stability afforded by spinal instrumentation, and it is for this reason that schemes such as the TLICS system have been developed to give surgeons some guidance in this area.
The general goals for surgical management of all spinal fractures are similar: decompress neural structures, correct deformity, and stabilize the spine. Achievement of these goals is intended to maximize neurological function, allow early mobilization and rehabilitation, and prevent deformity and pain. There is little debate that surgical intervention has far greater potential than nonsurgical treatment to correct deformity, decompress neural structures, and provide immediate stability. What complicates decision making in the management of thoracolumbar injuries (and in particular, burst fractures) is the fact that the relationship between residual kyphotic deformity and outcome is somewhat obscure (i.e., the presence of kyphosis does not necessarily condemn a patient to a poor outcome) and that many injuries are “stable enough” to not actually require the immediate stability afforded by internal fixation. Surgery brings with it the risk for infection and potential iatrogenic neural injury, and although it has been shown to shorten hospital stay, the complications that occur from surgery are not insignificant.56
In general, surgery for thoracolumbar injuries can be done from a posterior, anterior, or combined approach, depending on the goal of the procedure. The posterior approach is familiar to all surgeons and is the most commonly used. Posterior pedicle screw instrumentation is commonplace as the technique used for achieving rigid stability. In principle, correction of sagittal- and coronal-plane deformity is most easily achieved with posterior instrumentation, and thus pedicle screw fixation is particularly effective for fracture-dislocations with segmental translation or for flexion-distraction injuries with severe kyphosis. The corollary to this statement is that translational or rotational deformity, in general, is very difficult to reduce from the anterior aspect of spine, and it would be inadvisable to approach the spine anteriorly with the intent of achieving correction of deformity in this regard (although instrumentation that allows distraction of the anterior column can, to some extent, correct a purely kyphotic deformity). For flexion-distraction injuries, particularly those in which there is pure distraction and little to no anterior column injury, a short posterior pedicle screw construct will suffice to simply restore the posterior tension band of the spine. For burst fractures in which a fragment of bone (the “burst fragment”) is retropulsed into the spinal canal and compressing the ventral aspect of the dura, posterior distraction across the pedicle screws can indirectly reduce the burst fragment via a ligamentotaxis effect made possible by the integrity of Sharpey’s fibers and their attachments to the burst fragment. Reduction of the burst fragment can also be facilitated by using an instrument to tamp it forward (Fig. 319-9). Reduction of the fragment and ventral decompression of the neural elements can be confirmed with intraoperative ultrasound (Fig. 319-10). One should be wary of the fact that posterior distraction across pedicle screws tends to induce kyphosis, which would be undesirable in such a setting. This can be avoided at the thoracolumbar junction by bending the rods into a slight degree of lordosis and using fixed-angle or side-loading screws, which allow one to lever down on the proximal aspect of the cephalad screws (thus tipping up the distal shaft of the screws and tilting the vertebral body into lordosis). In addition, the rationale of distraction is applicable only to axial-loading injuries in which the PLC and posterior longitudinal ligament/Sharpey’s fibers are intact—indiscriminant distraction when these structures have been disrupted will just overdistract the segment (and will be unlikely to indirectly reduce the burst fragment).
An anterior approach is used primarily for thoracolumbar burst fractures in which vertebrectomy and anterior reconstruction are required either to decompress retropulsed fragments of bone directly off the ventral dura or to provide immediate restoration of the anterior weight-bearing column for reasons of mechanical stability. In principle, in the setting of severe neurological deficit as the result of a large retropulsed piece of bone, an anterior approach and vertebrectomy provide the most direct and ensured decompression of the neural elements for thoracolumbar burst fractures. Such injuries may be reconstructed with a cage implant (using autogenous bone graft from the vertebrectomy) or structural allograft and then stabilized with a variety of different anterior fixation screw-plate devices or rod-screw constructs. If the posterior elements are intact, such fixation may suffice to provide stability to the injury. If significant disruption of the PLC has occurred, it may be prudent to back up such an anterior construct with posterior pedicle screw-rod fixation. The drawback of the anterior approach is risk to retroperitoneal or intraperitoneal structures and the potential morbidity associated with the approach itself. Obesity, previous abdominal surgery, and severe pulmonary, chest, and abdominal trauma can limit use of the anterior approach.57
Circumferential stabilization is not necessary for most thoracolumbar injuries. The most common setting for 360-degree instrumentation is burst fractures with significant PLC disruption. Frequently, these injuries are difficult to classify as an isolated burst fracture or a fracture-dislocation because they share the features of severe anterior column compressive failure (like a burst fracture) with the displacement associated with a dislocation. Such injuries may require vertebrectomy and anterior reconstruction, followed by posterior pedicle screw instrumentation. Anterior column reconstruction can also be performed posterolaterally from a costotransversectomy approach, thereby obviating the need for formal anterior retroperitoneal exposure. In such cases, the use of expandable cages makes anterior reconstruction from a posterior approach much easier (Fig 319-11). The other area in which circumferential fixation is often necessary is in the lower lumbar spine (e.g., for L4 or L5 fractures). Here, although one may perform the vertebrectomy and reconstruction from the anterior approach, it is typically impossible to place a stabilizing rod or plate construct. These injuries therefore require posterior pedicle screw instrumentation as a backup.
In cases in which surgical treatment is chosen, again, the optimal management in some cases may be quite controversial. The literature on surgical management consists of mainly small institutional observational studies.58 Verlaan and colleagues in 2004 took on the task of performing a systematic review of the literature to identify what evidence exists on the surgical techniques, their performance, and complication rates for the management of thoracic and lumbar spine fractures.59 This paper highlighted the heterogeneity in thoracolumbar management among institutions and surgeons. In attempts to move toward evidence-based guidelines, the STSG in 2006 surveyed 22 leading spine surgeons in the field of trauma to define the major characteristics of thoracolumbar injuries that influenced their surgical decision making. These characteristics were then used to produce six possible clinical scenarios based on fracture morphology, integrity of the PLC, and patient neurological status.29 The goal of this initiative was to use an evidence-based approach to produce a user-friendly guideline for directing surgical treatment. The guidelines are meant to be the basis for ongoing randomized trials to generate class I evidence for future guidelines. The systematic review by Verlaan and associates and the results of consensus panel review by Vaccaro and colleagues from the STSG form the basis for the following discussion on surgical decision making.
Neurologically Intact/Posterior Ligamentous Complex Intact
This group of injuries is largely represented by burst fractures in which the PLC is intact and the neural elements have escaped injury (Fig. 319-12). Given the favorable natural history of these injuries, the panel thought that in most cases no surgical intervention is required. If surgery is chosen to promote early rehabilitation, the group was split between anterior (41%) and posterior (59%) approaches.
There is evidence that supports a conservative approach. A systematic review by Thomas and colleagues compared operative and nonoperative treatment of thoracolumbar burst fractures in patients without neurological deficit.60 They concluded that there was a lack of evidence to state that surgery was superior to conservative treatment. Moreover, no evidence exists to demonstrate that posttraumatic kyphosis is linked to clinical outcome. Even if some reduction is achieved, burst fractures with an intact PLC tend to return to their immediate postinjury kyphotic angle after 3 months.61 The systematic review on the surgical treatment of traumatic fractures of the thoracic and lumbar spine by Verlaan and coauthors demonstrated that all patients at follow-up had similar degrees of kyphosis regardless of surgical technique (Fig. 319-13).59
Neurologically Intact/Posterior Ligamentous Complex Disrupted
This scenario is associated with all injury morphologies, including flexion-distraction and severe compression injuries (Fig. 319-14).62,63 There was 91% agreement between panel members that this injury is best stabilized from a posterior approach. The group agreed that a combination approach is required if the anterior column is severely comminuted.
Incomplete Neurological Deficit or Cauda Equina Injury/Posterior Ligamentous Complex Intact
This injury is typically seen with burst fractures (with pure axial loading and no significant distraction through the posterior elements) and more rarely with extension-distraction injuries (Fig. 319-15). These injuries probably benefit the most from early surgical intervention to decompress the neural elements. If anterior neural compression is demonstrated, 91% of the panel members agreed that a direct anterior approach and decompression followed by arthrodesis with a strut graft/cage and anterior instrumentation should be done. A point emphasized in the guidelines is that all distraction or translational injuries should be stabilized posteriorly before anterior decompression. This holds true regardless of neurological status and PLC integrity.
Incomplete Neurological Deficit Or Cauda Equina Injury/Posterior Ligamentous Complex Disrupted
This injury pattern is typically seen with severe burst fractures, flexion-distraction fractures, and translational injuries (Fig. 319-16). There was 82% agreement that a combined anterior and posterior approach was required. Radiologic evidence of anterior compression required direct decompression. Although anterior decompression is a priority, the panel members thought that distraction or translational injuries required posterior alignment and stabilization before anterior/posterolateral decompression.
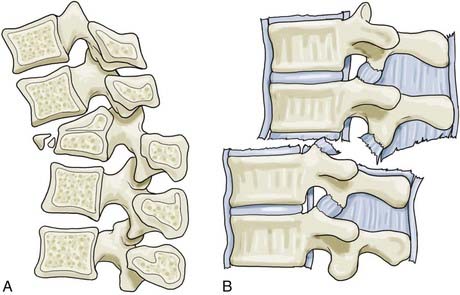
FIGURE 319-16 A, Flexion-distraction injury with translation. B, Pure translational injury.
(From Vaccaro A, Lim M, Hurlbert R, et al. Surgical decision making for unstable thoracolumbar spine injuries: results of a consensus panel review by the Spine Trauma Study Group. J Spinal Disord Tech. 2006;19:1-10.)
Complete Neurological Deficit/Posterior Ligamentous Complex Intact
This injury is typically seen with severe burst fractures and extension-distraction fractures. Given that functional neurological recovery is unlikely,64 55% of the panel members agreed a posterior approach for stabilization and realignment was sufficient. The other 45% thought that anterior decompression to avoid posttraumatic syringomyelia plus reconstruction of the anterior column was the optimal approach. In an experimental model, Cho and colleagues demonstrated that the arachnoid block produced by trauma and arachnoiditis is involved in the development of posttraumatic syringomyelia.65 Clinical evidence has demonstrated the relationship between posttraumatic deformity/stenosis and syrinx formation.66,67 Patients undergoing laminectomy as part of their procedure also have a significantly reduced incidence of posttraumatic syrinx formation. Surgery to reestablish the subarachnoid space may be beneficial, but prospective trials on patients with acute spinal cord injury are lacking.
Treatment Based on Fracture Morphology
Wedge Compression Fractures
Wedge compression fractures are morphologically characterized by their involvement of only the anterior aspect of the vertebral body, with the posterior aspect of the vertebral body and the PLC being left intact. These fractures are stable, do not cause neurological deficits, and heal uneventfully without an external orthosis. The greatest danger with this fracture morphology is misclassification, and in this regard one must be extremely cautious that the PLC is intact and, indeed, that the injury is restricted to the anterior part of the vertebral body. A severe flexion-distraction force can tear the PLC, cause only minimal anterior fracturing (because of the distraction), and then reduce back to a nearly anatomic position, thus giving this highly unstable injury the very benign appearance of an anterior wedge compression fracture (Fig. 319-17). If clinical or radiographic signs of PLC disruption are present, MRI is necessary. Additionally, any history of neurological deficit or clinical signs of neurological impairment mandate MRI to rule out not only PLC injury but also acute disk herniation. Individuals with significant loss of vertebral height, kyphosis, previous laminectomies, or persistent pain may be considered for short-segment pedicle screw stabilization or cement augmentation.
Burst Fractures
Management of thoracolumbar burst fractures in a neurologically intact or compromised individual is the most controversial topic. Traditionally, treatment of burst fractures without neurological compromise was based on unsubstantiated dogma regarding the extent of anterior height loss, localized kyphosis, or percentage of canal compromised (or any combination of these findings). We now recognize that the most important factor in determining the stability of these injuries is not these parameters, but rather the integrity of the PLC. Neurologically intact patients with a burst fracture and an intact PLC can be viewed as having a stable injury and can thus be treated nonoperatively in the large majority of cases. Surgery in such patients is unlikely to promote a better outcome. Strong evidence to support this statement comes from the systematic review of surgical and nonsurgical treatment of burst fractures published in 2006 by Thomas and colleagues.60 In this review, approximately 90% of patients returned to a “satisfactory” work level,68 although scores on the 36-item short-form health survey (SF-36) did not return to the level of age-matched peers.69 Surgically treated patients tended to rate their pain higher than did nonoperative patients.70 Almost 50% of patients treated nonoperatively were free of pain at follow-up.82 Surgically treated patients tended to have worse functional/disability outcome scores (Oswestry Disability Index, Roland-Morris Disability Scale) than their nonsurgically treated counterparts.84 Importantly, Thomas and associates’ systematic review showed that most investigators found no correlation between the final kyphotic angle and pain or functional outcome.60 This is an important point given that some surgeons remain fixated on the concept of using the immediate postinjury kyphosis as a guide to treatment without considering the integrity of the PLC. Regardless of treatment, neurological deterioration of patients is rarely reported.60 Given the evidence, the added risk associated with surgery is unjustified in the majority of patients who are considered to be mechanically stable.
It is worth noting that although surgery may not be required for such injuries, the nature of what constitutes appropriate nonoperative management is not so well defined. Bed rest followed by mobilization in a cast or thoracolumbar orthosis for up to 16 weeks was recommended at one point, but it was demonstrated that bed rest did little to prevent further kyphotic progression of the injury.71 Hence, such neurologically intact patients should be mobilized as soon as possible. It has also traditionally been thought that a thoracolumbar orthosis is necessary to provide some stability to these patients. This concept is also being challenged, and the interim results of a prospective randomized study of bracing versus no bracing for such fractures has failed to demonstrate any advantage with bracing. It is important to recognize that the fractures of these patients tend to fall into some kyphosis, regardless of whether they are treated with a brace, but this radiographic phenomenon does not appear to influence clinical outcome.
When deciding to operate for the purpose of improving neurological function, decompression of neural structures with stable internal fixation over the fewest segments is the goal. The best way to approach this objective, however, has not been established. The anterior approach allows direct neural decompression and reconstruction of the weight-bearing column by corpectomy, followed by structural bone graft and instrumentation involving only two motion segments.72 This approach does maintain sagittal alignment better than a posterior-only approach does, although it is recognized that there is no strong evidence that clinical outcome is related to late kyphosis.73 Additionally, at the thoracolumbar junction it is often necessary to use a thoracoabdominal approach and take down the diaphragm. Quantifying the morbidity of this approach against that of a standard posterior approach for this particular injury has not been done. In cases in which the posterior ligaments have been disrupted, anterior reconstruction may need to be supplemented with posterior instrumentation, although short-segment constructs in these cases may be acceptable with anterior column support (Fig. 319-18).
To address the incompetence of the anterior column without performing a formal reconstruction, augmentation with bone cement has generated increasing interest. Oner and coworkers developed a technique of end-plate reduction and kyphoplasty with short-segment pedicle screw fixation.74 Toyone and colleagues treated 15 consecutive patients with thoracolumbar burst fractures and incomplete neurological injury by indirect reduction and short-segment pedicle screw fixation, followed by transpedicular intracorporeal hydroxyapatite grafting to the fractured vertebra.75 Mean canal diameters improved postoperatively and at follow-up. Reduction was essentially maintained in all patients. Fourteen of 15 patients had less than 4 degrees of delayed kyphosis. No complications (infection, iatrogenic neurological deficit) or revisions occurred in their study population. Korovessis and colleagues used posterior pedicle screw constructs and kyphoplasty with calcium phosphate cement to treat 23 consecutive patients.76 Transpedicular balloon kyphoplasty was used for local reduction rather than traditional indirect distraction over multiple levels. No iatrogenic neurological injuries occurred. These preliminary studies demonstrate that posterior pedicle screw constructs followed by cement augmentation of the vertebral body may offer an alternative to more lengthy reconstructions of the anterior column in some settings.
Flexion-Distraction Injuries
Patients with flexion-distraction injuries have not been extensively studied with regard to long-term outcome. Finkelstein and coworkers found that patients treated with single-level posterior instrumentation maintained sagittal alignment and had minimal disability at follow-up.77 In a study performed at our institution, 40 patients with flexion-distraction injuries were monitored to assess radiographic, generic, and disease-specific health-related quality-of-life (HRQoL) outcomes.78 The HRQoL outcomes in patients without spinal cord injury were favorable overall but, somewhat surprisingly, did not return to baseline after 3 years. There was no relationship between the degree of initial or final kyphosis and outcome. This is somewhat of a surprising result given that it was expected that such injuries heal quite reliably and frequently with very little fixation required. Clearly, there are aspects of this injury that do not recover once bony stability has been achieved.
Fracture-Dislocations
Generally, this injury is always unstable and often associated with significant neurological compromise. These patients often have significant associated chest, abdominal, and pelvic injuries.79 Operative intervention is required for reduction and stabilization. A posterior approach usually achieves spinal alignment. A staged anterior procedure may be required if there is persistent ventral compression.
Complications
Complications associated with immobilization occur in both nonoperatively and operatively managed patients. Infection and implant failure are unique to operatively managed patients. In one large retrospective study of 117 patients treated surgically, the average wound infection rate was 10%, but in patients with complete neurological injuries, the infection rate climbed to 40%.80 Knop and colleagues reviewed the complications in 682 patients undergoing surgery for traumatic thoracolumbar fractures.81 Overall, 15% of patients experienced a complication in the intraoperative or postoperative period, with 6% requiring revision. As expected, the anterior approach had an increased rate of complications (40%). Few patients in this study underwent an anterior approach, which may have inaccurately inflated the complication rates. The lateral extracavitary approach allows ventral decompression and posterior fixation through a single incision, thereby avoiding an anterior approach. Although it may seem much safer, this approach can be associated with significant blood loss and pulmonary complications.82
Conclusion
Management of thoracolumbar injuries is an evolving process. Clearly, the literature on this topic does not contain a great deal of high-quality evidence in the form of randomized controlled trials (RCTs). Substantial obstacles to RCTs in all surgical fields are well described.83 Strong surgeon preferences, hesitancy to randomize patients, difficulty standardizing interventions, difficulty recruiting acutely unwell patients, and monitoring this typically young population are common obstacles to performing RCTs involving spine trauma patients. Unfortunately, these barriers have limited the ability to produce evidence-based management strategies for these injuries. Given the clinical equipoise that exists, there has been a movement toward removing these barriers in an attempt to produce higher quality evidence. The STSG’s ongoing development of the TLICS system along with the complementary consensus panel guidelines on surgical management is a first step. Stadhouder and colleagues identified the factors precluding RCTs in patients with thoracolumbar injuries and described the use of clinical equipoise to improve the methodology of retrospective observational studies.58 Regardless of the barriers that do exist, evidence from mainly smaller scale retrospective studies and systematic reviews has given a good indication of the outcomes in patients with thoracolumbar injuries. This field is evolving, and increasing use of minimal-access technology and methods for reconstructing the anterior column without formal vertebrectomy techniques will undoubtedly change the way that we view and treat these injuries over time.
Alanay A, Yazici M, Acaroglu E, et al. Course of nonsurgical management of burst fractures with intact posterior ligamentous complex: an MRI study. Spine. 2004;29:2425-2431.
Chapman JR, Agel J, Jurkovich GJ, et al. Thoracolumbar flexion-distraction injuries: associated morbidity and neurological outcomes. Spine. 2008;33:648-657.
Denis F. The three column spine and its significance in the classification of acute thoracolumbar spinal injuries. Spine. 1983;8:817-831.
Finkelstein JA, Wai EK, Jackson SS, et al. Single-level fixation of flexion distraction injuries. J Spinal Disord Tech. 2003;16:236-242.
Harrop J, Vaccaro A, Hurlbert R, et al. Intrarater and interrater reliability and validity in the assessment of the mechanism of injury and integrity of the posterior ligamentous complex: a novel injury severity scoring system for thoracolumbar injuries. Invited submission from the Joint Section Meeting on Disorders of the Spine and Peripheral Nerves, March 2005. J Neurosurg Spine. 2006;4:118-122.
Holdsworth F. Fractures, dislocations, and fracture-dislocations of the spine. J Bone Joint Surg Am. 1970;52:1534-1551.
Korovessis P, Repantis T, Petsinis G, et al. Direct reduction of thoracolumbar burst fractures by means of balloon kyphoplasty with calcium phosphate and stabilization with pedicle-screw instrumentation and fusion. Spine. 2008;33(4):E100-E108.
Kuklo TR, Polly DW, Owens BD, et al. Measurement of thoracic and lumbar fracture kyphosis: evaluation of intraobserver, interobserver, and technique variability. Spine. 2001;26:61-65.
Lee HM, Kim HS, Kim DJ, et al. Reliability of magnetic resonance imaging in detecting posterior ligament complex injury in thoracolumbar spinal fractures. Spine. 2000;25:2079-2084.
Magerl F, Aebi M, Gertzbein S, et al. A comprehensive classification of thoracic and lumbar injuries. Eur Spine J. 1994;3:184-201.
McAfee P, Yuan H, Fredrickson B, et al. The value of computed tomography in thoracolumbar fractures. An analysis of one hundred consecutive cases and a new classification. J Bone Joint Surg Am. 1983;65:461-473.
Oner FC, Ramos LM, Simmermacher RK, et al. Classification of thoracic and lumbar spine fractures: problems of reproducibility. A study of 53 patients using CT and MRI. Eur Spine J. 2002;11:235-245.
Oner FC, van Gils AP, Faber JA, et al. Some complications of common treatment schemes of thoracolumbar spine fractures can be predicted with magnetic resonance imaging: prospective study of 53 patients with 71 fractures. Spine. 2002;27:629-636.
Oner FC, Verlaan JJ, Verbout AJ, et al. Cement augmentation techniques in traumatic thoracolumbar spine fractures. Spine. 2006;31(11 Suppl):S89-S95.
Thomas KC, Bailey CS, Dvorak MF, et al. Comparison of operative and nonoperative treatment for thoracolumbar burst fractures in patients without neurological deficit: a systematic review. J Neurosurg. 2006;4:351-358.
Vaccaro A, Baron E, Sanfilippo J, et al. Reliability of a novel classification system for thoracolumbar injuries: the Thoracolumbar Injury Severity Score. Spine. 2006;31(11 suppl):S62-S69.
Vaccaro A, Lim M, Hurlbert R, et al. Surgical decision making for unstable thoracolumbar spine injuries: results of a consensus panel review by the Spine Trauma Study Group. J Spinal Disord Tech. 2006;19:1-10.
Vaccaro A, Zeiller S, Hulbert R, et al. The Thoracolumbar Injury Severity Score: a proposed treatment algorithm. J Spinal Disord Tech. 2005;18:209-215.
Vaccaro AR, An HS, Lin S, et al. Noncontiguous injuries of the spine. J Spinal Disord. 1992;5:320-329.
Vaccaro AR, Lee JY, Schweitzer J, et al. Assessment of injury to the posterior ligamentous complex in thoracolumbar spine trauma. Spine J. 2006;6:524-528.
Verlaan J, Diekerhof C, Buskens E, et al. Surgical treatment of traumatic fractures of the thoracic and lumbar spine: a systematic review of the literature on techniques, complications, and outcome. Spine. 2004;29:803-814.
Wood K, Buttermann G, Mehbod A, et al. Operative compared with nonoperative treatment of a thoracolumbar burst fracture without neurological deficit: a prospective, randomized study. J Bone Joint Surg Am. 2003;85:773-781.
Wood KB, Khanna G, Vaccaro AR, et al. Assessment of two thoracolumbar fracture classification systems as used by multiple surgeons. J Bone Joint Surg Am. 2005;87:1423-1429.
1 Cooper C, Dunham CM, Rodriguez A. Falls and major injuries are risk factors for thoracolumbar fractures: cognitive impairment and multiple injuries impede the detection of back pain and tenderness. J Trauma. 1995;38:692-696.
2 Frankel HL, Rozycki GS, Ochsner MG, et al. Indications for obtaining surveillance thoracic and lumbar spine radiographs. J Trauma. 1994;37:673-676.
3 Samuels LE, Kerstein MD. “Routine” radiologic evaluation of the thoracolumbar spine in blunt trauma patients: a reappraisal. J Trauma. 1993;34:85-89.
4 Terregino CA, Ross SE, Lipinski MF, et al. Selective indications for thoracic and lumbar radiography in blunt trauma. Ann Emerg Med. 1995;26:126-129.
5 Sheridan R, Peralta R, Rhea J, et al. Reformatted visceral protocol helical computed tomographic scanning allows conventional radiographs of the thoracic and lumbar spine to be eliminated in the evaluation of blunt trauma patients. J Trauma. 2003;55:665-669.
6 Brandser EA, el-Khoury GY. Thoracic and lumbar spine trauma. Radiol Clin North Am. 1997;35:533-557.
7 Saboe LA, Reid DC, Davis LA, et al. Spine trauma and associated injuries. J Trauma. 1991;31:43-48.
8 Reid D, Henderson R, Saboe L, et al. Etiology and clinical course of missed spine fractures. J Trauma. 1987;27:980-986.
9 Miyanji F, Fisher C, Keynan O, et al. Top Spinal Cord Inj Rehabil. 2006;12:58-69.
10 Hsu JM, Joseph T, Ellis AM. Thoracolumbar fracture in blunt trauma patients: guidelines for diagnosis and imaging. Injury. 2003:426-433.
11 Meldon SW, Moettus LN. Thoracolumbar spine fractures: clinical presentation and the effect of altered sensorium and major injury. J Trauma. 1995;39:1110-1114.
12 Richter D, Hahn MP, Ostermann PA, et al. Vertical deceleration injuries: a comparative study of the injury patterns of 101 patients after accidental and intentional high falls. Injury. 1996;27:655-659.
13 Vaccaro AR, An HS, Lin S, et al. Noncontiguous injuries of the spine. J Spinal Disord. 1992;5:320-329.
14 Andriacchi T, Schultz A, Belytschko T, et al. A model for studies of mechanical interactions between the human spine and rib cage. J Biomech. 1974;7:497-507.
15 Harrop J, Vaccaro A, Hurlbert R, et al. Intrarater and interrater reliability and validity in the assessment of the mechanism of injury and integrity of the posterior ligamentous complex: a novel injury severity scoring system for thoracolumbar injuries. Invited submission from the Joint Section Meeting on Disorders of the Spine and Peripheral Nerves, March 2005. J Neurosurg Spine. 2006;4:118-122.
15a Watson-Jones R. Fractures and Joint Injuries, 3rd ed. Edinburgh, Scotland: ES Livingston; 1943.
16 Viejo-Fuertes D, Liguoro D, Rivel J, et al. Morphologic and histologic study of the ligamentum flavum in the thoraco-lumbar region. Surg Radiol Anat. 1998;20:171-176.
17 Dickey JP, Bednar DA, Dumas GA. New insight into the mechanics of the lumbar interspinous ligament. Spine. 1996;21:2720-2727.
18 Adams MA, Hutton WC, Stott JR. The resistance to flexion of the lumbar intervertebral joint. Spine. 1980;5:245-253.
19 Bogduk N. Clinical Anatomy of Lumbar Spine and Sacrum. 4th ed. London: Churchill Livingstone. 2005.
20 Malas MA, Salbacak A, Büyükmumcu M, et al. An investigation of the conus medullaris termination level during the period of fetal development to adulthood. Kaibogaku Zasshi. 2001;76:453-459.
21 Soleiman J, Demaerel P, Rocher S, et al. Magnetic resonance imaging study of the level of termination of the conus medullaris and the thecal sac: influence of age and gender. Spine. 2005;30:1875-1880.
22 Bauer D, Shoja M, Loukas M, et al. Study of the effects of flexion on the position of the conus medullaris. Childs Nerv Syst. 2008;24:1043-1045.
23 Lu J, Ebraheim NA, Biyani A, et al. Vulnerability of great medullary artery. Spine. 1996;21:1852-1858.
24 Marino RJ, Barros T, Biering-Sorensen F, et al. International standards for neurological classification of spinal cord injury. J Spinal Cord Med. 2003;26(suppl 1):S50-S56.
25 Baldwin KM, Ziegler SM. Pressure ulcer risk following critical traumatic injury. Adv Wound Care. 1998;11:168-173.
26 Mawson A, Biundo JJr, Neville P, et al. Risk factors for early occurring pressure ulcers following spinal cord injury. Am J Phys Med Rehabil. 1988;67:123-127.
27 Lerner EB, Moscati R. Duration of patient immobilization in the ED. Am J Emerg Med. 2000;18:28-30.
28 Rogers LF, Taljanovic MS, Boles CA. Skeletal trauma. In Grainger RC, Allison D, Dixon AK, editors: Diagnostic Radiology: A Textbook of Medical Imaging, 5th ed, New York: Churchill Livingstone, 2008.
29 Vaccaro A, Lim M, Hurlbert R, et al. Surgical decision making for unstable thoracolumbar spine injuries: results of a consensus panel review by the Spine Trauma Study Group. J Spinal Disord Tech. 2006;19:1-10.
30 Kuklo TR, Polly DW, Owens BD, et al. Measurement of thoracic and lumbar fracture kyphosis: evaluation of intraobserver, interobserver, and technique variability. Spine. 2001;26:61-65.
31 Gehweiler JAJr, Daffner RH, Osborne RLJr. Relevant signs of stable and unstable thoracolumbar vertebral column trauma. Skeletal Radiol. 1981;7:179-183.
32 Daffner RH, Deeb ZL, Goldberg AL, et al. The radiologic assessment of post-traumatic vertebral stability. Skeletal Radiol. 1990;19:103-108.
33 Nagel DA, Koogle TA, Piziali RL, et al. Stability of the upper lumbar spine following progressive disruptions and the application of individual internal and external fixation devices. J Bone Joint Surg Am. 1981;63:62-70.
34 Dai LY, Yao WF, Cui YM, et al. Thoracolumbar fractures in patients with multiple injuries: diagnosis and treatment—a review of 147 cases. J Trauma. 2004;56:348-355.
35 Emery SE, Pathria MN, Wilber RG, et al. Magnetic resonance imaging of posttraumatic spinal ligament injury. J Spinal Disord. 1989;2:229-233.
36 Green R, Saifuddin A. Whole spine MRI in the assessment of acute vertebral body trauma. Skeletal Radiol. 2004;33:129-135.
37 Hyodoh H, Kawaharada N, Akiba H, et al. Usefulness of preoperative detection of artery of Adamkiewicz with dynamic contrast-enhanced MR angiography. Radiology. 2005;236:1004-1009.
38 Kliewer MA, Gray L, Paver J, et al. Acute spinal ligament disruption: MR imaging with anatomic correlation. J Magn Reson Imaging. 1993;3:855-861.
39 Terk MR, Hume-Neal M, Fraipont M, et al. Injury of the posterior ligament complex in patients with acute spinal trauma: evaluation by MR imaging. AJR Am J Roentgenol. 1997;168:1481-1486.
40 Lee HM, Kim HS, Kim DJ, et al. Reliability of magnetic resonance imaging in detecting posterior ligament complex injury in thoracolumbar spinal fractures. Spine. 2000;25:2079-2084.
41 Vaccaro AR, Lee JY, Schweitzer J, Karl M, et al. Assessment of injury to the posterior ligamentous complex in thoracolumbar spine trauma. Spine J. 2006;6:524-528.
42 Fassett DR, Politi R, Patel A, et al. Classification systems for acute thoracolumbar trauma. Curr Opin Orthop. 2007;18:253-258.
43 Oner FC, Ramos LM, Simmermacher RK, et al. Classification of thoracic and lumbar spine fractures: problems of reproducibility. A study of 53 patients using CT and MRI. Eur Spine J. 2002;11:235-245.
44 Wood KB, Khanna G, Vaccaro AR, et al. Assessment of two thoracolumbar fracture classification systems as used by multiple surgeons. J Bone Joint Surg Am. 2005;87:1423-1429.
45 Bohler L. Die Techniek deknochenbruchbehandlung Imgrieden und im Kreigen. Verlag von Wilhelm Maudrich. 1930.
46 Holdsworth F. Fractures, dislocations, and fracture-dislocations of the spine. J Bone Joint Surg Am. 1970;52:1534-1551.
47 Roaf R. A study of the mechanics of spinal injuries. J Bone Joint Surg Br. 1960;42:801-823.
48 Denis F. The three column spine and its significance in the classification of acute thoracolumbar spinal injuries. Spine. 1983;8:817-831.
49 McAfee P, Yuan H, Fredrickson B, et al. The value of computed tomography in thoracolumbar fractures. An analysis of one hundred consecutive cases and a new classification. J Bone Joint Surg Am. 1983;65:461-473.
50 Magerl F, Aebi M, Gertzbein S, et al. A comprehensive classification of thoracic and lumbar injuries. Eur Spine J. 1994;3:184-201.
51 Wood K, Khanna G, Vaccaro A, et al. Assessment of two thoracolumbar fracture classification systems as used by multiple surgeons. J Bone Joint Surg Am. 2005;87:1423-1429.
52 Vaccaro A, Zeiller S, Hulbert R, et al. The Thoracolumbar Injury Severity Score: a proposed treatment algorithm. J Spinal Disord Tech. 2005;18:209-215.
53 Vaccaro A, Baron E, Sanfilippo J, et al. Reliability of a novel classification system for thoracolumbar injuries: the Thoracolumbar Injury Severity Score. Spine. 2006;31(11 suppl):S62-S69.
54 Bedbrook GM. Treatment of thoracolumbar dislocation and fractures with paraplegia. Clin Orthop Relat Res. 1975;112:27-43.
55 Chow GH, Nelson BJ, Gebhard JS, et al. Functional outcome of thoracolumbar burst fractures managed with hyperextension casting or bracing and early mobilization. Spine. 1996;21:2170-2175.
56 Rechtine GRII, Cahill D, Chrin AM. Treatment of thoracolumbar trauma: comparison of complications of operative versus nonoperative treatment. J Spinal Disord. 1999;12:406-409.
57 Kirkpatrick JS. Thoracolumbar fracture management: anterior approach. J Am Acad Orthop Surg. 2003;11:355-363.
58 Stadhouder A, Buskens E, de Klerk LW, et al. Traumatic thoracic and lumbar spinal fractures: operative or nonoperative treatment: comparison of two treatment strategies by means of surgeon equipoise. Spine. 2008;33:1006-1017.
59 Verlaan J, Diekerhof C, Buskens E, et al. Surgical treatment of traumatic fractures of the thoracic and lumbar spine: a systematic review of the literature on techniques, complications, and outcome. Spine. 2004;29:803-814.
60 Thomas KC, Bailey CS, Dvorak MF, et al. Comparison of operative and nonoperative treatment for thoracolumbar burst fractures in patients without neurological deficit: a systematic review. J Neurosurg. 2006;4:351-358.
61 Alanay A, Yazici M, Acaroglu E, et al. Course of nonsurgical management of burst fractures with intact posterior ligamentous complex: an MRI study. Spine. 2004;29:2425-2431.
62 Terk MR, Hume-Neal M, Fraipont M, et al. Injury of the posterior ligament complex in patients with acute spinal trauma: Evaluation by MR imaging. AJR Am J Roentgenol. 1997;168:1481-1486.
63 Oner FC, van Gils AP, Faber JA, et al. Some complications of common treatment schemes of thoracolumbar spine fractures can be predicted with magnetic resonance imaging: prospective study of 53 patients with 71 fractures. Spine. 2002;27:629-636.
64 Bohlman HH, Freehafer A, Dejak J. The results of treatment of acute injuries of the upper thoracic spine with paralysis. J Bone Joint Surg Am. 1985;67:360-369.
65 Cho KH, Iwasaki Y, Imamura H, et al. Experimental model of posttraumatic syringomyelia: the role of adhesive arachnoiditis in syrinx formation. J Neurosurg. 1994;80:133-139.
66 Perrouin-Verbe B, Lenne-Aurier K, Robert R, et al. Post-traumatic syringomyelia and post-traumatic spinal canal stenosis: a direct relationship: review of 75 patients with a spinal cord injury. Spinal Cord. 1998;36:137-143.
67 Schurch B, Wichmann W, Rossier AB. Post-traumatic syringomyelia (cystic myelopathy): a prospective study of 449 patients with spinal cord injury. J Neurol Neurosurg Psychiatry. 1996;60:61-67.
68 Mumford J, Weinstein JN, Spratt KF, et al. Thoracolumbar burst fractures. The clinical efficacy and outcome of nonoperative management. Spine. 1993;18:955-970.
69 Briem D, Lehmann W, Ruecker AH, et al. Factors influencing the quality of life after burst fractures of the thoracolumbar transition. Arch Orthop Trauma Surg. 2004;124:461-468.
70 Wood K, Buttermann G, Mehbod A, et al. Operative compared with nonoperative treatment of a thoracolumbar burst fracture without neurological deficit: a prospective, randomized study. J Bone Joint Surg Am. 2003;85:773-781.
71 Willén J, Anderson J, Toomoka K, et al. The natural history of burst fractures at the thoracolumbar junction. J Spinal Disord. 1990;3:39-46.
72 McDonough PW, Davis R, Tribus C, et al. The management of acute thoracolumbar burst fractures with anterior corpectomy and Z-plate fixation. Spine. 2004;29:1901-1908.
73 Sasso RC, Renkens K, Hanson D, et al. Unstable thoracolumbar burst fractures: anterior-only versus short-segment posterior fixation. J Spinal Disord Tech. 2006;19:242-248.
74 Oner FC, Verlaan JJ, Verbout AJ, et al. Cement augmentation techniques in traumatic thoracolumbar spine fractures. Spine. 2006;31(11 suppl):S89-S95.
75 Toyone T, Tanaka T, Kato D, et al. The treatment of acute thoracolumbar burst fractures with transpedicular intracorporeal hydroxyapatite grafting following indirect reduction and pedicle screw fixation: a prospective study. Spine. 2006;31(7):E208-E214.
76 Korovessis P, Repantis T, Petsinis G, et al. Direct reduction of thoracolumbar burst fractures by means of balloon kyphoplasty with calcium phosphate and stabilization with pedicle-screw instrumentation and fusion. Spine. 2008;33(4):E100-E108.
77 Finkelstein JA, Wai EK, Jackson SS, et al. Single-level fixation of flexion distraction injuries. J Spinal Disord Tech. 2003;16:236-242.
78 Miyanji F, Fisher CG, Keynan O, et al. Flexion-distraction injuries of the thoracolumbar spine: health-related quality of life and radiographic outcomes. Top Spinal Cord Inj Rehabil. 2006;12:58-69.
79 Chapman JR, Agel J, Jurkovich GJ, et al. Thoracolumbar flexion-distraction injuries: associated morbidity and neurological outcomes. Spine. 2008;33:648-657.
80 Rechtine GR, Bono PL, Cahill D, et al. Postoperative wound infection after instrumentation of thoracic and lumbar fractures. J Orthop Trauma. 2001;15:566-569.
81 Knop C, Bastian L, Lange U, et al. Complications in surgical treatment of thoracolumbar injuries. Eur Spine J. 2002;11:214-226.
82 Resnick DK, Benzel EC. Lateral extracavitary approach for thoracic and thoracolumbar spine trauma: operative complications. Neurosurgery. 1998;43:796-802.
83 McCulloch P, Taylor I, Sasako M, et al. Randomised trials in surgery: problems and possible solutions. BMJ. 2002;324:1448-1451.