Chapter 11 Thoracic Aortic Disease
INTRODUCTION
Just as a primary cardiac problem can affect other organ systems, systemic disease or an abnormality in another organ system can secondarily cause cardiac dysfunction. Deciding which is the primary abnormality can pose a diagnostic dilemma. For example, in a patient with aortic regurgitation, is the cause intrinsic aortic valve disease with secondary aortic dilatation, or is it an aortic aneurysm with dilatation of the aortic annulus that prevents coaptation of the aortic leaflets? It is generally better to do noninvasive tests first—such as chest radiography, computed tomography (CT), echocardiography, and magnetic resonance imaging (MRI)—before invasive angiography to examine the aorta and the aortic root. These diseases require imaging of the thoracic aorta to size the aortic annulus and to detect stenoses or enlargement of the aorta (in addition to aortic valvular stenosis), fistulas to the cardiac chambers or systemic vessels, and intrinsic aortic wall abnormalities.
AORTIC ANATOMY AND SIZE
The size of the aorta is often critical for diagnosing aortic disease. Several measurements are useful in identifying the upper range of normal. On the frontal chest film the distance between the left border of the trachea and the lateral border of the aortic arch is always less than 4 cm in adults and usually less than 3 cm in those younger than 30 years of age. On an aortogram or tomographic scan of the ascending aorta, the normal diameter should be less than 4 cm (Table 11-1). Longitudinal enlargement is more difficult to quantitate but is manifest by tortuosity, occasional kinking or buckling, and displacement into the adjacent lung or mediastinum.
Mean (cm) | Upper Limit of Normal* (cm) | |
---|---|---|
Aortic root | 3.7 | 4.0 |
Ascending aorta | 3.2 | 3.7 |
Descending aorta | 2.5 | 2.8 |
* Two standard deviations above the mean.
From Aronberg DJ, Glazer HS, Madsen K, et al.: Normal thoracic aortic diameters by computed tomography, J Comput Assist Tomogr 8:247-250, 1984; Drexler M, Erbel R, Muller U, et al.: Measurement of intracardiac dimensions and structures in normal young adult subjects by transesophageal echocardiography, Am J Cardiol 65:1491-1496, 1990.
The diameter of the normal adult aorta has a wide range that gradually increases with age (Fig. 11-1).
AORTIC ANEURYSMS
Wall Mechanics
Wall stress or tension in any blood vessel is directly related to blood pressure and vessel radius. This finding is supported by Laplace’s law, whereby wall tension is proportional to the radius of the vessel at a given blood pressure. The larger the vessel radius, the greater the wall stress. Areas of wall weakness can and will expand, resulting in an even greater wall stress and an increase risk of rupture. Wall stress in aneurysmal blood vessels, however, is not uniform compared with adjacent nonaneurysmal vessel wall. The abrupt change in vessel radius in an aneurysmal segment results in significant wall tension differences throughout the aneurysm.
Size and Types
There are a number of definitions of thoracic aneurysm, but most are keyed on the size at which there is potential for rupture (Fig. 11-2). An aortic diameter greater than 1.5 times normal is a commonly accepted definition of aneurysm. For practical purposes, aneurysms in the ascending aorta are greater than 5 cm, and those in the descending aorta are greater than 4 cm.
True aneurysms have all elements of the aorta incorporated into the wall of the aneurysm. The most common true aneurysm is a fusiform aneurysm that involves the entire circumference of the aorta. A saccular aneurysm is an eccentric dilatation that involves one side of the aorta (Fig. 11-3). For example, most infected aneurysms are saccular. It can occur in a short segment or can involve the entire aorta. A vast majority of asymmetric or saccular aneurysms fall under the category of false or pseudoaneurysms. False aneurysms have a perforation into the intima and media. An example is an aortic transection from trauma.
A radiologic description of the aneurysm includes the location and extent, the type, and in conjunction with the clinical history, the cause. Box 11-1 lists the common associations, but many aneurysms fall into several categories. Atherosclerotic aneurysms are usually fusiform and occur in the inferior parts of the aorta. Infected aneurysms may be either true or false. Syphilitic aneurysms can be either saccular or fusiform. The list is not inclusive but serves as a starting point for diagnosis and management.
Chest Film Findings
The chest film is one of the first examinations obtained when thoracic aortic aneurysm, dissection, or transection is suspected, even though its sensitivity and specificity for these diagnoses are widely variable. The purpose of the chest film is to assess the size of the aorta and to identify a rupture. A valuable use of the chest film is to follow the mediastinal contours over a time interval to detect an increasing mediastinal width (Box 11-2). Tracheal or bronchial compression can be suspected when these structures are extrinsically deviated (Fig. 11-4). Compression of a pulmonary artery is recognized by unilateral pulmonary oligemia. For most of its thoracic course, the lesser curvature of the aorta is not visible on the chest film so the only signs of aortic enlargement are those of the greater curvature displacing adjacent structures. Other signs appear when the aorta has ruptured into the mediastinum. Unilateral pleural fluid or pericardial fluid usually indicates impending exsanguination or cardiac tamponade. Rupture into the mediastinum is initially constrained by the tissue in the mediastinum and pleural compartments. The chest film signs of aortic rupture are those of mediastinal hemorrhage (Box 11-3).
ACQUIRED DISEASES
Aortic Dissection
Although Morgagni recognized aortic dissection at necropsy over 200 years ago, even today it may be difficult to diagnose, and it certainly remains a therapeutic enigma. Most aortic dissections, or dissecting hematomas, at least in their early stages, are not aneurysms because they are not a localized enlargement of the aorta. The term dissecting aneurysm should be reserved for those cases in which the aorta (usually the false channel) is actually dilated. The clinical picture of a person with abrupt, severe chest pain associated with a loss of one or more peripheral pulses strongly suggests dissection; however, atypical onset of myocardial infarction, pulmonary or systemic emboli, musculoskeletal syndromes, and other chest pain syndromes may mimic the presence of aortic dissection, and therefore aortic imaging is mandatory for a definitive diagnosis. Similarly, a small percentage of dissections are “silent,” occurring without pain, and are discovered only from an abnormal chest film. In these cases, other types of thoracic aneurysm, penetrating aortic ulcer, or nonvascular mediastinal disease must be distinguished from aortic dissection.
Complications
Aortic rupture into the pericardium, pleural space, or mediastinum can be suggested on the plain chest film by a large heart diameter, pleural fluid, and a wide mediastinum. This heralds the need for immediate pericardiocentesis and other cardiopulmonary supportive measures. A dissection can partially or completely occlude a branch of the aorta by compression of the true channel by the false channel or by adjacent compression from an intimal flap. Any artery arising from the aorta can be occluded, but the right coronary artery and the three arch vessels are commonly affected. Surgical treatment aims at preventing retrograde tear into the heart and pericardium by resecting the segment that contains the entry tear. An interposition graft is then inserted, collapsing the false channel distally. Dissections in the aortic root are treated with a composite graft with the prosthetic aortic valve sewn to the graft and the coronary arteries replanted into the sides of the graft.
Plain Film Findings
The plain film findings of dissection are indirect but can suggest the need for further evaluation (Fig. 11-5). An abnormally wide mediastinum, separation of calcium from the wall of the aortic arch, a left apical pleural cap, pleural fluid, and displacement of the trachea and esophagus from the midline are important characteristics of a thoracic aortic abnormality. However, the chest film findings are insensitive for the detection of aortic dissection: Nearly one fifth of patients with dissection have normal chest films.
Tomographic Imaging
Computed tomography with intravenous contrast, magnetic resonance imaging (MRI), and echocardiography all have excellent sensitivity and specificity for detecting aortic dissection but each has limitations specific to its technology. Optimal computed tomography (CT) imaging is with intravenous contrast on a spiral scanner (Figures 11-6, 11-7). Spiral CT requires a correctly timed bolus of contrast media and has streak artifacts from the pulsating aortic wall that can mimic the intimal flap. Multidetector CT scanners with three-dimensional postprocessing reconstructions can rival the accuracy of catheter angiography.
Magnetic Resonance Imaging
Because of slow blood flow, clotted false channels, and occasionally the twisted shape of the intimal flap, aortic dissection can be difficult to distinguish from other types of aneurysm and aortitis (Fig. 11-8). MRI is particularly useful in these situations because different pulse sequences and reconstruction techniques can be exploited to produce a distinction between flowing blood and static tissue. Spin echo sequences producing “black blood” images easily show the intimal flap when there is moderate flow in both channels. But in regions of slowly flowing blood, the signal in that region may be similar to tissue in the aortic wall and adjacent mediastinum. A number of techniques exist that can image slow velocity differences and separate nonmoving and clotted blood in the false channel from slow-moving blood. Even echocardiographic rephasing, the fortuitous occurrence of velocity compensation in the second echocardiogram, is recognized as a higher signal intensity in the second echocardiogram in regions of slowly flowing blood (Fig. 11-9). A caveat is that failure of the signal intensity on the second echocardiogram to be greater than the first echocardiographic image is often observed in regions of complex velocity changes such as those that occur in vortices and eddies around bends in the aorta.
One of the most sensitive ways to make the distinction between thrombus and slowly flowing blood is to reconstruct the original data as a phase image. All MRIs are generated as complex numbers, which are typically reconstructed as magnitude images. However, the same data can be displayed as a phase image, which then becomes a picture of the velocity of the tissue within each pixel. The phase image needs to be interpreted with the magnitude image to identify the area of concern where a thrombosed channel may be present. Changes in signal intensity, including alternating white to black phase breaks in the phase image, indicate flowing blood (Fig. 11-10).
Another strategy that can be quite helpful is to obtain a gradient echo cine study through the area that has questionable flow in the standard spin echo image. With velocity compensation, the gradient echo pulse sequence should be obtained at only one slice to avoid the inflow of partly saturated spins from a neighboring slice (Fig. 11-11).
Unlike the other techniques, transesophageal echocardiography (TEE) can be carried out at the bedside. The major advantage over the other techniques is the quantitation of aortic regurgitation with color-flow Doppler. Its disadvantage is that the aortic arch cannot be completely imaged with either the transthoracic or transesophageal technique.
Catheter Approach
The site of catheterization will depend on which pulses are present. If no extremity pulses are felt, a pulmonary angiogram with delayed follow-through may show the dissection. However, a general principle is that angiography should be performed with an injection as close to the abnormality as possible, and therefore a femoral, axillary, or brachial arterial approach is preferred. Because the left iliac artery is most frequently involved with a dissection, the preferred route is a percutaneous transfemoral approach from the right side.
Signs of Dissection
The intimal flap, a lucency several millimeters thick outlined by contrast on both sides, is the hallmark of dissection. You can often identify the actual entry from one channel into another or into multiple channels and the subsequent flow of blood in either an antegrade or retrograde direction. This intimal tear may extend only a few centimeters (Fig. 11-12) or may extend the entire length of the aorta, even into peripheral vessels (Fig. 11-13). The leaflets of the aortic valve, particularly with annuloaortic ectasia or Marfan syndrome, may be effaced and appear as a lucency; the large aortic leaflets can be difficult to distinguish from a true intimal tear in the aortic root, particularly if large-film technique is used. Cine angiography usually resolves this problem. True tears may be further differentiated by their origin above the sinotubular ridge and extension backward into the valve.
An ulcerlike projection from the aorta may represent an early sign of dissection, although other types of aneurysms, including those caused by infection and penetrating atherosclerotic ulcer, may be associated with this finding. The base of the ulcer represents the defect in the intima leading to a thrombosed false channel (see Figure 11-13). In the descending aorta where side branches originate, the ulcer may be an occlusion or detachment of the intima from an intercostal artery.
The false channel may not opacify during angiography and, therefore, may present as a thick wall (usually >1 cm) along the greater curvature of the aorta (Fig. 11-14). An eccentric wall thickness greater than 1 cm is unusual in a clotted atherosclerotic or syphilitic aneurysm or aortitis. An unopacified channel may occasionally be seen if the injection was made proximal to the entry tear and the distal dissection has extended in a retrograde direction. Before the diagnosis of a thrombosed false channel is made, an injection should be made with the catheter at the level of the diaphragm to search for retrograde flow from a distant entry site. The thrombosed false channel has been called a “healed” dissection and is less liable to rupture late. Initially, the true channel is frequently smaller than the false channel, although either may enlarge to greater than the normal aortic width, then justifying the term dissecting aneurysm.
Aortic regurgitation may result from three mechanisms (Fig. 11-15):
In addition to occluding or transecting any vessels from the aorta, a dissection may compromise other mediastinal vessels. The expanding hematoma of the false channel may compress the right pulmonary artery. Similarly, it may both displace and compress the superior vena cava. On the left side of the mediastinum, because the false channel extends posterolaterally, the pulmonary veins are occasionally compressed. If this abnormality leads to reduced flow through the left lung, then a dissection may be confused with pulmonary embolism on a ventilationperfusion lung scan.
Annuloaortic Ectasia and Marfan Syndrome
Root Enlargement
In Marfan syndrome, the sentinel vascular abnormalities in the aorta are aortic regurgitation, fusiform dilatation of the aorta, and dissection. These are represented pathologically by cystic medial necrosis. Degeneration of the aortic media leads to dilatation of the annulus. The aortic leaflets are spread apart and aortic regurgitation ensues. However, patients who do not have other features of Marfan syndrome may have annuloaortic ectasia. The term annuloaortic ectasia describes pear-shaped dilatation of the sinuses of Valsalva and the proximal aorta. Persons without the Marfan syndrome but with annuloaortic ectasia are usually male (by a 2:1 ratio) and are typically first seen after age 40 (Fig. 11-19). Those with Marfan syndrome have clinical symptoms at a much younger age. The aorta in homocystinuria may have an identical appearance.
Marfan Syndrome Presentation
In contrast to isolated annuloaortic ectasia, Marfan syndrome is a generalized disorder of connective tissue demonstrating autosomal dominant inheritance and manifested by cardiovascular, ocular, and skeletal abnormalities. Involvement of the cardiovascular system occurs in more than half of affected adults. Dilatation of the aortic annulus and ascending aorta are usually the first abnormal signs. Later, aortic regurgitation appears, which may ultimately cause left ventricular failure. Aortic dissection is a common complication and is frequently the cause of death (Fig. 11-20). Mitral regurgitation is the most common cardiac abnormality in children and is the result of redundant, elongated chordae tendineae and overlarge leaflets that produce prolapse of the leaflets into the left atrium. Calcification of the mitral annulus in children may occasionally be seen.
Radiologic Techniques
Many of the clues to the diagnosis of Marfan syndrome are frequently visible on the chest film (Fig. 11-21). On the frontal film, the thoracic cage appears large and elongated with large-volume lungs. The heart may be shifted to the left from a narrow anteroposterior thoracic diameter. In normal young adults less than 20 years of age, the aorta should be inapparent. In contrast, aortic elongation and ectasia in this age group are common signs of Marfan syndrome. Cardiomegaly is usually nonspecific and may reflect only the pectus excavatum, but aortic regurgitation from annuloaortic ectasia and mitral regurgitation from prolapsing mitral leaflets are common conditions that pathologically enlarge the heart. On the lateral film, a pectus excavatum is frequently identified as well as a narrow thoracic diameter.
Marfan patients without symptoms are easily observed with serial MRI every 6 to 12 months. Surgical referral is usually undertaken if a previously stable aortic aneurysm begins to enlarge or if the aortic arch and descending aorta exceed a diameter of 5 cm. MRI allows detection of the onset of annuloaortic ectasia (Fig. 11-22) with dilatation of the aortic root and ascending aorta, and visualization of a dissection. Aortic regurgitation can be observed and quantified with velocity-encoded pulse sequences. Observations on the aortic root and quantification of aortic regurgitation can also be made by echocardiography.
Aortography is usually reserved for urgent clinical situations in which noninvasive imaging was inconclusive. Some surgeons request coronary angiography to evaluate whether a dissection extends near or into the coronary arteries. Occasionally, aortography can identify an entry site of a dissection that is not apparent on other methods (Fig. 11-23).
Sinus of Valsalva Aneurysms
Etiology
An outline of sinus of Valsalva aneurysms is presented in Box 11-4. Discrete aneurysms that involve a single sinus are usually congenital (Fig. 11-24), although rarely dilatation of two or all three sinuses may also be congenital. These are generally less than 4 cm in diameter and involve mainly the right sinus. The tissue in the aortic annulus adjacent to the leaflet histologically has sparse fibroelastic elements and grossly may have fenestrations through the cusp. A sinus of Valsalva aneurysm can develop as a consequence of a ventricular septal defect. One of the ways a ventricular septal defect can close spontaneously is to form fibrous tissue around its edges. As the membranous ventricular septal defect becomes smaller, the adjacent leaflet of the aortic valve is pulled inferiorly into the defect. The clinical consequence of the developing leaflet prolapse is that the left-to-right shunt through the ventricular septal defect is transformed to that of aortic regurgitation.
Because the sinuses of Valsalva lie completely within the cardiac silhouette (Fig. 11-25), the discrete type of aneurysm is not visible on the plain chest film. If the ascending aorta is also dilated, the right side of the mediastinum will have the characteristic convexity of the aorta as it extends into the adjacent lung.
Calcification
Calcification of the sinuses of Valsalva above the aortic leaflets is rare. If there is also extensive aortic calcification, this indicates syphilitic aortitis. Mild calcification of the nondilated sinuses and flecks in the ascending aorta suggest the presence of type II hyperlipoproteinemia. Rarely, congenital or nonsyphilitic aneurysms in the aortic root may calcify.
Complications
Aortic regurgitation is the main complication of progressive dilatation of the aortic annulus and the resultant lack of coaptation of the leaflets. Any type of sinus of Valsalva aneurysm can rupture into an adjacent structure. The onset is abrupt with severe aortic regurgitation or a torrential left-to-right shunt. Most sinus aneurysms rupture into the right sinus; they perforate anteriorly into the right ventricular outflow tract, dissect into the ventricular septum, or perforate posteriorly in the right atrium. Aneurysms of the noncoronary sinus rupture into the right atrium. Rupture of the left sinus into the left atrial appendage is extremely rare. When an aneurysm ruptures, aortography shows contrast medium entering the cardiac chamber and opacifying downstream structures on subsequent films (Fig. 11-26). Both a left ventriculogram and an aortogram may be necessary to distinguish a ventricular septal defect with aortic regurgitation from a ruptured sinus of Valsalva aneurysm. The contrast in the right ventricle from an aortogram could have passed into the left ventricle from aortic regurgitation and then across a ventricular septal defect, or it could have flowed directly from the aorta through the rupture into the right ventricle.
Aortitis
A number of clinical syndromes have vasculitis that involves the aorta. Most of these diseases are either associated with or caused by immune complexes deposited in the vessel wall. Intimal proliferation and fibrosis, degeneration of the elastic fibers, round cell infiltration, and occasionally giant cells usually allow a specific histologic diagnosis. The gross changes, except in Takayasu disease, are far less specific, regrettably so because these are the features seen on angiography and cross-sectional imaging. Aortitis produces aneurysms in many portions of the aorta and its related branches. They are usually fusiform but occasionally saccular. Takayasu disease is the only aortitis that produces stenoses in the thoracic aorta. Significant stenoses in the aortic arch are well known in the acquired disease: aortic dissection, false aneurysms from laceration of the aortic arch after a motor vehicle accident, infected aortic aneurysms with abscess formation, Behçet’s disease, and rarely atherosclerotic and syphilitic disease.
Takayasu Aortitis
Classifications
There are four types of Takayasu aortitis (Fig. 11-27). The Shimizu-Sano or type 1 is characterized by stenoses throughout the aortic arch and the innominate, carotid, and subclavian arteries. Type 2, or Kimoto type, shows segmental stenoses in the descending thoracic and abdominal aortas, including in the renal arteries. The Inada, or type 3, includes stenoses of both the aortic arch and the distal thoracic and abdominal aorta. Pulmonary artery stenoses with any aortic involvement define the disease as type 4. The most prevalent type of Takayasu disease is type 3 (55%), followed by type 2 (11%) and type 1 (8%). About half of these patients have pulmonary stenoses. Aortic regurgitation is usually mild, although it may occasionally be severe.
Imaging Assessment
Because of its diffuse and widespread nature, you may need both MRI and angiography to assess the extent and severity of Takayasu aortitis. Biplane thoracic aortograms or gradient echo MRI will detect aortic regurgitation and aortic arch stenosis (Figures 11-28, 11-29, 11-30). Abdominal aortography or magnetic resonance angiography will outline renal artery involvement and evaluate the arteries to the legs and gastrointestinal tract (Fig. 11-31). Occasionally, you will need angiography and ventilation-perfusion scans to assess pulmonary involvement. Conventional CT and CT angiography are useful in disease diagnosis (Fig. 11-32).
The earliest change seen on the angiogram is an irregularity or narrowing of the aortic lumen even though there is no pressure gradient. MRI frequently shows a thickened aortic wall (Fig. 11-33). More severe stenoses have collateral circulation with reconstitution of distal vessels. Associated aneurysms may be either saccular or fusiform and show an irregular dilatation of a long segment of the aorta. A distal stenosis in the aorta may secondarily produce proximal aortic dilatation, so it may be difficult to distinguish a concomitant aortitis of the proximal aorta from secondary dilatation of a normal aortic wall as a result of a more distal stenosis.
Other Types of Aortitis
From an imaging perspective, the aorta dilates in response to the weakened structural support of its wall (Fig. 11-34). As a rule, the ascending aorta dilates more than the arch, and the abdominal aorta is little involved (the opposite of atherosclerosis). As healing progresses, the aortic wall may become quite thick (Fig. 11-35). Aortic rupture can occur as a consequence of any type of aortic dilatation from aortitis. Aortic regurgitation mainly is from dilatation of the aortic annulus, but there may be inflammatory valvulitis and bacterial endocarditis. As listed in Box 11-5, giant cell arteritis, ankylosing spondylitis, rheumatoid arthritis, the aortitis associated with rheumatic fever, relapsing polychondritis, Reiter syndrome, and syphilis all may produce aortic regurgitation and dilatation of the ascending aorta and arch. The aorta may be involved in the collagen diseases of systemic lupus erythematosus, scleroderma, and rarely in ulcerative colitis or psoriasis. Although these entities pathologically and clinically are rather specific, the major angiographic finding is fusiform and symmetric dilatation of the aorta.
Box 11-5 Diseases that may produce aortitis with dilatation of the ascending aorta and aortic regurgitation
Ankylosing Spondylitis
Ankylosing spondylitis affects that portion of the aorta behind the sinuses of Valsalva (Fig. 11-36). The process extends downward to the aortic cusps, which becomes thickened, and upward a few centimeters in the ascending aorta. Ankylosing spondylitis is one kind of severe aortitis that crosses the distinct junction of the sinotubular ridge as it dilates the aortic root and ascending aorta. In contrast to syphilis, the scarring process in ankylosing spondylitis involves the sinuses of Valsalva, both the free edge and the base of the aortic leaflets, and extends below the aortic valve to the mitral annulus. In syphilis, the scarring begins above the sinuses of Valsalva and only the free edge of the aortic valve is thickened and curled.
Behçet’s Disease
Behçet’s disease is a rare vasculitis with oral, skin, and genital ulcers, eye lesions, aneurysms of the aorta and pulmonary arteries, and occlusion of the vena cava. Although any of the medium-sized and large arteries may be involved by this disease, the aorta and pulmonary arteries are the most common site (Fig. 11-37). The ostium of arteries originating from the aorta may be stenosed or occluded. The Hughes-Stovin syndrome with pulmonary aneurysms and venous thrombosis may be the same disease as Behçet’s disease without the oral and genital ulcers. Other similar entities are the aortic aneurysms and brachiocephalic stenoses in Takayasu disease.
Cardiovascular Syphilis
The aortitis of syphilis characteristically involves the ascending aorta and begins above the sinotubular ridge. The sinotubular ridge is preserved and does not dilate. The diagnosis of aortitis rests on two signs: calcification and dilatation. Calcification, which occurs in about 25% of those with luetic aortitis, is initially thin with sharp margins. Later, when severe atherosclerosis has developed, there are larger, irregular chunks of calcium. The calcification tends to occur along the anterolateral wall of the aorta, but in later stages involves the entire circumference (Fig. 11-38). Calcification of the ascending aorta is also seen in pure atherosclerosis, and rarely, in Takayasu arteritis so that neither its presence nor absence is diagnostic. However, a densely calcified ascending aorta from the sinuses of Valsalva to the arch vessels is typical of the severe, superimposed atherosclerosis of cardiovascular syphilis.
Aortic aneurysm occurs in about half of patients with cardiovascular syphilis and is mainly found in the thoracic aorta (Fig. 11-39). Counting multiple aneurysms, approximately 50% occur in the ascending aorta, 30% in the arch, 15% in the descending aorta, and less than 5% in the abdominal aorta. These aneurysms may rupture into or compress the adjacent superior vena cava, bronchi, esophagus, pulmonary artery, and pleural and pericardial cavities. The sinuses of Valsalva may be the site of syphilitic aneurysms either with primary involvement or with extension of the dilated ascending aorta. In contrast to sinus dilatation from cystic medial necrosis, luetic involvement of the sinuses may be eccentric. Although the shape of the aneurysms is unpredictable, they tend to be eccentric and saccular. In the series of Steinberg and colleagues, of 60 luetic aneurysms, 43 (72%) were saccular, and 17 (28%) had fusiform dilatation.
Infected Aortic Aneurysms
In 1885, William Osler used the term mycotic aneurysm to describe an infectious process involving an arterial wall. This designation has been replaced by the term infected aneurysm to include organisms of both bacterial and fungal origin. Infected aneurysms occur in persons of all ages, although they are usually seen in adults. Aneurysms caused by bacterial infection in children are usually associated with an underlying congenital abnormality, such as coarctation of the aorta, Marfan syndrome, or sinus of Valsalva aneurysm. Such aneurysms can also arise in an aorta damaged by trauma, as from previous aortotomy or at the tip of an indwelling catheter. In adults, a predisposing condition is almost always present because aortic involvement is extremely rare in overwhelming septicemia. Bacterial endocarditis, occurring on a substrate of either congenital or rheumatic heart disease or intravenous drug addiction, is a predisposing factor. Vascular infection may also arise by contiguous spread from adjacent empyema or infected lymph nodes; this is the usual pathophysiology of a tuberculous aneurysm.
Because the shape, size, and location of an aneurysm are not specific for infection, any aneurysm is a candidate for the site of the infection in a patient with the clinical manifestations of a systemic infection. Several causative organisms are listed in Box 11-6. The most prevalent site of infected aneurysm is the femoral arteries, although infections are commonly located in the thoracic and abdominal aorta. The most frequent location in the thorax is the lesser curvature of the aortic arch in the region of the ligamentum arteriosum. This type of aneurysm occurs at the junction of the aorta with a patent ductus arteriosus and at the site of a coarctation. In the latter case, the aneurysm is frequently located just distal to the coarctation because aneurysms occurring as part of the coarctation are proximal, and an aneurysm occurring in the poststenotic segment in the jet stream is prone to be infected. The aortic root is a common location for infection in patients with aortic valve disease. These aneurysms are located in the sinuses of Valsalva and extend outward into the adjacent mediastinum.
Abscesses in the valve ring within the myocardium are difficult to image (Fig. 11-40). These patients have active infective endocarditis on one or more valves, usually the aortic valve, and there is always valvular regurgitation. The angiographic detection depends on seeing the deformity of the valve ring or its displacement by the abscess cavity. In the aortic region, the valve ring abscess usually lies behind the aortic valve adjacent to the left atrium and mitral annulus (Fig. 11-41).
An infected aneurysm is usually saccular, although it may be any shape, including fusiform (Fig. 11-42). It may not be possible to distinguish between a saccular true aneurysm and a false aneurysm that has perforated into the mediastinum (Fig. 11-43). In the thorax, two thirds of these aneurysms are apparent on plain chest films that show mediastinal enlargement. Accessory findings include tracheal deviation and adjacent gas density. Infected aneurysms in the aortic root may be difficult to distinguish from the normal curvature of the sinuses of Valsalva. In these patients, a biplane aortography should be performed to search for the eccentric outpouching. Because of the adjacent inflammatory response, aortic root abscesses have a thick wall, which can be imaged with CT or MRI.
The natural history of infected aneurysms is expansion and subsequent rupture. Infections originating primarily in the mediastinum may rupture into adjacent structures, causing arteriovenous fistula or exsanguination into a bronchus. Rupture into the left pleural space occurs because of the left-sided descent of the descending aorta. A para-aortic mediastinal or pleural abscess may secondarily rupture into the aorta. One of the complications of a tuberculous lymph node is erosion into the aorta. Bone erosion of the anterior portion of the vertebral bodies and lateral displacement of the mediastinal lines are accessory signs of a chronic and slow-growing tuberculous aneurysm.
Thoracic Cardiovascular Trauma
Penetrating Wounds
The purpose of imaging in evaluating penetrating cardiovascular trauma is severalfold:
Aortic Tears
Although the aorta may be torn in any location, in over 90% of nonpenetrating trauma patients who survive the point of the laceration is the isthmus. It is here that the relatively mobile ascending aorta and arch join the rigid descending aorta, which is fixed by the pleura, resulting in a plane of shear. The tears at the isthmus are usually transverse but are occasionally ragged or spiral. When the aorta is completely ruptured, the distal end may retract several centimeters so that the intervening vascular channel is composed entirely of periadventitial and pleural tissue (Fig. 11-44). Lesser injuries that may be imaged include intimal hemorrhage with laceration, and occasionally, intravascular thrombus attached to the isthmus.
In the 10% of patients with traumatic rupture of the aorta from blunt trauma, the origins of the innominate, left carotid, or left subclavian arteries are injured, occasionally with other injury to the ascending aorta. The innominate artery injury may be a pseudoaneurysm at its origin or in its proximal segment. This artery may also be occluded, in which case collateral circulation comes from the circle of Willis and flows retrograde into the ipsilateral vertebral artery.
The imaging evaluation in the diagnosis of suspected aortic transection remains controversial even after hundreds of articles analyzing the role of the chest film, CT, transesophageal ultrasound, and angiography (Figures 11-45, 11-46, 11-47, 11-48). The imaging of each patient needs to be individualized according to the amount of trauma, the cardiovascular stability of the patient, and the medical resources available. Several guidelines appear to have reasonable reliability in the triage of patients with blunt chest injury. The chest film is a useful first step in mild trauma, in addition to identifying lung and musculoskeletal injuries, because if it is normal with no signs of mediastinal hemorrhage, then it is quite unlikely that an aortic tear is present. If, however, despite the negative chest film, the amount of trauma or its mechanism was potentially sufficient to tear the aorta, then CT or angiography is required for definitive diagnosis (Fig. 11-49). In like manner, normal findings on chest CT with intravenous contrast virtually exclude aortic injury. If the CT shows mediastinal widening or hemorrhage, the diagnostic possibilities are arterial injury, which requires surgery, or rupture of small mediastinal veins, which does not. In these instances, aortography, although not foolproof, is the accepted practice (Fig. 11-50).
Cardiac Injury
Blunt trauma to the heart and pericardium is seen indirectly on chest films with signs of cardiac tamponade and ventricular dysfunction (Box 11-7). An enlarging cardiac silhouette or pulmonary edema implies cardiac injury. In general, echocardiography and angiography are needed to analyze the site and physiologic extent of the trauma.
Box 11-7 Blunt cardiac trauma
PERICARDIUM
Damage to the cardiac valves occurs much less frequently with nonpenetrating chest trauma. The aortic valve may be disrupted along the base of the leaflets or by laceration of the middle or edge of a cusp (Fig. 11-51). Damage to the mitral and tricuspid valves is rare and is associated with varying degrees of leaflet stability, culminating in a flail leaflet when a papillary muscle is ruptured.
Myocardial ischemia after blunt trauma is a sign of coronary artery injury. The task of cardiac imaging is to distinguish among cardiac contusion, myocardial infarction from preexisting coronary artery disease, and direct traumatic injury to the coronary artery. Coronary arteriography may demonstrate normal coronary arteries, indicating that there is a contusion of the heart. There may be coronary tears, dissections, or extrinsic stenoses from an adjacent hematoma. When an artery is transected, the distal ends constrict in a natural attempt to reduce blood flow so that arteriographic visualization may be subtle. After injury to the coronary arteries, ventriculography may show abnormalities such as ventricular aneurysm, pseudoaneurysm, or papillary muscle dysfunction. After a penetrating injury, there may be arteriovenous fistulas, either to the adjacent coronary vein or into the coronary sinus, right atrium, or right ventricle.
Thoracic Atherosclerotic Aneurysms
Appearance
Atherosclerotic thoracic aneurysms can be either saccular or fusiform. The saccular variety involves expansion of only a portion of the wall and looks like an eccentric blister when viewed in tangent (Fig. 11-52). The most common type is the fusiform aneurysm in which the entire aortic segment is cylindrically dilated (Fig. 11-53). The thin wall of the aortic aneurysm may appear thick because of a laminated thrombus within the sac. The aortic lumen may not be dilated if the aneurysm contains an intraaneurysmal thrombus. In such cases, the correct diagnosis of thrombus in an aneurysm may hinge on locating intimal calcifications in the wall of the aorta, which is widely separated by thrombus from the apparent lumen or on finding an adjacent soft tissue mass that is concentric to the aneurysm.
Location
The diseases that are associated with the greatest extent of atherosclerotic change in the ascending aorta are type II hyperlipoproteinemia, syphilis, and diabetes mellitus. In type II hyperlipoproteinemia, the calcific deposits involve the sinuses of Valsalva and aortic cusps, but they rarely produce aortic stenosis. Diabetes mellitus and syphilis may also result in extensive plaques in the ascending aorta. Severe calcification confined to the ascending aorta as seen on a chest radiograph represents dystrophic calcification from any inflammatory process, including atherosclerosis. An aortitis such as Takayasu disease may calcify after many years.
Imaging
Imaging in the diagnosis of atherosclerotic thoracic aortic aneurysms is performed with spiral CT, MRI (Fig. 11-54), or biplane angiography. With MRI, the oblique image plane can be rotated to show the entire aorta. The images should show:
Acute Aortic Syndrome
These lesions often occur in similar locations and patients who present with these processes clinically tend to be hypertensive. Making the appropriate diagnosis is important because the risk of rupture is significantly higher in patients with a penetrating ulcer or intramural hematoma than in patients with classic aortic dissection.
Table 11-2 lists characteristics of different imaging modalities in the setting of acute aortic syndrome. Table 11-3 lists clinical and diagnostic findings of the three pathologic processes that comprise acute aortic syndrome.
TABLE 11-2 Imaging modality characteristics for acute aortic syndrome
Modality | Advantages | Disadvantages |
---|---|---|
Chest radiograph | Easily performed | Low sensitivity Low specificity |
Cardiac-gated multidetector computed tomography | High sensitivity and specificity Rapid scan and interpretation Multiplanar images |
High radiation dose Large contrast dose |
Angiography | High sensitivity and specificity for aortic dissection and aneurysmal disease | Invasive Large contrast dose Cannot diagnose intramural hematoma |
Magnetic resonance imaging | High sensitivity and specificity Multiplanar images |
Long scan times |
Transesophageal echocardiography | High specificity and sensitivity for ascending aortic injury and dissection | Operator dependent |
Penetrating Aortic Ulcer
The presence of dissection in this process is not the same type of dissection seen in classical aortic dissection. In penetrating aortic ulcers, the flap is irregular and thick and does not significantly encroach on the true lumen of the aorta. The dissection also is limited in the extent of its course. The dissection is usually focal, whereas, in classic aortic dissection, the dissection plane often extends the entire length of the aorta. This focal and limited dissection may be secondary to scarring within the media as a result of atherosclerotic disease. Most penetrating aortic ulcers occur in the descending thoracic aorta in an area of severe atherosclerosis, although rarely they are present in the ascending aorta and in the abdominal aorta. Roughly 42% of patients with penetrating aortic ulcer also show evidence of abdominal aortic aneurysmal disease. Ulcers can occur in the ascending thoracic aorta, which may weaken the aortic wall resulting in disruption of the aortic valve and subsequent aortic regurgitation.
The chest film frequently shows diffuse or focal enlargement of the descending thoracic aorta with a widened mediastinum. If the aortic ulcer is leaking, there may be pleural effusions. To obtain a precise diagnosis, further imaging is required such as angiography, CT, MRI, or TEE. Contrast-enhanced, cardiac-gated multidetector CT is nearly 100% sensitive and specific for evaluating acute aortic syndrome. TEE is about 95% sensitive and specific for diagnosing acute aortic dissection, and intramural hematoma, and associated valvular regurgitation; however, this modality is operator dependent, and therefore accuracy in test performance and interpretation can vary. Penetrating ulceration may be more difficult to identify by TEE than by CT or MRI. Angiography has become less popular as a first-line investigation because of its invasive nature and because it may give rise to false-negative results if the ulcer is not profiled on the projections obtained. On CT, penetrating ulcers appear as focal excavation or crater within an area of mural thickening (Fig. 11-55). The aortic wall is frequently thickened, and there is inward and upward displacement of the calcified intima by the hematoma. Extensive adjacent calcification and ragged edges help to distinguish this entity from aortic dissection. Intravenous contrast may flow into a crescentic intramural hematoma, which can extend into the mediastinum.
MRI may be the best modality for differentiating intramural hematoma from atherosclerotic plaque and chronic intramural thrombus. By MRI a subacute hematoma in the wall of the aorta demonstrates high signal intensity on both T1- and T2-weighted images. As the methemoglobin is further degraded and absorbed, the signal intensities will return to those of adjacent tissues (Fig. 11-56).
The natural history of penetrating aortic ulcers continues to emerge in the literature. Most penetrating ulcers are probably asymptomatic, with a small number causing a clinical problem. If the patient presents with pain, he or she is at significant risk of having or developing complications such as pseudoaneurysm or contained rupture, fistula or even free rupture. Over the next few days, the pain may resolve as the ulcer stabilizes. If the pain continues or recurs, this corresponds to progression of the pathologic process and the probable development of complications. If the pain subsides or complications have not occurred, the ulcer may remain unchanged for many years, although there is a possibility of aortic dilatation and aneurysm formation.
Intramural Hematoma
Intramural hematoma is characterized as a concentric aortic wall hematoma without radiographic evidence of an intimal flap or penetrating ulceration. The hematoma is usually located within the media of the aortic wall. Like penetrating ulcers, it is most often found in the descending thoracic aorta. The hematoma may be secondary to hemorrhage, without intimal disruption, from the aortic vasa vasorum as seen in patients with cystic medial necrosis. A true intramural hematoma occurs in absence of identifiable penetrating aortic ulcer (Fig. 11-57). It can, however, be secondary to rupture of an atherosclerotic plaque/ulcer that penetrates into the internal elastic lamina and allows subsequent hematoma formation within the media of the aortic wall (Fig. 11-58). A subacute hematoma in the wall of the aorta can be characterized with MRI by high signal intensity on both T1- and T2-weighted images.
CONGENITAL ANOMALIES
The imaging evaluation of a suspected vascular ring begins with a chest film and a barium esophagogram. The right aortic arch is easily identified on adult chest films. A posterior indentation of the barium-filled esophagus at the level of the aortic arch suggests a retroesophageal vascular structure. A small imprint implies a retroesophageal subclavian artery and a larger impression is the aorta between the esophagus and spine. Double aortic arch usually has a higher and larger impression on the right side of the esophagus compared with the smaller and inferior left-sided convexity. Most complex arch anomalies require cross-sectional imaging or angiography to map the entire anomaly before surgery. These anomalies can be difficult to analyze and frequently require multiple projections. With angiography, cranially angled oblique views will project the ductus below the aortic arch. With MRI, coronal, axial, and oblique views in the plane of the aorta are needed to trace each vascular structure through the mediastinum in relation to the trachea.
Left Aortic Arch
There are many normal variations of the origins of the aortic arch arteries. The right and left carotid arteries, the right and left subclavian arteries, and the left vertebral artery can all originate separately from the aortic arch or be joined or originate with their nearest neighbor. An aberrant right subclavian artery is seen in about 1% of persons and usually goes behind the esophagus but may pass between the esophagus and trachea or may go anterior to the trachea (Figures 11-59, 11-60). The origin of the right subclavian artery, if it is dilated, is called a diverticulum of Kommerell (Fig. 11-61).
The aortic diverticulum is on the inferior curvature of the aortic arch in the isthmus (Fig. 11-62). In the embryo, the right side of the double aortic arch rejoins the left arch to form the descending aorta. The aortic diverticulum is the obliterated end of the right arch. A ductus diverticulum is the obliterated aortic end of the ductus arteriosus. These diverticula may enlarge and be confused with an aortic aneurysm or a traumatic laceration.
Right Aortic Arch
Mirror-image branching from a right aortic arch is almost always associated with congenital heart disease. The order of origin of the branches is typically a left brachiocephalic artery, right carotid artery, and right subclavian artery (Fig. 11-63). The ductus arteriosus goes from the left subclavian artery to the left pulmonary artery in front of the trachea and does not cause a vascular ring. About 25% to 50% of patients with truncus arteriosus have a right aortic arch with mirror-image branching, and about 25% with tetralogy of Fallot have this type of right aortic arch. A rare variation of mirror-image branching with right aortic arch has an aortic diverticulum behind the esophagus, which connects with the left ductus arteriosus, completing the vascular ring.
The most common type of right aortic arch has an aberrant retroesophageal left subclavian artery (Fig. 11-64). If a left ductus connects this left subclavian artery to the left pulmonary artery, a vascular ring is formed. Although there is a potential for compression of the trachea and esophagus if a left ductus persists, most patients are asymptomatic. The incidence of congenital heart disease with this type of right arch is less than 2%.
An unusual right aortic arch anomaly has a stenosis in the left subclavian artery. The left subclavian artery may arise from the left pulmonary artery (isolation of the left subclavian artery) or may have a stenosis near the aortic diverticulum (Fig. 11-65).
A right aortic arch is identified on the chest film as a right paratracheal mass that displaces both the trachea and the esophagus leftward (Fig. 11-66). The barium-filled esophagus is displaced anteriorly on the lateral film if there is an aberrant left subclavian artery. The para-aortic stripe in the upper middle mediastinum is present on the right side and absent on the left. In the lower thorax above the diaphragm the aorta crosses to the left side and the left para-aortic stripe again becomes visible. On CT and MRI the aortic arch arteries can be traced from their origins to map their position in relation to the trachea and esophagus (Fig. 11-67).
Double Aortic Arch
The ascending aorta may split into right and left aortic arches, which pass on both sides of the trachea to join posteriorly behind the esophagus (Fig. 11-68). In most cases, the right arch is larger and higher than the left arch (Fig. 11-69). Although double aortic arch is rarely associated with congenital heart disease, there is frequently compression and malacia of the trachea. The plain chest film may show bilateral paratracheal masses with compression of the intervening trachea. A barium esophagogram shows bilateral indentations by the two aortic arches along the lateral sides of the esophagus with the right side superior and larger than the left side.
The angiographic picture of a double aortic arch is an “Aunt Mary.” The characteristic double-looped aorta is visualized with either a left ventriculogram or aortogram (Fig. 11-70). MRI is more complex but also shows the heart and mediastinal structures (Figures 11-71, 11-72).
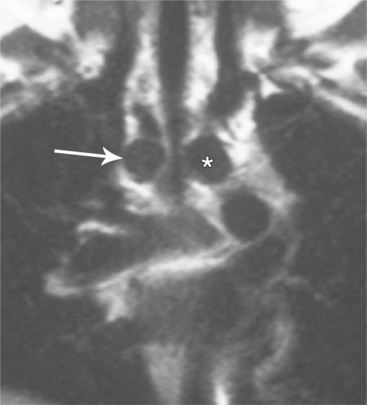
FIGURE 11-71 Coronal T1 magnetic resonance image of double aortic arch. Right arch (arrow) and left arch (*).
Pulmonary Artery Sling
Aberrant origin of the left pulmonary artery from the right pulmonary artery is part of an unusual anomaly in which the left pulmonary artery passes between the trachea and the esophagus (Figures 11-73, 11-74, 11-75, 11-76). The diagnosis can occasionally be made on a lateral chest film taken during a barium swallow so that the aberrant left pulmonary artery is a mass between the trachea and the esophagus. Most patients have cardiovascular and tracheoesophageal anomalies. The major respiratory abnormality is usually a significant stenosis in the right main stem bronchus and the tracheal bifurcation. In the compressed trachea and bronchus, the cartilage may be formed abnormally because that segment of the bronchus usually remains stenotic even after surgical relocation of the aberrant pulmonary artery. The radiologic features of air trapping from the stenotic bronchus include an opaque right upper lobe caused by poor clearing of fetal fluid and lobar emphysema with a hyperlucent right or left lung.
Coarctation of the Aorta
In 1791, Paris delivered a paper on the pathology of coarctation. Although the clinical and pathologic signs of collateral flow in coarctation were known in the 19th century, the radiologic recognition of rib notching and the abnormal mediastinal silhouette were not firmly established until nearly 30 years after the first chest films were taken. In 1928, Abbott described the rib notching from enlarged intercostal vessels and the size discrepancy between the aortic arch and the descending aorta at the level of the left subclavian artery. Some of the first angiographic examinations were performed for coarctation in 1941.
Classification
There are numerous classifications of coarctation based on the age of the patient, the position of a patent ductus arteriosus in relation to the coarctation, and the length of the coarctation. Most of these schemata, including the classification into infantile or adult types, have limited usefulness in patient management because there is great variability within the categories, and the adult type of coarctation is frequently present in infants. Preductal and postductal coarctation are meaningful if the ductus is patent. Box 11-8 is a useful list of imaging observations that includes ductal patency, extent of collaterals, aortic arch anomalies, and coarctation in unusual locations.
Characteristics
The position of a patent ductus arteriosus with respect to the coarctation affects both the clinical presentation and the imaging interpretation. A ductus arteriosus may originate proximal, distal, or adjacent to a coarctation. If the coarctation is distal to the ductus arteriosus, blood flow is initially from the aorta to the pulmonary arteries in a left-to-right direction. If later the pulmonary vascular resistance increases because of an Eisenmenger reaction, the shunt may become bidirectional or reversed. If the coarctation is proximal to the ductus arteriosus, flow through the ductus will depend on the size of the ductus and the difference between the pulmonary and systemic vascular resistances. In this situation, the blood flow is frequently from the pulmonary artery to the descending aorta, a state that produces cyanosis in the lower half of the body. Oxygenated blood from the left ventricle goes to the aortic arch arteries, whereas deoxygenated blood from the right ventricle goes through the ductus to the lower body. A juxtaductal coarctation produces a complex pattern of blood flow, which may vary dynamically as the pulmonary and systemic vascular resistances change with daily activity.
Congenital bicuspid aortic valve is frequently associated with coarctation. Between a quarter and half of the patients with aortic coarctation also have a bicuspid aortic valve. Anomalies associated with aortic coarctation are listed in Box 11-9.
Chest Film Abnormalities
Plain film findings have their angiographic counterpart and are particularly useful in searching for the extent of collateral supply. The thoracic aorta shows an abnormal contour on the chest film in roughly 60% of patients with coarctation. The “figure 3 sign” is the undulation in the distal aortic arch at the site of the coarctation (Fig. 11-77). The distal convexity in this region represents the poststenotic dilatation. There is considerable variability in the size of the ascending aorta and in the upper half of the figure 3 sign. The ascending aorta may be large, normal, or invisible on the chest film, reflecting the wide morphologic variety of aortic coarctation. Because the left subclavian artery dilates in response to the hypertension on the proximal side of the coarctation, this vessel is frequently visible as it swings from the mediastinum toward the apex of the left lung.
Rib notching is the result of enlarged and tortuous intercostal arteries that serve as collateral channels. The notches are an exaggeration of the neurovascular groove in the inferior aspect of the rib (Fig. 11-78). The degree of notching ranges from minimal undulations, which are variations of normal findings without coarctation, to deep ridges in the inferior rib margin. A small notch near the costovertebral joint is normal, so that the more lateral the notching, the more likely it is to be pathologic. Rarely, the intercostal artery is so tortuous that it notches the superior aspect of the adjacent inferior rib. Rib notching is uncommon before the age of 6 years, and its frequency increases with age, so most adults have this sign. The notching may occur at scattered sites and is usually not present on all ribs. After surgical repair of the coarctation, rib notching regresses as the bone is remodeled and the collateral vessels become smaller.
The intercostal arteries that serve as collateral channels originate from the descending aorta. For this reason, the first and second ribs do not have notches because their intercostal arteries come from the superior intercostal artery, which originates from the subclavian artery, above the coarctated site. Unilateral rib notching implies the presence of an anomalous subclavian artery. Notching of the left ribs only occurs when an aberrant right subclavian artery originates below the coarctated segment. Unilateral notching of the right ribs exists when the coarctation originates between the left carotid and left subclavian arteries. The size and extent of the notching reflects the amount of collateral blood flow through the intercostal arteries. When the coarctation is mild, no notching may be present; conversely, severe stenosis in the adult almost always has some element of rib notching.
In infancy, the abnormal mediastinal contours are invisible because of the overlying thymus. The typical chest film displays signs of congestive heart failure with a large cardiac silhouette and perihilar pulmonary edema (Fig. 11-79). The extent of both of these findings depends on the severity of the coarctation and the patency of the ductus arteriosus. A barium esophagogram may be useful to outline the medial side of the aorta. The barium in the esophagus, when fully distended, has a reversal of the figure 3 sign. The sharp lateral outpouching represents the site of the coarctation and the inferior area of constriction represents the poststenotic dilatation of the aorta.
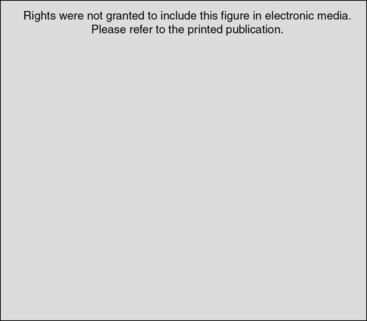
FIGURE 11-79 Coarctation in a 2-month-old infant. The large cardiac silhouette and the indistinct pulmonary vessels reflect congestive heart failure. The wide mediastinum, particularly on the right side, is the thymus. The site of the coarctation (the “figure 3 sign”) is typically not visible at this age.
(From Miller SW: Aortic arch stenoses: coarctation, aortitis, and variants, Appl Radiol 24:15-19, 1995.)
Imaging Examination
MRI is the preferred vascular study in older children and adults because it has no ionizing radiation and can image long segments of the aorta (Fig. 11-80). Because the stenosis typically is severe and the aorta tortuous at the poststenotic segment, the technique must be tailored to the pathologic findings. Slice thickness should be about 5 mm. The coronal plane images are useful to show the extent and size of collaterals. A multislice series then is designed from the axial stack to obtain a series of oblique images parallel to the long axis of the aorta centered on the coarctated segment (Fig. 11-81). Gradient echo sequences (Fig. 11-82) in the aortic plane can produce images similar to those of aortography. Visualization of a jet indicates a significant stenosis and pressure gradient across the coarctation. CT and CT angiography with multidetector technology and three-dimensional reformatting continue to rival the resolution of conventional catheter angiography (Fig. 11-83).
Aortography gives the highest resolution of the coarctated segment, the aortic arch vessels, and the flow through the collateral channels (Fig. 11-84). The catheter from the femoral artery can almost always be advanced through the coarctated segment with a guidewire and positioned in the ascending aorta. Retrograde flow of contrast material then outlines the aortic root and identifies any bicuspid aortic valve. The left anterior oblique projection with cranial angulation projects a ductus inferior to the aortic arch (Fig. 11-85). A delayed imaging sequence of up to 10 to 12 seconds is desirable to include late collateral opacification. At the conclusion of a retrograde aortogram, a measurement of the pressure gradient across the stenotic segment should be recorded. Box 11-10 lists the elements demonstrated in an aortogram.
A common collateral channel is for blood to flow from the subclavian arteries to the internal mammary arteries, then in a retrograde direction in the intercostal arteries to the descending aorta (Fig. 11-86). This pathway is responsible for the radiologic signs of large, undulating soft tissue in the retrosternal region on the lateral chest film and for the presence of rib notching. Obviously, if a subclavian artery originates anomalously in the low-pressure region below the coarctation, there will be no collateral flow in that side of the thorax. Another collateral pathway involves the thyrocervical and costocervical arteries, which originate from the subclavian artery. These vessels course through the scapular region to join intercostal arteries in the inferior thoracic region. Collateral pathways that are rarely seen on angiography include the superior and inferior epigastric arteries, which form a bridge from the intercostal arteries to the lumbar and iliac arteries, and the anterior spinal artery and other communicating arteries adjacent to the spinal cord.
Pseudocoarctation
Pseudocoarctation is a term used by Dotter and Steinberg to denote a lesion that has the same morphology as the classic coarctation but does not produce obstruction. This anomaly has a buckling of the aorta at the isthmus with little or no pressure gradient across it. All features of a true coarctation, including the figure 3 sign, may be seen in pseudocoarctation except that there is no rib notching or sign of collateral flow (Fig. 11-87). Coarctations that have a focal constriction of less than 50% have no pressure gradient across them and have no evidence of collateral flow (such as rib notching). The chest film has a mediastinal mass that is an elongated and high aortic arch. A gradient of less than 30 mm Hg is acceptable for the diagnosis of pseudocoarctation.
Pseudocoarctation may be two separate aortic anomalies. One type is a true coarctation without a pressure gradient. The embryologic abnormality that causes coarctation presumably had only a minor expression that produced the intimal infolding and distal dilatation (Fig. 11-88). The second type is an abnormal elongation of the thoracic aorta, which is kinked at the ligamentum attachment (Fig. 11-89).
Aortic Arch Interruption
Classifications
The site of the arch interruption determines classification into one of three types (Fig. 11-90). Type A has the interruption distal to the left subclavian artery. In a variation of this type, the right subclavian artery arises from the descending aorta or the pulmonary artery. In type B, the interruption is after the left common carotid artery (Fig. 11-91). The left subclavian artery arises from the descending aorta. This variety is the most common form of interrupted arch; its variations include forms in which the right subclavian artery comes from the right pulmonary artery via a right ductus and another variation in which the right subclavian artery connects to the descending aorta. Type C interruption is a discontinuity of the aortic arch distal to the innominate artery. The left carotid and left subclavian arteries connect to the descending aorta.
Imaging Examination
The characteristic angiographic features depend on the anatomic type of interruption and the collateral flow to arteries not attached to the proximal aorta. In type A interruption with all brachiocephalic vessels arising from the ascending aorta, the angiographic appearance of these vessels resembles the letter V or W. There is a deep notch filled by the left lung between the large main pulmonary artery and the left subclavian artery. In type B interruption, the V configuration is formed by the two carotid arteries (Fig. 11-92).
Berdon WE, Baker DH. Vascular anomalies and the infant lung: rings, slings, and other things. Semin Roentgenol. 1972;7:39-64.
Bissett GSIII, Strife JL, Kirks DR, et al. Vascular rings: MR imaging. Am J Roentgenol. 1987;149:251-256.
Cigarroa JE, Isselbacher EM, DeSanctis RW, et al. Medical progress. Diagnostic imaging in the evaluation of suspected aortic dissection: old standards and new directions. Am J Roentgenol. 1993;161:485-493.
DeSanctis RW, Doroghazi RM, Austen WG, et al. Aortic dissection. N Engl J Med. 1987;317:1060-1067.
Dinsmore RE, Liberthson RR, Wismer GL, et al. Magnetic resonance imaging of thoracic aortic aneurysms: comparison with other imaging methods. Am J Roentgenol. 1986;146:309-314.
Doroghazi RM, Slater EE, editors. Aortic dissection. New York: McGraw-Hill, 1983.
Dotter CT, Steinberg I. Angiocardiography in congenital heart disease. Am J Med. 1952;12:219-237.
Dotter CT, Steinberg I. The angiographic measurement of the normal great vessels. Radiology. 1949;52:353-358.
Fisher RG, Bladlock F, Ben-Menachem Y. Laceration of the thoracic aorta and brachiocephalic arteries by blunt trauma: report of 54 cases and review of the literature. Radiol Clin North Am. 1981;19:91-110.
Fisher RG, Chasen MH, Lamki N. Diagnosis of injuries of the aorta and brachiocephalic arteries caused by blunt chest trauma: CT vs aortography. Am J Roentgenol. 1994;162:1047-1052.
Godwin JD, Turley K, Herfkens RJ, et al. Computed tomography for follow-up of chronic aortic dissections. Radiology. 1986;139:655-660.
Gomes AS, Lopis JF, George B, et al. Congenital abnormalities of the aortic arch: MR imaging. Radiology. 1987;165:691-695.
Groskin S, Maresca M, Heitzman ER. Thoracic trauma. In: McCort JJ, Mindelzun RE, editors. Trauma radiology. New York: Churchill Livingstone, 1990.
Heggtveit HA. Syphilitic aortitis: a clinicopathologic autopsy study of 100 cases. Circulation. 1964;29:346-355.
Hilgenberg AD. Trauma to the heart and great vessels. In: Burke JF, Boyd RJ, McCabe CJ, editors. Trauma management: early management of visceral, nervous system, and musculoskeletal injuries. St Louis: Mosby-Year Book; 1988:153-175.
Ishikawa K. Diagnostic approach and proposed criteria for the clinical diagnosis of Takayasu’s arteriopathy. J Am Coll Cardiol. 1988;12:964-972.
Jaffee RB. Complete interruption of the aortic arch: 1. Characteristic radiographic findings in 21 patients. Circulation. 1975;52:714.
Jaffee RB. Complete interruption of the aortic arch: 2. Characteristic angiographic features with emphasis on collateral circulation of the descending aorta. Circulation. 1976;53:161-168.
Kampmeier RH. Saccular aneurysm of the thoracic aorta. A clinical study of 633 cases. Ann Intern Med. 1938;112:624.
Kazerooni EA, Bree RL, Williams DM. Penetrating atherosclerotic ulcers of the descending thoracic aorta: evaluation with CT and distinction from aortic dissection. Radiology. 1992;183:759-765.
Kersteing-Sommerhoff BA, Higgins CB, White RD, et al. Aortic dissection: sensitivity and specificity of MR imaging. Radiology. 1988;166:651-655.
Kirks DR, Currarino G, Chen JT T. Mediastinal collateral arteries: important vessels in coarctation of the aorta. Am J Roentgenol. 1986;146:757-762.
Lande A, Berkmen YM, McAllister HAJr. Aortitis: clinical, pathologic, and radiographic aspects. New York: Raven Press, 1986.
Lande A. Takayasu’s arteritis and coarctation of the descending thoracic and abdominal aorta: a critical review. Am J Roentgenol. 1976;127:227-233.
Liberthson RR, Pennington DG, Jacobs M, et al. Coarctation of the aorta: review of 234 patients and clarification of management problems. Am J Cardiol. 1979;43:835-840.
Lindsay JJr, Hurst JW. The aorta. New York: Grune & Stratton, 1979.
Lindsey JJr. Diseases of the aorta. Philadelphia: Lea & Febiger, 1994.
Liu YQ. Radiology of aortoarteritis. Radiol Clin North Am. 1985;23:671-688.
Lupi-Herrera E, Sanchez-Torres G, Marcushamer J, et al. Takayasu’s arteritis. Clinical study of 107 cases. Am Heart J. 1977;93:94-103.
Macura KJ, Corl FM, Fishman EK, et al. Pathogenesis in acute aortic syndromes: aortic aneurysm leak and rupture and traumatic aortic transection. Am J Roentgenol. 2003:303-307.
Manghat NE, Morgan-Hughes GJ, Roobottom CA. Multi-detector row computed tomography: imaging in acute aortic syndrome. Clin Radiol. 2005;60:1256-1267.
Miller SW, Holmvang G. Differentiation of slow flow from thrombus in thoracic magnetic resonance imaging, emphasizing phase images. J Thorac Imaging. 1993;8:98-107.
Movsowitz HD, Lampert C, Jacobs LE, et al. Penetrating atherosclerotic aortic ulcers. Am Heart J. 1984;128:1210-1217.
Neye-Bock S, Fellows KE. Aortic arch interruption in infancy: radio- and angiographic features. Am J Roentgenol. 1980;135:1005-1010.
Park JH, Chung JW, Im JG, et al. Takayasu arteritis: evaluation of mural changes in the aorta and pulmonary artery with CT angiography. Radiology. 1995;196:89-93.
Park JH, Han MC, Bettmann MA. Arterial manifestations of Behçet disease. Am J Roentgenol. 1984;143:821-825.
Parmley LR, Thomas WM, Manion WC, et al. Nonpenetrating traumatic injury of the aorta. Circulation. 1985;15:405-410.
Petasnick JP. Radiologic evaluation of aortic dissection. Radiology. 1991;180:297-305.
Raptopoulos V. Chest CT for aortic injury: may be not for everyone. Am J Roentgenol. 1994;162:1053-1055.
Roberts WC. Aortic dissection: anatomy, consequences, and causes. Am Heart J. 1981;101:195-214.
Shuford WH, Sybers RG. The aortic arch and its malformations: with emphasis on the angiographic features. Springfield, IL: Charles C Thomas, 1974.
Simoneaux SE, Bank ER, Webber JB, et al. MR imaging of the pediatric airway. Radiographics. 1995;15:287-298.
Slater EE, DeSanctis RW. The clinical recognition of dissecting aortic aneurysm. Am J Med. 1976;60:625-633.
Smith AD, Schoenhagen P. CT imaging for acute aortic syndrome. Cleve Clin J Med. 2008;75:7-24.
Smyth PT, Edwards JE. Pseudocoarctation, kinking or buckling of the aorta. Circulation. 1972;46:1027-1032.
Soulen RL, Fishman EK, Pyeritz RE, et al. Marfan syndrome: evaluation with MR imaging versus CT. Radiology. 1987;165:697-701.
Steinberg I, Dotter CT, Peabody G, et al. The angiographic diagnosis of syphilic aortitis. Am J Roentgenol. 1949;62:655.
Steward JR, Kincaid OW, Edwards JE. An atlas of vascular rings and related malformations of the aortic arch. Springfield, IL: Charles C Thomas, 1964.
Taylor DB, Blaser SI, Burrows PE, et al. Arteriopathy and coarctation of the abdominal aorta in children with mucopolysaccharidosis: imaging findings. Am J Roentgenol. 1991;157:819-823.
Tunaci A, Berkman YM, Gökmen E. Thoracic involvement in Behçet’s disease: pathologic, clinical, and imaging features. Am J Roentgenol. 1995;164:51-56.
Vasile N, Matheier D, Keita K, et al. Computed tomography of thoracic aortic dissection: accuracy and pitfalls. J Comput Assist Tomogr. 1986;10:211-215.
Walker TG, Geller SC. Aberrant right subclavian artery with a large diverticulum of Kommerell: a potential for misdiagnosis. Am J Roentgenol. 1987;149:477-478.
White RD, Ullyot DJ, Higgins CB. MR imaging of the aorta after surgery for aortic dissection. Am J Roentgenol. 1988;150:87-92.
Yamada I, Numano F, Suzuki S. Takayasu arteritis: evaluation with MR imaging. Radiology. 1993;188:89-94.
Yamada T, Tada S, Harada J. Aortic dissection without intimal rupture: diagnosis with MR imaging and CT. Radiology. 1988;168:347-352.
Yamato M, Lecky JW, Hiramatsu K, et al. Takayasu arteritis: radiographic and angiographic findings in 59 patients. Radiology. 1986;161:329-334.
Yao JS T, Pearce WH. Aneurysms: new findings and treatments. Norwalk, CT: Appleton & Lange, 1994.
Yucel EK, Steinberg FL, Egglin TK, et al. Penetrating aortic ulcers: diagnosis with MR imaging. Radiology. 1990;177:779-781.